Fig. 10.1
Congenital cystic adenomatoid malformation. Chest radiograph in a neonate with a left-sided amorphous cystic mass filling most of the left hemithorax. The cardiac silhouette is shifted to the right. The right hemithorax is slightly opacified
Classification of CCAM
Stocker originally classified CCAM based on cyst size and postnatal histology, yielding three types. Subsequently type 0 (tracheobronchial defect with small firm lungs and a bronchial airway) and type 4 (an entirely alveolar defect) were added to complete the current classification. Since types 1–3 are usually adenomatoid and types 1, 2, and 4 are cystic, Stocker proposed a broader term for these defects: congenital pulmonary airway malformations (CPAM) [58, 63]. However, CCAM remains the primary acronym for these defects. A simpler classification for this defect was proposed: macrocystic or microcystic (solid), based on anatomy and appearance on antenatal ultrasound, but Stocker’s classification has persisted [58, 59, 63–65] (Table 10.1).
Table 10.1
The Stocker classification of congenital cystic adenomatoid malformation (CCAM)
Stocker type 0 (rare). This is a fatal defect in which the lungs do not develop beyond the pseudoglandular level, resulting in small hypoplastic lungs bilaterally with a bronchial airway. Also known as acinar dysplasia |
Stocker type I (macrocystic adenomatoid malformation) (60–70 %). Single or multiple large “cysts” (>2 cm diameter) are present, which communicate with the proximal airways and distal lung parenchyma. The lesion is relatively localized and most infants have a good prognosis after cyst resection. Good prognosis |
Stocker type II (microcystic adenomatoid malformation) (10–15 %). Multiple small spongelike cysts (<1 cm) replace the distal lung parenchyma. This has a worse prognosis and is more commonly associated with other anomalies, such as renal agenesis and cardiac and chromosomal abnormalities |
Stocker type III (solid cystic adenomatoid malformation) (5 %). The abnormality represents a severe end of the spectrum with multiple airless cysts involving an entire lobe or even lung. This has a poor prognosis, more commonly reported in stillbirths with CCAM |
Stocker type IV (rare). This is an entirely alveolar pulmonary defect in which large cysts replace the numerous alveoli in the periphery of the lung. Good prognosis |
Diagnosis of CCAM
The diagnosis is usually made by antenatal ultrasound at approximately 20 weeks gestation [66–70]. The lesions are monitored throughout gestation following one of three courses: increase in size, remain unchanged, or undergo spontaneous resolution. In aggressive disease, the lesion may respond to maternal steroids. A few specialist centers offer fetal interventions for high-risk cases causing hydrops including thoracoamniotic shunt for isolated cysts, open fetal surgery, or an EXIT procedure at delivery (ex-utero intrapartum treatment), depending on gestational age, the size of the cyst, and the health of the mother [59, 68, 70].
Infants present with symptoms of respiratory distress in the neonatal period, with tachypnea, increased work of breathing, and desaturation in 30 % of cases, or occasionally with hyperinflation on the side of the CCAM. Some infants may appear to be relatively asymptomatic. Ten percent of the cases present with recurrent pneumonia or pneumothorax in later childhood. A significant number of infants remain asymptomatic and are only detected incidentally.
CCAM is evident on chest X-ray, especially if it is large. Microcystic lesions may be fluid filled, but macrocystic lesions are usually aerated and may be difficult to visualize. A CT scan with contrast is usually performed to delineate the limitations of the lesion. Ultrasound may be used to identify the blood supply and exclude an anomalous systemic artery.
Management of CCAM
Initial management involves respiratory support as needed and imaging to confirm and define the extent of the lesion. Neonates with hydrops have a very high perioperative mortality as a result of the pulmonary hypoplasia and pulmonary hypertension. These neonates should be medically stabilized before embarking on surgical interventions [61, 70]. Surgery may be beneficial for neonates with significant respiratory signs and symptoms and/or compression of adjacent cardiac or major vascular structures. Controversy exists regarding the appropriateness and timing of surgery in asymptomatic or smaller lesions [60, 71–74]. Resection in infancy or early childhood is increasingly advocated to preclude infectious complications and malignant transformation (rhabdomyosarcoma, squamous cell carcinoma, bronchoalveolar carcinoma, and pleuropulmonary blastoma in 2–4 % of cases) and to encourage compensatory lung growth. If surgery is not undertaken, ideally these neonates should be followed into adulthood to ensure a timely intervention should the lesion turn malignant [74].
Surgical Considerations
The aim of surgery is to preserve viable lung tissue and reduce the mediastinal shift. The standard approach is posterolateral thoracotomy and lobectomy. Segmental resection may be used for small lesions or multilobular disease but is associated with a greater incidence of postoperative air leak. Thoracoscopic resection can be performed but access may be difficult with large cystic lesions.
Anesthetic Considerations
Thoracotomy and lung resection are generally well tolerated in neonates; blood transfusions are not usually required. Full invasive monitoring should be used. CCAM are connected to the bronchial tree, and preoperative hyperinflation resulting in ball-valve effect is a possibility, although one-lung ventilation is not usually required. Nitrous oxide is best avoided.
Infants with severe respiratory distress may require HFOV preoperatively, but it is usually possible to wean to conventional ventilation in the early postoperative period [70]. Analgesia may be provided by intravenous opioids such as fentanyl or remifentanil or thoracic epidural analgesia with the catheter inserted via the caudal route [75, 76]. Most neonates require only a brief period of postoperative ventilation, although ventilation may be extended if pulmonary hypoplasia and/or pulmonary hypertension is present.
Lung Sequestration
This lesion consists of nonfunctioning lung tissue that does not have a tracheobronchial communication. There is anomalous systemic arterial supply, usually directly from the aorta, with more than one vessel in 15 % of lesions. Sequestrations may have a patent or non-patent connection to the gastrointestinal tract (bronchopulmonary foregut malformations) [70].
Classification of Lung Sequestration
Intralobar sequestration (ILS) (75 %): the abnormal tissue is contained within normal lung predominantly within the lower lobes (often left sided). The child is generally asymptomatic but may present with hemoptysis, pneumothorax, or recurrent infections in later childhood. The venous drainage is often via the pulmonary veins. Right-sided ILS may be associated with anomalous pulmonary venous drainage characteristic of scimitar syndrome; care must be taken not to ligate pulmonary veins during surgical resection.
Extralobar sequestration (ELS) (25 %): the sequestration is completely separated from the lung by visceral pleura. There is an infradiaphragmatic arterial supply in 20 % and the lesion itself is infradiaphragmatic in 3 % of cases. Venous drainage is to the azygous system in the majority. ELS affects males four times more commonly than females and is more often associated with other anomalies such as congenital diaphragmatic hernia (CDH) (16 %), CCAM (15 %), congenital heart disease, chest wall abnormalities, and hindgut duplications.
Diagnosis of Lung Sequestration
These lesions may be detected on antenatal ultrasound or postnatal chest X-ray. The systemic blood supply must be delineated using ultrasound, CT, or MRI.
ELS is generally asymptomatic but is often detected earlier than intralobar sequestrations. Both ILS and ELS may be detected antenatally and may resolve. Symptomatic infants with large sequestrations may present with respiratory distress, feeding difficulties, or cardiac failure if the sequestered lobe is associated with significant arteriovenous or left-to-right shunting [70]. Hydrops may result from severe cardiac failure in utero, and pulmonary hypoplasia and pulmonary hypertension may be associated with large lesions, as in CCAM.
Management of Lung Sequestration
Respiratory support should be instituted as required, and the systemic blood supply defined on imaging as the first step. Surgical excision is generally advocated. The timing of surgery depends on the clinical situation. Complications such as infection and cardiovascular compromise due to shunting should be treated before resection.
Surgical Considerations
As for CCAM, thoracotomy is the standard approach for pulmonary sequestrations. Thoracoscopic resection is a viable alternative due to the relatively smaller size of the lesions. A laparotomy or laparoscopic approach is required for infradiaphragmatic lesions. Additional therapeutic interventions have been reported anecdotally including radiofrequency ablation and coil embolization [77].
The anomalous systemic vessels can be fragile elastic vessels with significant blood flow. Careful dissection and meticulous control are required to prevent hemorrhage. Control of the systemic blood supply during surgery is critical especially when the origin of the vessel is on the opposite aspect of the diaphragm to the operative field (infradiaphragmatic vessel for intrathoracic lesions). A loss of control can result in retraction of the vessel out of the operative field and catastrophic hemorrhage. A crossmatch should be available for all of these neonates.
Anesthetic Considerations
These are similar to CCAM. Neonates with severe pulmonary hypertension should be optimized medically before surgery.
Congenital Lobar Emphysema
Congenital lobar emphysema (CLE) is an obstructive overinflation disorder, which may be due to defective bronchiolar development. The affected lobe is hyperinflated and compresses the adjacent normal lung. Hyperinflation becomes progressive after birth, although neonates usually present with respiratory distress in the first few days of life. Males are more commonly affected than females (3:1). The left upper lobe is involved in almost 50 % of cases followed by the right middle lobe (28 %) and the right upper lobe (20 %); bilateral involvement is rare (Fig. 10.2). Cardiac anomalies are present in 20 % of neonates with CLE [70].


Fig. 10.2
Congenital lobar emphysema. Chest radiograph with hyperinflation of the left chest and displacement of the cardiac silhouette toward the right chest. A large gastric air bubble is present
Diagnosis of CLE
CLE may be detected antenatally but is difficult to distinguish from CCAM. Postnatally there is often early respiratory distress and clinical signs suggestive of pneumothorax. A CXR may show lobar hyperinflation, mediastinal shift, and flattening of the ipsilateral diaphragm. Ultrasound can help distinguish from a tension pneumothorax, or if stable enough, CT or MRI scan will confirm the diagnosis. If the neonate is stable, then a preoperative echocardiogram should be also performed.
Management of CLE
The primary treatment is lobectomy, which may be required on an emergent basis before a full preoperative workup can be performed. More recently, segmental lung resection has proven successful in preserving lung without an increased incidence of a recurrence [78]. Positive pressure ventilation worsens the hyperinflation, and if respiratory support is required preoperatively, then HFOV may be preferred. A chest drain should never be inserted as it may cause preferential ventilation of the abnormal lung leading to respiratory failure.
Surgical and Anesthetic Considerations
Induction of anesthesia with positive pressure ventilation may cause rapid deterioration due to air trapping. If possible, spontaneous ventilation should be maintained until one-lung ventilation has been achieved or until the chest is opened. Anesthesia should be introduced by inhalation, and lines placed while spontaneous ventilation is maintained. Muscle relaxants may be administered, but in these neonates, the inspiratory pressure should be minimized [79]. Alternatively, muscle relaxants may be avoided and intubation achieved using a combination of inhalational anesthesia, intravenous propofol with topical lidocaine (3 mg/kg) administered to the cords [54, 80]. The thoracotomy instruments and the surgeon must be ready in the event that urgent thoracotomy and decompression become necessary.
Esophageal Atresia/Tracheoesophageal Fistula
Esophageal atresia (EA) and tracheoesophageal fistula (TEF) occur in 1:3,000–1:4,500 live births [81–83]. The etiology is believed to be due to a defect in the separation of the trachea and esophagus from a common foregut, which normally occurs after 4 weeks gestation. The etiology and exact embryology remain unclear, although the theories put forth include failure of fusion of lateral tracheoesophageal folds or the tracheoesophageal septum, imbalance in epithelial proliferation and apoptosis, trifurcation of the lung bud, and a possible role of the notochord. Animal evidence suggests that a greater developmental role of the foregut in this defect as the fistula and distal esophagus may be respiratory in origin [58]. There is also evidence that specific targets in the molecular mechanisms responsible for complete separation of the trachea and the esophagus may be responsible for EA/TEF defects [84].
Classification
First classified in 1929, and modified in 1953, five common types of EA/TEF have been described, although it is probably more appropriate to describe the conditions anatomically [81, 82, 85] (see Fig. 10.3) (Table 10.2).


Fig. 10.3
Classification of esophageal atresia and tracheoesophageal fistula according to Vogt. Type 1: Obliteration of the esophagus. Type II: Atresia without fistula. Type IIIa: Atresia with proximal fistula. Type IIIb: Atresia with distal fistula. Type IIIc: Atresia with proximal and distal fistula. Type IV: Tracheoesophageal fistula (H-type fistula). Adapted from: HolzKi J. Pediatric Anaesthesia 1992; 2: 297–303
Table 10.2
EA/TEF classification and incidence in neonates
EA with distal TEF (80–85 %) |
Pure EA (5–7 %) |
Isolated TEF (“H”-type fistula) (3–6 %) |
EA with proximal and distal TEF (3–5 %) |
EA and proximal TEF (2 %) |
The length of the gap between proximal and distal esophagus is variable, as is the position of fistula (or fistulae) within the trachea. These anatomical variations have important implications for surgical strategy and anesthesia management. In one series, the fistula was mid-tracheal in 61 %, at or just above the carina in 33 %, cervical in 8 %, and bronchial in 1 %. There was more than one fistula in 3 % of patients [85, 86]. In the “H”-type TEF, the fistula is typically in the cervical region, whereas in EA with a proximal fistula, the fistula is usually 1–2 cm from the blind-ending upper pouch [83].
Neonates with EA/TEF are often born premature, with low birth weight (Fig. 10.4) [82]. Approximately 50 % of neonates with EA/TEF have associated anomalies, an incidence that increases with decreasing birth weight (<2,500 g) and with pure EA. In contrast, anomalies are less common in those with an isolated H-type fistula [82, 83, 87, 88]. The most common anomalies associated with EA/TEF are cardiac anomalies (29 %), followed by duodenal atresia and anorectal anomalies (14 %), genitourinary anomalies (14 %), intestinal malrotation (13 %), chromosomal abnormalities (trisomies 21, 18, and 13q deletion) (4 %), vertebral and skeletal anomalies (10 %), and specific-named associations (see below). The most common cardiac defects are atrial or ventricular septal defects or tetralogy of Fallot [88, 89] (Fig. 10.5) [90]. A right-sided aortic arch is present in 2.5–5 % of infants with EA/TEF [91].



Fig. 10.4
Esophageal atresia in a neonate. This chest radiograph depicts a multi-orifice orogastric tube (with side holes) ending at the mid-thoracic level. A tracheal tube ends at the thoracic inlet. Umbilical vein and artery catheters enter radiograph from below; one terminates at T6–T7 and a second at T8–T9. A gastric air bubble is not visible here

Fig. 10.5
EA/TEF with situs inversus. Chest radiograph of a neonate with EA/TEF. The multi-orificed orogastric tube (with side orifices) ends at T3–T4 reflecting EA. The cardiac silhouette and gastric bubble are reversed, present on the right side. Echocardiogram verified the presence of a ventricular septal defect. The large gastric air bubble is consistent with a distal tracheoesophageal fistula
Several disorders have been associated with EA/TEF [82]. The VATER/VACTERL association, an association of unknown etiology, is defined by the presence of at least three of the following congenital malformations: vertebral defects, anorectal anomaly, cardiac defects, TEF, renal anomalies, and limb (radial) abnormalities [90, 92, 93]. Approximately 47 % of neonates with EA have VACTERL anomalies.
VACTERL-H association is the VACTERL association with hydrocephalus, although hydrocephalus is often listed as a non-VACTERL anomaly (see above). CHARGE syndrome is an autosomal dominant disorder caused by a mutation on chromosome 7. It is associated with TEF, coloboma, cardiac defects, choanal atresia, neurocognitive and growth impairment, and genital, ear, and cranial nerve defects. Potter’s syndrome, which is associated with renal agenesis, pulmonary hypoplasia, dysmorphic facies, and Schisis association, which is associated with omphalocele, cleft lip and/or palate, and genital hypoplasia, may also be associated with EA/TEF. EA/TEF is also associated with CATCH syndrome (22q deletion that includes cardiac defects, abnormal facies, thymic aplasia, cleft palate, and hypocalcaemia). Of the trisomy syndromes [13, 20], EA/TEF is most often associated with Edwards syndrome (trisomy 18). Feingold syndrome (dominant inheritance) is similar to VACTERL syndrome but features microcephaly and learning difficulties [82]. Other syndromes associated with EA/TEF include DiGeorge sequence, Pierre Robin sequence, Fanconi syndrome, and polysplenia [58].
Diagnosis
EA may be suspected antenatally in the presence of polyhydramnios with a small or absent gastric bubble or associated abnormalities present in the fetus. Most cases, however, present after birth. A history of polyhydramnios should prompt the pediatrician to pass a size 8–10 Fr orogastric tube immediately after birth. The tube cannot pass the upper esophagus (EA). The diagnosis is strongly suspected in the first few hours after birth if an orogastric tube cannot be passed and mucous that accumulates in the upper airway cannot be cleared by swallowing. The neonate chokes or becomes cyanosed if it attempts to feed. Aspiration pneumonia due to delayed diagnosis was common decades ago but is quite uncommon in modern medicine.
The diagnosis of EA/TEF should be confirmed on plain X-ray of the chest and abdomen. A coiled nasogastric tube will be identified in the upper third of the esophagus (level T2–T4); air in the gastrointestinal tract indicates the presence of a distal TE fistula. A gasless gastrointestinal tract suggests the absence of a distal fistula, although a proximal fistula remains a possibility. Other abnormalities such as vertebral anomalies (usually in the lower thoracic region) or the “double bubble” of duodenal atresia can also be detected on preoperative films. A thorough clinical examination is important to exclude associated anomalies and the presence of coexisting problems, such as respiratory distress syndrome in premature infants. An echocardiogram is strongly recommended before surgery to identify cardiac defects and the position of the aortic arch. If the neonate has passed urine (thus excluding bilateral renal agenesis), then a renal US can be delayed until after surgery.
An H-type TEF is infrequently detected in the neonatal period but should be suspected with a history of recurrent chest infection due to repeated aspiration.
Risk Stratification
It is helpful to stratify neonates with EA/TEF according to risk. The original stratification by Waterson was based on birth weight, associated anomalies, and the presence of pneumonia [83]. Neonatal care has improved leading to a current risk stratification that is based solely on birth weight and the presence of cardiac anomalies [88, 89, 95]. Improved outcomes have resulted in survival rates exceeding 98 % in neonates with birth weights >1,500 g and without major cardiac anomalies, 82 % with birth weights <1,500 g or major cardiac anomalies, and 50 % with birth weights <1,500 g and major cardiac anomalies [95]. A four-part classification has been suggested more recently, with prediction of 100 % survival in neonates with birth weights >2,000 g without cardiac anomalies and 40 % survival in high-risk neonates with birth weights <2,000 g with major cardiac anomalies [89]. The premature infant with a major cardiac anomaly remains a high risk. Parents of infants with bilateral renal agenesis or major complications of prematurity such as grade IV intraventricular hemorrhage should be given the option of nonoperative treatment.
Medical Management
Initial management of neonates with EA/TEF is to prevent aspiration of oral secretions before definitive surgery. A 10Fr double-lumen oro-esophageal Replogle tube should be inserted into the upper pouch and placed on continuous suction, or secretions should be cleared frequently by naso-esophageal tube suction. The neonate should be given maintenance intravenous fluids and nursed 30° head up or in the decubitus position. Blood should be crossmatched and available for surgery. If preoperative ventilation is required, inspiratory pressures should be kept to a minimum, if possible placing the tip of the tracheal tube distal to the fistula (see below). Premature infants should receive surfactant according to standard protocols.
Surgical Considerations
The aim of surgery is to restore continuity of the esophagus and ligate the TE fistula, if present. Surgery is usually performed on the first or second day of life if the neonate is stable and does not require respiratory support. Primary esophageal anastomosis is usually possible in EA with distal TEF, although it may be preferable to divide the fistula and place a feeding gastrostomy in a neonate with severe comorbidities such as a duct-dependent cardiac anomaly. Primary esophageal repair can be performed 6–12 weeks after cardiac surgery.
Emergency surgery may be required if the child requires preoperative ventilation, as in the case of the preterm neonate with respiratory distress syndrome. If the lung compliance is poor, gas from the ventilator may preferentially enter the gastrointestinal tract via the fistula. This may lead to gastric distension, deteriorating cardiorespiratory status, and possible gastric rupture. Inadvertent intubation of the fistula must be excluded in cases of severe gastric distention with cardiorespiratory instability [96]. To prevent gastric distention from ventilation via the fistula, some have recommended clamping the distal esophagus as soon as the chest is opened [97]. Decompressive gastrostomy should not be undertaken as a primary intervention as it will lead to a torrential gas leak via the gastrostomy and worsening minute ventilation. The child should undergo emergency transpleural ligation of the fistula, with delayed division of the fistula and possible repair of EA at 8–10 days [83].
Neonates with pure EA or EA with a proximal TEF often have a long gap between proximal and distal ends of the esophagus. A feeding gastrostomy is inserted and the length of the gap estimated radiographically at the time of surgery. If the gap is greater than the vertical height of three vertebral bodies, upper pouch suction is continued postoperatively and delayed primary closure is usually possible by about 12 weeks of age; if the gap is greater than six vertebral bodies, a cervical esophagostomy is often fashioned and the esophagus repaired at a later date. Esophageal replacement surgery is occasionally required [83].
An esophagoscopy or bronchoscopy is often performed at the start of surgery to provide absolute confirmation of the diagnosis, to assess the position of the fistula if present, and to exclude multiple fistulae [86]. A variety of techniques have been described to identify fistulae in neonates: rigid bronchoscopy, esophagoscopy, or flexible fiberoptic bronchoscopy via the tracheal tube. The advantage of using a small flexible bronchoscope is that the scope may also be used to assess the position of the tip of tracheal tube, to pass through the fistula to assist the surgeon in identifying the fistula during surgery, and to assess the airway at the end of surgery to exclude a residual blind-ending tracheal pouch and the severity of the tracheomalacia near the fistula [98].
The traditional approach to repair of EA/TEF is extrapleural via a right posterolateral thoracotomy with the neonate placed in the lateral decubitus position with a roll under the chest to facilitate surgical access. The posterior mediastinum is approached via the 4th and 5th intercostal spaces and the extrapleural route, gently compressing the right lung. The approach is delicate and time consuming but reduces morbidity from an anastomotic leak, should one occur. If a right arch is confirmed on preoperative echo, a left-sided approach should be considered and a double aortic arch may be approached via the standard right thoracotomy [91]. If the child becomes unstable, a transpleural approach may be used.
Thoracoscopic repair has become popular in specialist centers in recent years and, in expert hands, has the same complication rate as open techniques, with comparable blood gases, operating times, and reduced time in intensive care postoperatively [99–101]. Currently recommendations are based on large case series, since no randomized controlled trials have compared the two techniques [34, 35, 102]. For thoracoscopic repair, the child is placed semiprone with the right side of the chest elevated at 45° so that the structures may be easily visualized. Lung deflation is produced by CO2 insufflation, and care should be taken to minimize hypercarbia, as described previously.
Major concerns during open or thoracoscopic surgery include accurate identification of anatomical structures and cardiorespiratory instability due to OLV and distortion of the trachea. The anesthesiologist may be asked to push on the Replogle tube to help identify the proximal esophageal pouch. Test occlusion of the fistula is good practice to ensure that the right lung can still be inflated and that a vital structure (e.g., the pulmonary artery or main bronchus) has not been clamped in error. If the neonate does not tolerate OLV, surgery may have to proceed with intermittent two-lung ventilation once the neonate recovers. This requires good communication between the anesthesiologist and surgeon. Increased FiO2 and hand ventilation may be required, but respiratory compromise usually improves after ligation of the fistula. The integrity of tracheal repair can be checked by instilling warm saline in the chest during a sustained inflation to identify an air leak by the presence of air bubbles. The lower esophagus should not be aggressively mobilized in order to avoid devascularization, as this may cause later problems with esophageal motility. A gas leak from the upper pouch during esophageal anastomosis should raise suspicion of an upper pouch fistula. Dissection of the upper pouch helps to identify a proximal fistula, if one is present, and allows mobilization of the esophagus to minimize tension of the repair. If there is significant tension at the anastomosis, the neonate should remain paralyzed and the lungs ventilated mechanically for approximately 5 days postoperatively [83]. A transanastomotic tube (TAT tube) placed under direct vision before the anastomosis is completed facilitates early feeding and should be clearly marked to preclude accidental removal postoperatively.
Early complications at EA/TEF repair include tracheobronchomalacia (20–40 %), anastomotic leak (15–20 %), anastomotic stricture (30–50 %), and recurrent fistula (10 %) [103]. Tracheomalacia is due to abnormal cartilage in the region of the fistula and often produces a typical barking cough. In severe cases, the child may develop recurrent chest infections or “near death” episodes due to acute airway collapse, and emergency aortopexy may be required in the first few months after repair [83]. An early anastomotic leak may cause a tension pneumothorax; a chest drain should be inserted and the leak explored and repaired. Late complications include gastroesophageal reflux (severe reflux in 40 %) and recurrent chest infections, probably related to gastroesophageal reflux [82]. Long-term respiratory complications including bronchiectasis may result from aspiration, GERD, and chest wall abnormalities [104]. Both the parents and their neonate are at risk for psychological and traumatic stress (including post-traumatic stress disorder) [105]. These are long-term issues centered around feeding difficulties, multiple painful procedures and surgeries, feeding, and airway issues.
Anesthetic Considerations
Anesthetic considerations include general factors relating to the thoracotomy or thoracoscopic surgery in neonates and the high incidence of comorbidity. Specific considerations include airway management and positioning the tracheal tube in the presence of a tracheal fistula. Selective OLV is not usually required as the surgeon can easily compress the lung to access the fistula. Every neonate should have a preoperative echocardiogram to identify the presence of a congenital heart defect that may range from a patent ductus arteriosus or ASD/VSD to a hypoplastic left heart. Failure to be aware of the presence of a congenital heart defect during one-lung anesthesia for an EA/TEF repair could lead to catastrophic complications including hypoxia, hypotension, and cardiac arrest.
The main anesthesia complications relate to inadvertent intubation of the fistula or preferential ventilation of the fistula causing gastric distension and desaturation [95, 106, 107]. As described above, the fistula may vary in position; two-thirds occur in the mid-trachea and one-third occur at or near the carina. The majority of complications have been described with large fistulae, particularly when they occur near the carina.
The traditional technique to secure the airway in these neonates has been to intubate the trachea while the neonate remains awake and maintain spontaneous respiration until the fistula was controlled. However, this is no longer recommended. A variety of anesthetic techniques and techniques of airway management have been described [82, 98, 106–109]. Most advocate inhalational or intravenous induction, according to personal preference, with muscle relaxant and gentle mask ventilation before intubating the trachea [98, 106–108].
Several approaches have been popularized to position the tracheal tube while avoiding intubating the tracheoesophageal atresia. One popular technique is to deliberately intubate the right main bronchus after general anesthesia is induced and then withdraw the tube until bilateral air entry is confirmed. This ensures the tracheal tube is below the fistula but does not prevent intubation of a large fistula at the carina [96]. Alternatively, rigid bronchoscopy (or flexible bronchoscopy after intubation) may be used to demonstrate the precise level of the fistula (or exclude multiple fistulae) and then plan the intubation strategy. If the fistula is mid-tracheal, the tip of the tracheal tube is ideally positioned just below the fistula, with the bevel facing anteriorly (to avoid ventilating the fistula since the origin of the fistula is the posterior tracheal wall) and gentle positive pressure ventilation used. If the fistula is at the carina, the tracheal tube may still be placed at the mid-tracheal level, provided the fistula is small. The tracheal tube should be fixed carefully and the position of the tube checked again after positioning for surgery to make sure that the dependent lung remains ventilated.
In the unusual situation of a large fistula at the level of the carina resulting in preferential gastric ventilation, some authors suggest passing a 2 or 3Fr Fogarty embolectomy catheter through the fistula into the stomach via the rigid bronchoscope; the balloon of the Fogarty catheter is then inflated to occlude the fistula. The tracheal tube is positioned alongside the Fogarty catheter [106]. This may not be a suitable technique if the child is very small or unstable. In such a case, the surgeon should proceed directly to thoracotomy to ligate the fistula as quickly as possible. Alternately, the chest may be opened rapidly and the distal esophagus ligated below the fistula [97]. If the stomach becomes very distended before the fistula is occluded, the tracheal tube should be disconnected intermittently to decompress the stomach via the airway.
Analgesia may be managed using an opioid-based technique such as fentanyl or remifentanil intraoperatively and morphine IV postoperatively, particularly for the child with long-gap EA who will remain ventilated postoperatively. Regional techniques using caudal catheters have been described and are most suitable for low-risk infants who are likely to be extubated in the immediate postoperative period. Many pediatric surgeons prefer a controlled extubation by anesthesia immediately postoperatively to reduce the risk/need for emergency reintubation that may damage the surgical closure.
Blood loss during surgery is usually minimal; intravenous crystalloid such as Ringer’s is ideal and fluid volume of 10–20 ml/kg is usually required. The blood glucose concentration should be measured [24]. Broad-spectrum antibiotics should be given before skin incision and continued postoperatively. Secure intravenous access should be obtained, and some prefer arterial access to monitor blood pressure beat to beat and to sample the blood gases during one-lung ventilation, particularly in infants with significant comorbidity. Neonates with cardiac disease have a greater incidence of critical events such as desaturation or the need for new inotropic support compared with those without cardiac disease as well as a 57 % mortality during the hospitalization for those with ductal-dependent congenital heart disease [87]. These data underscore the need for a preoperative echocardiogram to identify a possible cardiac defect in all neonates with EA/TEF and, if a heart defect is present, to discuss the need for central venous access. Phenylephrine should be prepared to treat a “tet spell” in a neonate with an unrepaired tetralogy of Fallot.
Congenital Diaphragmatic Hernia
Congenital diaphragmatic hernia (CDH) occurs in 1:2,500 live births, with a slight predilection for males. There are two major types: Bochdalek (posterolateral defect) (Fig. 10.6a, b) and Morgagni (anterior) (Fig. 10.7a, b) [110]. The posterolateral defect accounts for 85–95 % cases of CDH and most are diagnosed antenatally. CDH is associated with herniation of the abdominal viscera into the affected hemithorax, with displacement of the mediastinum to the contralateral side (Fig. 10.6a, b). The lung on the side of the hernia is hypoplastic, whereas the lung on the contralateral side is usually normal (if survival is likely) or hypoplastic (if survival is unlikely). The degree of lung hypoplasia and associated pulmonary hypertension are the major determinants of outcome; surgical repair of the diaphragm has a relatively minor contribution to the long-term outcome. Anomalies occur in 40–60 % of neonates with CDH. These include cardiac, neural tube, chromosomal, renal, and genital anomalies and pulmonary sequestrations, as well as malrotation and duodenal atresia [111, 112]. The anterior (Morgagni) defect accounts for only 2 % of diaphragmatic hernias, located retrosternal (at the level of the xiphoid) or anteromedially in the diaphragm (Fig. 10.7a, b). These are often asymptomatic and may not be detected until adulthood [113].



Fig. 10.6
Congenital diaphragmatic hernia (CDH): foramen of Bochdalek defect. (a) This chest radiograph depicts a neonate with congenital diaphragmatic hernia in the left (classic Bochdalek defect) chest with stomach and bowel in the chest, displacing the heart to the right chest. Note the multi-orificed orogastric tube (with side holes) in the esophagus, curving up and across the diaphragm and terminating in the stomach in the left chest. The tracheal tube ends at the thoracic inlet. PICC line enters the right chest and ends in the superior vena cava. (b) A schematic of a neonate with a CDH with bowel present in the left chest; the right lung is compressed and the heart is deviated toward the right chest (Courtesy of Dr. Satyan Lakshminrusimha, Division of Neonatology, Women and Children’s Hospital of Buffalo, Buffalo, NY)

Fig. 10.7
(a, b). Congenital diaphragmatic hernia. Foramen of Morgagni defect. (a) This AP chest radiograph depicts a neonate with congenital diaphragmatic hernia with a loop of bowel that herniated through a defect in the anteromedial (retrosternal) area of the diaphragm (foramen of Morgagni). (b) This lateral chest radiograph depicts a loop of bowel in the immediate retrosternal space (see arrow), rising above the anterior aspect of the diaphragm (Courtesy of Dr. K. Valle, Division of Pediatric Surgery, Women and Children’s Hospital of Buffalo, Buffalo, NY)
Embryology
The diaphragm develops during weeks 4–8 from four embryological elements. A Bochdalek hernia results from failure of closure of the pleuroperitoneal canals during early embryonal life, often with early in-growth of the liver through the defect. The exact cause for CDH remains unknown but may be associated with genetic factors that lead to failure in cell migration, myogenesis and formation of connective tissue, or abnormalities of the retinoid signaling pathway, which is important in the development of the diaphragm. CDH may occur as an isolated abnormality (often associated with a mutation on chromosome 15q26) or occasionally associated with syndromes such as Pallister-Killian, Fryns syndrome, Cornelia De Lange, or Edwards syndrome [111]. Abnormal karyotyping has been reported in 16 % of neonates with CDH, in 4 % of those without anomalies, and 39 % of those with anomalies [114, 115].
Diagnosis
The diagnosis of CDH is made by antenatal ultrasound in ~70 % of cases, due to the presence of intrathoracic bowel loops or stomach, often signaled by a history of maternal polyhydramnios [114, 116]. All cases should be referred to a specialist center. The fetus should be screened for associated anomalies and the family provided with antenatal counseling, particularly if there are features associated with a poor prognosis (see below) [117, 118]. Antenatal MRI scans may provide further prognostic information about lung volume, liver herniation, left ventricular mass, and pulmonary diameter, but is not routine [111].
Postnatally, the neonate may present with an exacerbation of their respiratory distress. Symptoms may range from mild to severe, depending on severity of the CDH. The abdomen is scaphoid as the intestine is in the chest, and breath sounds are reduced on the affected side. The diagnosis is confirmed by X-ray of the chest and abdomen, which helps differentiate from other chest pathologies such as CCAM. A nasogastric tube should be placed before imaging as this may lie or curl above the diaphragm if the stomach is in the chest. An echocardiogram should be sought to delineate associated cardiac abnormalities and to estimate the severity of the pulmonary hypertension [119]. Serial cranial ultrasound should be performed to exclude an intracranial bleed.
Outcomes
Despite the advances in the medical and surgical management of this condition, the overall mortality remains at 21–48 %. However, specialist centers with a large number of cases have better survival rates of up to 80 % live births [120]. Mortality in nonsyndromic infants is primarily related to pulmonary hypoplasia and pulmonary hypertension and, as surrogate markers of severity, the need for ECMO/HFOV or for patch repair [121]. Right-sided, bilateral, or large defects, a lung to head ratio of 1.0 or less, and the presence of the liver in the chest portend worse outcomes, as do prematurity, chromosomal anomalies, severe cardiac defects (particularly transposition of the great arteries or single ventricle physiology), and spinal anomalies [122, 123]. In an effort to establish a standardized scoring system, an international consensus concluded that the size of the diaphragmatic defect (as a surrogate for the severity of pulmonary hypoplasia and hypertension) and the severity of the cardiac defect may predict outcome [124]. ECMO required for more than two weeks or associated with renal complications, or persistent pulmonary hypertension for more than 3 weeks are also associated with high mortality [117, 118, 120, 121].
Morbidity associated with CDH in the longer term includes ongoing respiratory problems with recurrent infections and reduced exercise capacity compared with age-matched peers, neurocognitive defects secondary to neonatal hypoxia or intracranial hemorrhage, and visual impairment and deafness, possibly related to intensive care management. Gastroesophageal reflux is common and may require medical or surgical intervention. Recurrence rates of up to 50 % are reported in CDH especially if an initial patch repair is required. Scoliosis and chest wall deformity may also occur [111].
Prenatal Treatment
Prenatal treatment for CHD has been trialed with varying success. The most promising treatment is fetal endoscopic tracheal occlusion (FETO) with a balloon for babies with poor prognosis based on lung measurements. FETO prevents the egress of lung fluid from the fetal lung and improves lung growth and reduces vascular resistance. The tracheal balloon is either removed before delivery, by ex-utero intrapartum treatment (EXIT), or punctured immediately after delivery by tracheoscopy or percutaneous puncture [125, 126]. Promising results have been reported, but the complication rate is relatively high and may include previously unrecognized conditions such as tracheomegaly or bronchomegaly [127]. Premature delivery contributes to the poor outcomes of FETO [125]. In a randomized controlled trial of FETO, survival in neonates with isolated severe CDH is improved [125, 126, 128].
Medical Management
Medical management of CDH has changed dramatically over the last two decades, from a strategy of early emergency surgery with aggressive hyperventilation to reduce pulmonary hypertension to optimizing the cardiorespiratory status using a “gentle ventilation” strategy to minimize barotrauma that includes minimum peak inspiratory pressures, maintaining spontaneous ventilation and permissive hypercarbia followed by a planned, surgical intervention [129].
Delivery should be planned at or near a center with pediatric surgical and NICU expertise so that the optimal postnatal respiratory support and timely surgical intervention can be provided. The neonate should be allowed to mature to term to permit maximum maturation of the lung. A nasogastric tube should be passed after delivery to decompress the stomach, central and arterial access obtained, and the warming strategies commence with minimal handling. Pulmonary surfactant has not been shown to be beneficial [130].
Since most neonates require some level of ventilatory support after birth, it seems prudent to intubate their tracheas at delivery. The initial ventilation strategy (conventional or high-frequency oscillation) varies among centers in the absence of randomized controlled trials [131]. The aim should be to achieve pre-ductal SpO2 85–95 %, post-ductal SpO2 >70 %, arterial pCO2 45–60 mmHg, and pH > 7.25. If a conventional ventilation strategy is used, the initial settings should include a peak inspiratory pressure (PIP) 20–25 cmH2O, positive end-expiratory pressure (PEEP) 2–5 cmH2O, and a frequency 40–60 breaths per minute with minimum required inspired oxygen to maintain the threshold oxygen saturation. Prolonged use of muscle relaxants is ideally avoided, and the neonate should be allowed to breathe spontaneously between assisted breaths. If HFOV is used, the initial settings should include a mean airway pressure 13–17 cmH2O, frequency 10 Hz, amplitude (∆P) 30–50 cmH2O, and I:E ratio 1:1. Hyperinflation of the lungs should be avoided (<8 ribs on unaffected side on chest X-ray).
Echocardiography should be performed to estimate the pulmonary artery pressure, direction of shunting across the arterial duct/foramen ovale, myocardial contractility, the presence of a congenital heart/vascular defect, and the response to treatment. The oxygenation index (OI) should be calculated (OI = mean airway pressure (cmH2O) × FiO2 × 100/PaO2 (mmHg)). Inhaled nitric oxide (iNO) 10–20 ppm may be indicated if the OI is >20 or the pre-post-ductal oxygen saturation difference is >10 %. The use of iNO is controversial and response should be assessed using echocardiography. Prostacyclin or prostaglandin E1 (PGE1) should be considered if there is no response to iNO. Many units use PGE1 routinely to prevent closure of the arterial duct and to off-load the right ventricle. Sildenafil or milrinone may be indicated if pulmonary hypertension is refractory to treatment or persistent [132], but may cause systemic hypotension [130]. Endothelin receptor antagonists (bosentan) and tyrosine kinase inhibitors (imatinib) are currently under investigation. Fluid boluses may be required if peripheral perfusion is poor or hypotension is present. If cardiovascular instability persists, inotropes may be required to increase the mean arterial pressure to the upper normal range in order to reduce right to left shunting across the arterial duct.
Venoarterial extracorporeal membrane oxygenation (ECMO) may be offered in some centers as temporary stabilization and support in cases of severe cardiorespiratory failure. Specific ECMO criteria vary between centers; current European criteria for ECMO include inability to maintain pre-ductal saturation >85 % or post-ductal saturation >70 %, respiratory or metabolic acidosis with a pH < 7.15, need for aggressive ventilation, or refractory systemic hypotension [133]. The neonate should be >2 kg and >35 weeks gestation, have no lethal congenital abnormalities, have no irreversible organ dysfunction (including neurological injury), and have no contraindication to systemic anticoagulation.
Surgical Considerations
The objectives of surgery in the management of CDH are to reduce the herniated contents safely into the abdomen and repair the defect. Ideally, surgery should be delayed until the neonate is stable, that is, off inotropes (with the possible exception of dopamine) for 24 h. Some have used “stability” criteria to determine the neonate’s eligibility for surgery; however these criteria may be more appropriate for determining the timing, rather than eligibility, for surgery [134]. Surgical intervention is possible while the neonate is on ECMO, although early studies suggested that the mortality was increased because of a greater risk of bleeding. Many centers undertake surgery only after weaning the neonate from ECMO, although some have achieved acceptable mortality rates repairing CDH on ECMO by limiting the use of anticoagulants and using antifibrinolytics [135]. For large defects, a patch repair may be required as a dome shape rather than a flat repair. Surgery may be performed by using an open technique (usually laparotomy) or a minimally invasive technique.
Laparotomy via an upper abdominal transverse incision allows good access to the length of the diaphragm and can be extended easily if further access is required. The abdominal contents are reduced into the abdomen and the defect is identified. The spleen may require gentle finger reduction rather than simple traction on the gastric and colonic attachments, to avoid damage and bleeding. A hernial sac, which is present in 10–20 % of cases, is usually excised during surgery. Pulmonary sequestrations may also be found, either supra- or subdiaphragmatic, and these are also excised. The hypoplastic ipsilateral lung may be seen via the defect in the chest. The diaphragmatic rim should be mobilized and, if possible, a primary repair performed without tension using nonabsorbable interrupted sutures. If the diaphragm is deficient laterally, then the sutures should incorporate a large bite of rib or muscle to prevent recurrence. If the defect cannot be closed without tension or is very large, a patch repair is required, although this technique is associated with worse short-term and long-term outcomes. A variety of nonabsorbable materials have been used, including Gore-Tex®, Marlex®, Dacron®, and Silastic®. Nonabsorbable materials have the advantage of cost, reduced bleeding, and easy handling; however they do not grow with the child and may actually shrink over time. These materials are associated with adhesions, increased recurrence, gastroesophageal reflux, and chest wall deformities [135]. Newer biosynthetic patch materials such as collagen lattices with embedded growth factors (Surgisis®, AlloDerm®) are under investigation, although they may increase the risk of postoperative small bowel obstruction. Techniques that involve muscular flaps have been described, but they are time-consuming and may be associated with increased bleeding and abdominal wall deformity. Myogenic patches may be developed in the future [135]. A chest drain is not used routinely as the underwater drain may cause overdistension of the hypoplastic lung. Such a drain may be inserted at a later date if a clinically significant effusion develops. The advantage of the abdominal approach is that a Ladd’s procedure can be performed at the same time if the position of the duodenojejunal (D-J) flexure is consistent with malrotation and there is the potential for malrotation.
MIS for CDH has been reported using both laparoscopic and thoracoscopic approaches. The laparoscopic approach enables better instrument handling for the diaphragmatic repair. Reduction of contents against the pneumoperitoneum and the subsequent lack of intra-abdominal space after reduction can be challenging. The thoracoscopic approach is preferred by many and has the advantage that the pneumothorax encourages reduction and there is an excellent view of the diaphragm after reduction. The lateral suture placement can be very difficult due to the rigidity and shape of the thoracic cavity. Initial reports of thoracoscopic repair also suggested an early higher recurrence rate [136]. Right-sided CDH, CDH-associated liver herniation, and the need for patch closure may be better suited for an open procedure. As for thoracoscopic repair, carbon dioxide absorption and acidosis can be problematic during MIS [101]. For this reason, an open surgical approach is probably preferred for neonates with CDH and congenital heart disease, for those who required ECMO, or for those who have continuing systemic right ventricular pressures or require significant inotropic support [137].
It is important to avoid a “flat” diaphragmatic repair during primary or domed patch repair. When the herniated chest contents are reduced into the abdomen, the intra-abdominal pressures may increase to cause abdominal compartment syndrome. If there is evidence of significant venous congestion of lower limbs after closure, then an abdominal wall silo should be left as a laparotomy at the site of the abdominal incision, as for gastroschisis repair. This may be closed a few days later.
Anesthetic Considerations
Management of pulmonary hypertension and pulmonary insufficiency and the timing of surgery are primary considerations in these neonates. Surgical repair should only be performed in physiologically stable neonates, ideally when inotropic support is no longer required and the neonate has been weaned off ECMO, usually at 2–6 days after birth [120]. If the child becomes unstable on transfer to or in theater before surgery begins, then surgery should be postponed. Some units perform surgery in the NICU, but it remains a contentious issue (see Chap. 13) [135].
A balanced anesthesia technique that includes opioids such as high-dose fentanyl 20–50 mcg/kg [10], muscle relaxants, and an inhalational anesthetic should be used to preclude a pulmonary hypertensive crisis. Nitrous oxide should be avoided. The ventilatory strategy should be the same as in NICU; an intravenous technique will be required if the child is receiving HFOV or remains dependent on ECMO. Invasive monitoring should be continued from the NICU, with transcutaneous carbon dioxide monitoring to supplement direct measurement of arterial PaCO2, particularly in MIS.
Surgical repair is usually uneventful and blood loss usually limited (special precautions are required if the child remains on ECMO). Hypotension usually responds to fluid boluses of 10–20 ml/kg balanced salt solution or an increase in the infusion rates of inotropes. Hypotension in the presence of an increasing difference between the pre- and post-ductal SpO2 values may indicate an intraoperative pulmonary hypertensive crisis. Simple interventions include increasing FiO2, increasing depth of anesthesia, opioid administration, and correction of the acidosis that are usually effective. iNO should be available in the event of a pulmonary hypertensive crisis unresponsive to these measures. If oxygenation deteriorates intraoperatively, a pneumothorax on the contralateral (good lung) side and an endobronchial intubation must be excluded. At the conclusion of surgery, the neonate should be transferred back to the NICU; the duration of postoperative ventilation is determined by the severity of pulmonary hypoplasia and pulmonary hypertension.
Abdominal Surgery
Inguinal Hernia
Inguinal hernias occur commonly with a childhood incidence of 0.8–4.4 %. Males are affected 8–10 times more than females. Premature infants have an increased risk of hernias with an incidence of 13 % in neonates born at <32 weeks gestational age (GA) and 30 % in infants <1 kg birth weight [138]. This group also has an increased risk of complications such as obstruction and incarceration. Inguinal hernia is also associated with cystic fibrosis, connective tissue disorders, and abdominal wall defects.
Pathophysiology
Inguinal hernias result from the failure of obliteration of the patent processus vaginalis (PPV), which develops during testicular descent. Up to 50–80 % of neonates may have a PPV and remain asymptomatic until bowel or other intra-abdominal contents enter this sac. When that occurs, it is classified as an inguinal hernia. The right side is more frequently affected (60 %) than the left, with 10–15 % of cases occurring bilaterally (with a greater incidence in infants).
Diagnosis
An inguinal hernia is diagnosed clinically with the history or presence of a groin swelling (Fig. 10.8). This may extend into the scrotum on the ipsilateral side. The testis should also be palpated during examination, separate to the groin swelling. The hernia may contain bowel, omentum, or the ovary in females. Examination should ensure that the hernia is reducible; if the hernia is irreducible (i.e., incarcerated), then the hernia immediately becomes a surgical emergency. The majority (60 %) of incarcerated inguinal hernias occur in the first 6 months of life.


Fig. 10.8
Inguinal hernia in a neonate. Close-up of a right inguinal hernia (Courtesy of Dr. YH Lee, Division of Pediatric Surgery, Strong Hospital, University of Rochester, Rochester, NY)
Surgical Management
Inguinal hernias require surgical closure of the PPV. In many centers, hernia repair is performed as an open procedure via an inguinal incision with ligation of the sac after separating it from the vas and testicular vessels. Care must be taken to fully mobilize and ligate the sac to prevent recurrence, while preserving the vas and vessels with minimal surgical trauma. The duration of anesthesia and surgery for inguinal hernia repair in neonates is greater than in older children. The risks of recurrence or testicular atrophy are greater in neonates, particularly if the hernia has become incarcerated.
Laparoscopic repair may also be performed with closure of the internal ring with a nonabsorbable suture. This technique allows assessment of the contralateral side and bilateral repair if indicated, which may be particularly beneficial in infants as there is a 60 % incidence of bilateral hernia or contralateral PPV in infants. There is less dissection of the cord structures, which may result in less damage; laparoscopic hernia repair in infants is a safe procedure with a small complication rate, although long-term outcomes are not yet available [139]. The laparoscopic approach is feasible even in very small neonates, although the ability to tolerate carbon dioxide insufflation is an important factor, and the procedure may be more challenging technically in the very premature or low-birth-weight neonates. Instead of carbon dioxide insufflation, some advocate gasless laparoscopy in neonates thereby obviating the cardiorespiratory effects of a carboperitoneum [140].
Anesthetic Management
The timing of surgery in neonates who require hernia repair remains controversial, particularly in premature neonates; the younger the neonate, the more susceptible they are to postoperative apneas as well as surgical complications. This has to be balanced with the risk of incarceration if surgery is delayed. Many centers plan surgery for premature infants near the time of discharge from the hospital, and others delay surgery until after discharge to reduce the potential for postoperative apnea and the need for prolonged postoperative ventilation [141].
An analysis of data from prospective studies of former preterm infants undergoing hernia repair under general anesthesia in the mid-1980s and late 1990s suggested that the probability of postoperative apnea in premature infants was <1 % at PCA 56 weeks, with negligible risk of postoperative apnea at 60 weeks PCA. The risk factors for postoperative apnea were gestational age at birth, postconceptional age, ongoing preoperative apneas, and anemia (hematocrit < 30 %) [142]. Factors that may reduce the frequency of apneas in premature infants after general anesthesia include regional block (spinal anesthesia) without sedation, an ilioinguinal/iliohypogastric nerve block, administration of intravenous caffeine 10mg/kg, and the avoidance of neuromuscular blockade or potent opioids [143, 144]. Respiratory events after sevoflurane and desflurane anesthesia occur with similar frequencies in premature neonates [145]. Current practice guidelines recommend postoperative apnoea monitoring for neonates/infants who are full term but less than 44 weeks PCA and infants who were premature at birth (e.g., ≤37 weeks gestation) and who are less than 60 weeks PCA, although it has been suggested that this guidance could be relaxed to 46 weeks for former preterm infants without other risk factors for postoperative apnea [146].
Spinal or caudal epidural anesthesia as a sole technique may be associated with fewer postoperative respiratory complications than general anesthesia but has a significant failure rate [146, 147]. The GAS study (general anesthesia versus spinal anesthesia for infants undergoing hernia repair) may yield further evidence to support the choice of anesthesia for hernia repair in this vulnerable group of infants [148].
Pyloric Stenosis
Pyloric stenosis occurs in 1–3:1,000 births and is four to five times more common in males than females and more commonly in firstborn males. The etiology of pyloric stenosis has been elusive, although there is some evidence for a genetic basis for the disease, feeding practices, erythromycin exposure postnatally (or prenatally), sleeping position (prone increases the risk), and possibly infectious moieties [149–152]. The age at which pyloric stenosis presents has been gradually decreasing. Currently, most cases present at 2–5 postnatal weeks, after several days of projectile vomiting [153–155]. Pathological hypertrophy of the inner layer of smooth muscle in the pylorus results in gastric outlet obstruction, which leads to the projectile nature of the vomiting.
Pyloric stenosis is usually an isolated defect. However, in many cases, it is associated with genetic syndromes including Cornelia de Lange and Smith–Lemli–Opitz syndromes as well as chromosome 8 and 17 translocations and (partial) trisomy 9 [150].
Diagnosis of Pyloric Stenosis
The classic symptom of pyloric stenosis is non-bilious projectile vomiting. Term infants are predominantly affected, although pyloric stenosis does occur in premature infants. The diagnosis may be confirmed by clinical examination with visible gastric peristalsis and a palpable pyloric “tumor.” These signs may be more clearly demonstrated with a “test feed.” The classical biochemical picture is one of dehydration with hypochloremic metabolic alkalosis due to loss of gastric acid. Total body potassium may be depleted due to the renal compensation and tubular reabsorption of hydrogen and sodium ions and water in exchange for potassium. However, mounting evidence suggests that neonates with pyloric stenosis present earlier in their disease process, resulting in less severe electrolyte imbalance in the past decade [155, 156]. As a result of the less severe electrolyte imbalance and the increasing reliability of ultrasound to delineate both the thickness and length of the pylori (with a sensitivity of 100 % and specificity of 98 %), ultrasound has supplanted the electrolyte panel as the cardinal criterion for diagnosis of pyloric stenosis [153–155, 157].
Medical Management
This disorder is a medical, not surgical, emergency. Initial management consists of rehydration and correction of any electrolyte imbalance. Depending on the severity of the fluid and electrolyte derangements, 24–48 h preparation may be required, although affected neonates are reaching pediatric surgeons earlier in the disease process currently, reducing the time required to correct electrolyte disturbances [155, 156]. Surgical intervention should not be undertaken until the neonates have been stable medically and the plasma bicarbonate is <28 mmol/l and plasma chloride >100 mmol/l. Infants with severe dehydration may require an initial fluid bolus of 20 ml/kg 0.9 % saline, followed by maintenance fluid with 5–10 % dextrose and 0.45 % saline, provided the plasma sodium is in the normal range. The stomach should be decompressed with a nasogastric tube, and gastric losses replaced ml for ml with intravenous 0.9 % saline and 10 mmol KCL per 500 ml. Potassium should be added to maintenance fluids only after the child begins to pass urine [153].
Surgical Management
The traditional operation for pyloric stenosis is Ramstedt’s pyloromyotomy, an extramucosal longitudinal splitting of the hypertrophied muscle (Fig. 10.9). This was originally described using an upper midline incision but many centers now use a supraumbilical approach to provide better cosmesis. Laparoscopic pyloromyotomy is also widely performed, and a recent randomized controlled study and meta-analysis have demonstrated some benefits from this approach (reduced time to full feed and length of stay), without an increase in postoperative complications although the debate continues regarding the superiority of the laparoscopic over the open approach for pyloric stenosis [158] (Fig. 10.10) [30]. Whether laparoscopic approach includes multiple incisions, a single incision, or a microlaparoscopic (<2 mm diameter instruments) approach [159], it would appear to be the evolving standard for pyloromyotomy.



Fig. 10.9
Pyloromyotomy. The thickened muscle layer of the pylorus can be seen, incised down to the mucosa. To ensure the muscle has been completely incised, laparoscopic alligators distract the walls of the muscle layer. Note the very thick muscular wall that has been peeled off the pylorus. The liver edge is present immediately above the pylorus at the top of the figure (Courtesy of Dr. K. Bass, Division of Pediatric Surgery, Women and Children’s Hospital of Buffalo, Buffalo, NY)

Fig. 10.10
Laparoscopic insufflation of the abdomen. The neonate’s head is at the top of the figure and the legs are at the bottom. During laparoscopic pyloromyotomy, three ports are placed: the largest trocar (5 mm Mini Step) is inserted through the umbilicus, whereas the two smaller graspers are passed laterally through simple skin incisions. The peritoneal cavity is insufflated with carbon dioxide to ~8 mmHg pressure
Anesthetic Considerations
Surgery should only be scheduled after the fluid status and electrolyte concentrations (including pH) have been normalized; otherwise, the child will be at increased risk of postoperative apnea, arrhythmias, and circulatory instability. A nasogastric tube will be in situ before induction of anesthesia. After administering atropine 0.02 mg/kg IV, the nasogastric tube is replaced with a large red rubber catheter in some centers to suction the gastric contents in the supine and decubitus positions, before induction of anesthesia. When complete, the red rubber catheter is removed. The traditional induction technique for pyloromyotomy is a modified RSI, in which cricoid pressure is avoided after consciousness is lost, but gentle mask ventilation continues with 100 % oxygen until laryngoscopy begins. Surveys of experienced pediatric anesthesiologists reveal that fewer than 50 % apply cricoid pressure to infants with pyloric stenosis [160, 161]. In fact, cricoid pressure is difficult to apply correctly in young infants and may distort the airway, complicating laryngoscopy and tracheal intubation [16, 162, 163]. The modified RSI as described is commonly practiced in neonates and young infants with full stomachs to prevent desaturation during the interval between loss of consciousness and securing the airway.
The anesthetic regimen for pyloric stenosis in the neonate presents two offsetting conditions: to facilitate rapid tracheal intubation and recovery from anesthesia in ~30 min, the duration of surgery. IV induction of anesthesia is usually accomplished with IV propofol using a dose that depends on adjunctive medications. If succinylcholine (2 mg/kg) (preceded by atropine) is included in the regimen, then a dose of 2 mg/kg propofol may be used. Following the black box warning by the Food and Drug Administration, many clinicians in the US avoid succinylcholine in young males, so use either a non-depolarising muscle relaxant or other medications to facilitate intubation of the airway. If a nondepolarizing relaxant is used (most commonly rocuronium), then a small dose should be used to preclude difficulty antagonizing its effects after the brief surgery [164, 165]. Suggamadex may be effective for terminating the action of the relaxant, but it is expensive and not available in every country.
Faced with a male with pyloric stenosis, a fast surgeon, and reluctance to use a nondepolarizing muscle relaxant, several other regimens may be used. Some administer a short-acting IV opioid such as fentanyl 1–2 μg/kg or remifentanil followed by a large dose of propofol (3–5 mg/kg) to induce anesthesia and secure the airway. Alternately, others administer sevoflurane in oxygen while preoxygenating the neonate [166], judiciously timing a bolus of IV propofol (2–4 mg/kg) ± a short-acting opioid, to provide optimal intubating conditions.
In preparation for tracheal intubation, a hockey-stick curve to the tracheal tube (a 3.5 uncuffed or 3.0 Microcuff® tube) molded with a stylet to maintain its shape ensures a rapid and successful tracheal intubation, particularly in the absence of a muscle relaxant. Once the airway is secured, a gastric tube may be reinserted with a stopcock attached to permit inflation of the pylori with 20 ml boluses of air to test the integrity of the underlying mucosa.
Delayed emergence after pyloric stenosis is a common and perplexing problem. This has been attributed to several causes including the use of intraoperative opioids. Infiltration of the wounds with local anesthetic is usually sufficient to control the pain after pyloromyotomy, obviating the need for any opioid intraoperatively, although some prefer a short-acting opioid such as fentanyl or remifentanil [167]. Others advocate a regional block such as a rectus sheath block or epidural, depending on the surgical approach [168, 169]. IV or rectal acetaminophen may also provide mild pain relief intraoperatively and postoperatively, without slowing emergence [28]. However, despite avoiding opioids entirely, many continue to experience a very slow to emerge from anesthesia after this surgery. Some have attributed the slow emergence from anesthesia to the use of nondepolarizing muscle relaxants, although one retrospective study disputed this claim, noting that 0.7 mg/kg rocuronium minimally delayed the time to transport to recovery compared with succinylcholine, after a propofol/sevoflurane anesthetic [164, 165, 170]. Insufflating the abdomen in neonates and infants does not mandate the need for a nondepolarizing muscle relaxant. If relaxants were not used for tracheal intubation and are not part of the anesthetic regimen for surgery, then insufflation requires a deep level of anesthesia and controlled ventilation. This usually involves large concentrations of inhalational anesthetics, which could delay emergence unless the least soluble anesthetics, desflurane and nitrous oxide, were used for maintenance. At least one MAC of desflurane is required during insufflation, which corresponds to an end-tidal desflurane concentration of 9.6 % in this age group [171]. To reduce the concentration of inhalational anesthetic required, remifentanil may be added [167]. Antiemetics are not indicated in neonates as the incidence of postoperative vomiting in this age group is very small and surgeons may use ongoing vomiting as a sign of an incomplete repair or complication [172]. As soon as the surgeon desufflates the peritoneum, the inspired concentration of desflurane is reduced to 3 %. When the skin incisions are closed and dressed, all anesthetics are discontinued.
Intestinal Atresias
Pyloric Atresia
Pyloric atresia is an extremely rare condition (1:100,000 live births) representing 1 % of intestinal atresias. Up to 30 % of patients have other associated anomalies including epidermolysis bullosa, aplasia cutis congenita, and esophageal atresia. Presentation is with non-bilious vomiting with a single gastric bubble on abdominal X-ray and no distal gas. Surgery involves a laparotomy to either excise the obstructing membrane or perform a bypass procedure (gastroduodenostomy or gastrojejunostomy) to restore intestinal continuity. The practical considerations are similar to those for duodenal atresia.
Duodenal Atresia
Duodenal atresia or stenosis occurs in 1:5,000–1:10,000 live births with a male preponderance. Half of the patients have associated anomalies, commonly trisomy 21 (30–40 %), malrotation (30–40 %), and cardiac anomalies (20 %). Anorectal and genitourinary anomalies, esophageal atresia, and Meckel’s diverticulum are also associated with duodenal atresia and, more rarely, biliary anomalies. Up to 45 % of babies are born prematurely [173]. Duodenal atresia may be classified as follows (Table 10.3).
Table 10.3
Classification of duodenal atresia
Type I—mucosal diaphragmatic membrane |
Type II—short fibrous cord connecting two ends of the atretic duodenum |
Type III—complete separation of the two ends of the duodenum |
Embryology
Duodenal atresia may be due to abnormal embryological development of the duodenum, pancreas, and biliary tree. Proposed mechanisms include failure of recanalization of the duodenum during the 8–10th week and altered rotation of the ventral analogue of the pancreas resulting in an annular pancreas. The obstruction is distal to the ampulla in the majority of patients (60–85 %). An alternative theory common to all atresias is the possibility of a vascular accident (see jejunoileal atresia below).
Diagnosis
Polyhydramnios occurs in 33–60 % of pregnancies and antenatal ultrasound may demonstrate a “double bubble.” Cardiac abnormalities may also be detected at this time. Postnatally the baby develops bilious vomiting in the majority, although vomiting may be non-bilious if the atresia is proximal to the ampulla. Abdominal X-ray shows the characteristic double bubble with an absence of gas distally. Distal gas may occasionally be seen if the atresia is periampullary with the main pancreatic and accessory duct opening on either side. Gas can then travel via the biliary tree into the distal intestine. The antenatal and abdominal X-ray findings are less clear in duodenal stenosis, which may present later depending on the degree of obstruction. The differential diagnosis of duodenal atresia is malrotation and volvulus, which can have catastrophic consequences if not detected. If there is any doubt about the diagnosis, for instance, if there is distal gas or no antenatal history, an urgent upper gastrointestinal contrast study should be performed to exclude malrotation. If doubt still remains, then an urgent laparotomy should be performed.
Management
Ultimate management is surgical; however the patient should be resuscitated and stabilized as required. A nasogastric tube should be passed with replacement of losses and maintenance fluids given intravenously. Preoperative workup includes a thorough clinical examination and echocardiography to exclude associated anomalies.
Outcomes
There is low reported mortality and morbidity associated with duodenal atresia. Early operative mortality is less than 5 %, predominantly due to complex cardiac anomalies, and long-term survival is 90 %. Morbidity associated with this condition includes gastroesophageal reflux disease, delayed gastric emptying, peptic ulcer disease, duodenal stasis and blind loop syndrome or megaduodenum, and adhesive small bowel obstruction. These complications may not be apparent until much later in life.
Surgical Considerations
A laparotomy and duodenostomy (either end-to-side or Kimura diamond anastomosis) are the primary procedures performed. Access is via a supraumbilical transverse or umbilical (Bianchi) incision. The minimally invasive laparoscopic approach is feasible and safe and is being introduced in some centers, although liver retraction and exposure of the duodenum can be difficult especially in a small infant or in the presence of hepatomegaly. It is important to check that a second duodenal web is not present (seen in 1–3 % of cases), and there are no distal atresias. Intestinal rotation should be checked and malrotation corrected if present. The gall bladder should be visualized for bile. Some surgeons choose to leave a transanastomotic tube in situ although full enteral feeds are usually achieved without this maneuver.
Anesthetic Considerations
Anesthetic considerations relate mainly to anesthesia for laparotomy in the presence of upper gastrointestinal obstruction and coexisting abnormalities, especially complex cardiac anomalies, and prematurity. If the child is otherwise well, the aim should be to extubate at the end of the procedure.
Jejunal/Ileal Atresia
Small bowel atresia or stenosis is a common cause of neonatal intestinal obstruction occurring in 1:3,000 live births [80]. A stenosis is due to a localized narrowing of the lumen without loss of continuity in the intestine or mesentery (Fig. 10.11). Small bowel atresias are classified into four major types [175, 176] (Table 10.4).


Fig. 10.11
Jejunal atresia. At laparotomy, normal jejunum is held up on the right side of the photo. The jejunal lumen narrows at the atresia in the middle of the photograph, with a very small lumen thereafter. The mesentery, which supplies blood to the bowel, is intact (Courtesy of Dr. YH Lee, Division of Pediatric Surgery, Strong Hospital, University of Rochester, Rochester, NY)
Type I—a membrane or web |
Type II—blind ends separated by a fibrous cord |
Type IIIa—disconnected blind ends |
Type IIIb—absence of the superior mesenteric artery resulting in the “apple peel,” “Xmas tree,” or “Maypole” abnormality |
Type IV—multiple segment atresia (“string of sausages”) |
Types II and IIIa occur most commonly. There may be a family history especially in type IIIb atresia. Multiple atresias are not uncommon, with up to 67 % of jejunal atresias and 25 % of ileal atresias having further distal atresias. Chromosomal abnormalities are much less common in the more distal atresias compared to duodenal atresia although 12 % of patients with ileal atresia will also have cystic fibrosis. These patients should have genetic screening and a sweat test performed at an appropriate time postoperatively. There is an association between gastroschisis and small bowel atresias.
Embryology
The most popular theory is that the atresia is a result of an intrauterine “vascular accident.” Interruption of the blood supply results in sterile necrosis and resorption of affected segments. Multiple causes of vascular interruption have been proposed including fetal intussusceptions, midgut volvulus, thromboembolic occlusions, transmesenteric internal hernias, and incarceration as the result of an abdominal wall defect. The use of methylene blue in amniocentesis for twin pregnancies has also been implicated. The insult is felt to occur late (after week 11), and this is supported by the findings of bile, lanugo hair, and squamous epithelial cells from swallowed amniotic fluid distal to the atresia.
Diagnosis
Polyhydramnios may be present prenatally although less common the more distal the obstruction. Dilated bowel lops may be seen on antenatal scans. Presentation after birth is with signs of intestinal obstruction including vomiting (usually bilious), abdominal distension, and failure to pass meconium. Respiratory compromise may occur if the distension is severe, and the baby may require preoperative respiratory support (and hence postoperative support). A plain abdominal X-ray will show dilated bowel loops (number depending on the level of the obstruction). A contrast enema may be performed preoperatively to exclude the differential diagnoses of meconium ileus, Hirschsprung’s disease, and coexisting more distal atresias. Ten percent of neonates present with meconium peritonitis due to antenatal perforation; calcification or meconium pseudocyst may be seen on abdominal X-ray.
Outcomes
The long-term survival for patients with jejunoileal atresia is 84 %. The primary cause of morbidity and mortality is short bowel syndrome or intestinal failure requiring total parenteral nutrition, with associated risk of sepsis and liver disease.
Medical Management
As with all neonatal intestinal obstruction, the initial goal is to stabilize the neonate with nasogastric decompression, nil by mouth, intravenous resuscitation, and maintenance fluids. Investigations should be performed as above.
Surgical Considerations
The most common surgical procedure to localize the atresia is a laparotomy. Once it has been identified, the atresia is excised with a primary anastomosis. There may be significant discrepancy in size between the proximal and distal ends of the atresia, making an end-to-end primary anastomosis challenging. Despite this, a 7:1 discrepancy can be accommodated with meticulous technique and 7-0 suture material. If bowel length is not a problem, then the dilated bowel can be resected back to a more reasonable caliber. If a type IIIb “apple peel” atresia is discovered, particular care must be taken to avoid compromising the retrograde vascular supply from the marginal colic arteries to the remaining distal small bowel. Problems with absorption of feed and short bowel syndrome are also more common with this type of atresia. This has been attributed to the severity of the vascular insult. Given the high risk of multiple atresias, the continuity of the distal bowel should be confirmed by passing a small balloon catheter through the lumen and flushing with either air or saline before the anastomosis is performed. If the neonate is unstable or the distal bowel is significantly compromised, then a proximal stoma and mucous fistula are preferred as a temporizing measure, with restoration of bowel continuity restored when the neonate has fully recovered. The remaining length of small bowel should be measured and documented to help predict and manage neonates with possible short bowel syndrome.
Anesthetic Considerations
These are the same as for any neonate undergoing laparotomy. However, postoperative ventilation may be required after prolonged laparotomy and a significant fluid shift. Long term vascular access may be required for parenteral nutrition.
Colonic Atresia
This is a very rare cause of intestinal obstruction and represents <10 % of all intestinal atresias. A vascular insult is the likely cause of these atresias. These occur when closing abdominal wall defects especially gastroschises secondary to localized vascular interruption. Applying the Grosfeld classification, most are type IIIa or type I. Associated proximal atresias are common (22 %), and right-sided atresias are associated with Hirschsprung’s disease.
Diagnosis
Colonic atresia presents with symptoms of distal obstruction, including abdominal distension, failure to pass meconium, and bilious vomiting. Multiple loops of distended bowel on plain abdominal X-ray confirm the presence of distal bowel obstruction. A hugely distended loop of bowel is often present because of the closed loop obstruction in the presence of a competent ileocecal valve. Contrast enema confirms the location of the most distal atresia.
Management
Preoperative management is the same as for all neonates with intestinal obstruction. This has been discussed above. Early surgical intervention is essential, as the mortality from perforation may reach 100 % if surgery is delayed for more than 4 days.
Surgical Considerations
Surgical options are laparotomy with either formation of decompressing colostomy or primary anastomosis. Although a high incidence of anastomotic leakage and sepsis has been reported previously in neonates undergoing primary anastomosis, more recent reports support this approach. Hirschsprung’s disease should be considered if an anastomotic leak is detected. A stoma is preferred to direct anastomosis, if resection to bowel with a more appropriate caliber results in short bowel syndrome. As per jejunal/ileal atresias, the proximal bowel must be assessed intraoperatively by flushing with air or fluid to identify additional atresias.
Meconium Ileus
Meconium ileus results from the obstruction of the distal small bowel due to thick inspissated meconium. The majority (90 %) are due to intestinal and pancreatic dysfunction secondary to cystic fibrosis (CF). Up to 25 % of neonates with underlying cystic fibrosis will present with meconium ileus. Once the obstruction has been successfully treated, the infant must be tested for CF. The presence of meconium ileus does not predict a worse, long-term outcome from CF, although almost all children with meconium ileus develop pancreatic insufficiency and will require pancreatic enzyme replacement when feeds are introduced [177]. It is important to involve the respiratory physicians early, even though clinical lung disease is very uncommon in neonates.
Diagnosis
Simple meconium ileus presents with distal intestinal obstruction. Plain abdominal X-ray shows multiple loops of dilated bowel with a “soap bubble” appearance in the right lower quadrant (Neuhauser’s sign). This results from the mixing of air and the tenacious meconium. A contrast enema will show a microcolon with pellets of meconium in the terminal ileum.
Complications occur in 50 % of cases. Perforation may occur in the antenatal period if the proximal bowel becomes ischemic or perforates secondary to a volvulus. This will lead to meconium peritonitis and possibly a giant pseudocyst. The neonate may present with a large abdominal mass or meconium may be passed vaginally or be evident in a patent processus vaginalis in the scrotum. Calcification is often evident on a flat plate (X-ray) of the abdomen. Intestinal volvulus or atresias may also occur.
Outcomes
Outcomes are slightly more favorable in simple meconium ileus with a 1-year survival of 92 % compared with 89 % in complicated meconium ileus [177].
Management
The usual resuscitative measures should be performed in conjunction with broad-spectrum intravenous antibiotics, and the neonate should remain nil by mouth. If the diagnosis is consistent with simple meconium ileus, the obstruction may be relieved by nonoperative measures using a hyperosmolar contrast enema (Gastrografin® or Omnipaque®), which may be repeated. Operative intervention is indicated for enema failures and complicated meconium ileus.
Surgical Considerations
Surgical options include manual disimpaction at laparotomy either via a proximal enterotomy or with the intraluminal injection of 4 % N-acetylcysteine. A combination of these two may be required to fully clear the impacted plugs. If this is not possible, a distal loop or double-barreled stoma should be performed, although this is rarely required. Primary laparotomy is performed in complicated meconium ileus; the options are resection and anastomosis or, alternatively, stoma formation. It is essential to ensure that the obstruction into the microcolon is relieved completely if a stoma is not performed. Additional N-acetylcysteine via a nasogastric tube is sometimes advocated. The stoma should be closed as soon as possible to avoid excessive sodium losses from the gastrointestinal tract and from sweat.
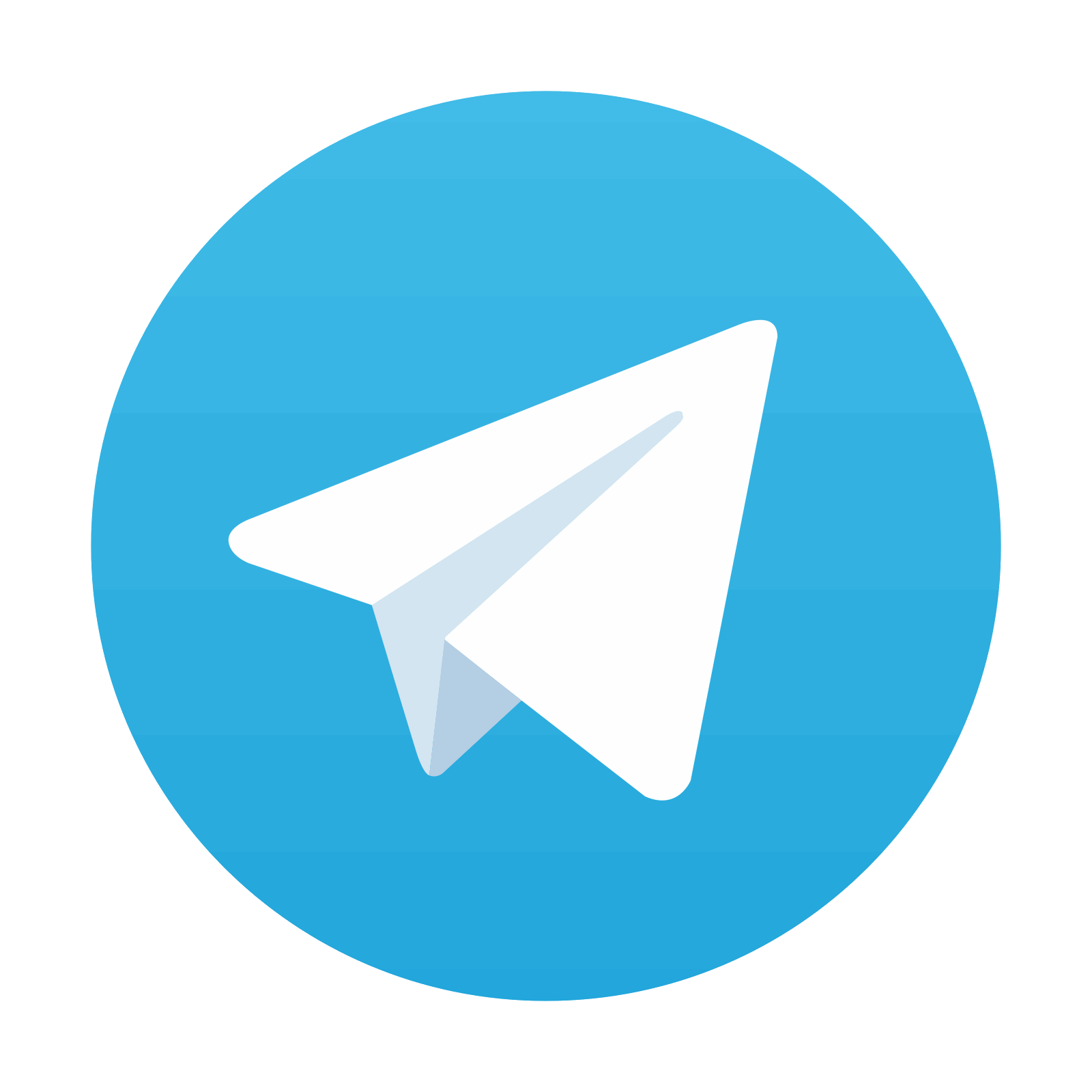
Stay updated, free articles. Join our Telegram channel

Full access? Get Clinical Tree
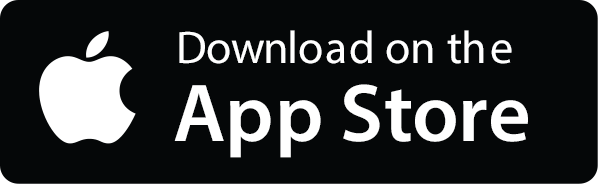
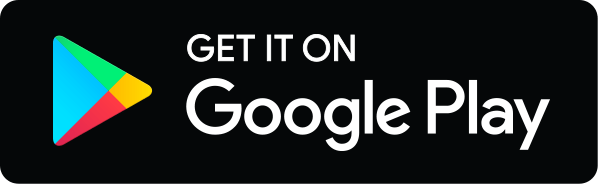