The Unstable Patient: Cardiopulmonary Optimization for Emergency Airway Management
Jarrod M. Mosier, Alan C. Heffner, and John C. Sakles
THE CLINICAL CHALLENGE
The primary goal of airway management is to maintain airway patency and support systemic oxygenation and effective ventilation in patients who cannot sustain these vital functions on their own. Unlike elective intubation in the operating room, intubation in critical illness is often performed because cardiorespiratory physiology is disturbed. As such, these patients are particularly vulnerable to the adverse consequences of apnea and hemodynamic changes associated with induction and positive-pressure ventilation. Furthermore, failure to maintain oxygenation and ventilation during airway management leaves patients at risk of hemodynamic deterioration and cardiac arrest.
In the context of the unstable patient, the emergency airway manager is faced with patients who are hemodynamically compromised or cannot maintain adequate gas exchange prior to intubation. These patients are particularly vulnerable to rapid decompensation during and after the procedure. This chapter will focus on techniques to decrease the risk of deterioration during intubation of the unstable patient. A clear understanding of pertinent principles and physiology helps to optimize the peri-intubation period.
OPTIMIZATION FOR FIRST LARYNGOSCOPY ATTEMPT SUCCESS
Airway management of the critically ill patient is a high-risk situation. Determining the need for, and timing of, intubation requires balancing respiratory and cardiovascular considerations in these fragile patients. The airway manager should optimize conditions to achieve first-pass success, because prolonged or repeated attempts with laryngoscopy are associated with increased risk of adverse events. Box 32-1 summarizes important practical issues and modifications required for safe airway management of unstable critically ill patients.
Considerations and modifications necessary for unstable patients.
• Hemodynamic monitoring and optimizing preintubation status
• Review current hemodynamic status for high-risk features
• Hypotension, SI ≥ 0.8, pulmonary hypertension, RV failure, pericardial effusion
• Establish monitoring for precipitous hemodynamic decline
• Consider invasive, continuous arterial monitoring for high-risk patients
• Confirm adequate IV access for rapid fluid boluses and/or vasopressor infusion
• Empiric fluid loading prior to airway management (i.e., 20 mL per kg crystalloid) in absence of fluid overload or RV failure
• When appropriate, delay intubation to improve hemodynamic status
• Initiation or immediate availability of vasopressor support to treat or avoid hypotension
• Preoxygenation
• Suboptimal preoxygenation resulting from high systemic oxygen extraction, ineffective spontaneous breathing, shunt physiology, or equipment limitations
• Rapid desaturation limiting period of apneic normoxia for laryngoscopy
• Preoxygenation with positive pressure should be considered. If unavailable, standard NRB plus standard nasal cannula up to the flush flow rate should be used
• RSI and drug use
• Greater untoward cardiovascular effects of RSI drugs
• Need for reduced dose sedative-hypnotic agent
• Slower onset of action of RSI drugs
• Awake intubation is an option in some patients
• Postintubation management
• Expected ventilatory requirement should guide intubation plan. Patients with very high minute ventilation may require awake intubation to control their own respiratory drive
• Lung-protective ventilation (≤7 mL per kg IBW) for all patients
• Avoid dynamic hyperinflation and auto-PEEP
• Low-dose titrated analgesia and sedation
Timing of Airway Management
Unstable patients add complexity to the airway management plan. The very procedure intended to secure the airway and improve gas exchange may contribute to patient deterioration when the traditional “A-B-Cs” of resuscitation are rigidly pursued. Prioritization of immediate airway management versus preintubation support is a common clinical dilemma. Three main considerations may assist with the decision:
• What is the reversibility and severity of respiratory compromise?
Knowledge of the underlying pathophysiology inciting respiratory compromise is critical for determining the best course of action. As an example, acute cardiogenic pulmonary edema with reversible precipitants (i.e., uncontrolled hypertension or volume overload) often responds to aggressive medical therapy in minutes, averting the need for intubation. In contrast, hypoxemia due to pneumonia or acute respiratory distress syndrome (ARDS) does not reverse rapidly, and consideration of early intubation is prudent to avoid worsening gas exchange and preintubation conditions. Severe intrapulmonary shunt physiology that accompanies these conditions also contributes to difficult preoxygenation. These patients are at high risk of rapid desaturation during intubation, which can lead to cardiovascular collapse prior to successful endotracheal tube placement.
Noninvasive positive-pressure ventilation (NIPPV) and high-flow nasal cannula (HFNC) are effective methods of managing acute respiratory failure in many patients. However, it is vital to identify patients who are failing these modalities and require tracheal intubation. Sustained high FIO2 requirement (>75%) to maintain oxygen saturation (SpO2) > 92% indicates severe intrapulmonary shunt, and intubation should be strongly considered in the absence of immediate reversibility. Delaying intubation until manifestation of refractory hypoxemia is associated with a high incidence of peri-intubation complications and adverse outcomes.
Acute respiratory failure due to obstructive lung diseases, such as asthma or chronic obstructive pulmonary disease (COPD), often responds to noninvasive ventilation. Mechanical ventilation of the patient with severe obstructive lung physiology is complicated, and intubation is often reserved as a last resort after unequivocal failure of NIPPV and medical support. However, these patients require vigilant monitoring to recognize the early signs of deterioration that help avoid delayed intubation in the setting of severe hypercapneic acidosis.
Patients with ventilatory failure due to a metabolic acidosis present different management considerations. In patients with a metabolic demand that outstrips their ability to compensate because of shock (e.g., sepsis), the metabolic acidosis is often best improved by early intubation to reduce the respiratory work of breathing and oxygen consumption by the respiratory muscles. However, when the metabolic demand outstrips compensatory capability because of organic acid formation, such as diabetic ketoacidosis or salicylate toxicity, maintaining and supporting spontaneous respiration to avoid intubation, given the patient’s ability to protect their airway, is the best course of action. This is due to the physical limitations of the mechanical ventilator in meeting the ventilatory requirements required to compensate for the metabolic acidosis that will not improve with offloading of respiratory muscle work.
• What is the current cardiovascular status and risk of peri-intubation deterioration?
Cardiovascular shock is the final common pathway for many life-threatening diseases. Preintubation hemodynamic instability increases the likelihood of severe complications, including cardiac arrest, during or following intubation. Postintubation hypotension (PIH) complicates up to 25% of emergency intubations and is strongly associated with adverse outcomes including death. Rapid sequence intubation (RSI) and mechanical ventilation can have a substantial negative impact on the already fragile cardiopulmonary status. Structured assessment of cardiovascular status coupled with preinduction hemodynamic preparation are important facets of emergency airway management.
Work of breathing can be substantial and is often underestimated. Patients in shock may expend up to 20% or more of the cardiac output on ventilation, and intubation of patients who are failing cardiovascular resuscitation is frequently advocated to allow redistribution of blood flow to other vital organs. The cause of shock is an important consideration in the decision and timing of intubation in shocked patients. Effects of positive-pressure ventilation on cardiac function vary depending on the underlying cardiovascular state. Positive intrathoracic pressure reduces transmural cardiac pressure and thereby reduces left ventricular afterload. This impact may improve the performance of severe left ventricular dysfunction. In contrast, patients with normal or mildly reduced function suffer greater impact because of impedance of venous return. Prioritizing early fluid resuscitation and use of vasopressor support to maintain systemic pressure and venous return during induction sympatholysis is important for these patients, especially those with prominent vasodilation (i.e., sepsis, cirrhosis, and anaphylaxis).
In contrast to vasodilated shock and left heart disease, induction and mechanical ventilation can precipitate cardiovascular collapse in other forms of shock. Decompensated right heart failure is very sensitive to the increase in pulmonary vascular resistance that is normally induced by mechanical ventilation. Patients with cardiac tamponade preserve venous return via intense peripheral vasoconstriction. Loss of sympathetic tone with induction and initiation of mechanical ventilation are associated with cardiovascular collapse and arrest in patients with both of these conditions, and delaying intubation for effective therapies (including emergency pericardial drainage) is prioritized over early intubation. Bedside echocardiography can be a useful tool when evaluating the hemodynamic profile of a critically ill patient and to predict the hemodynamic response to intubation.
Most of the critically ill patients present in compensated shock with a narrow pulse pressure but sustained normotension. Episodic or sustained hypotension characterizing uncompensated shock is a late sign of hypoperfusion that develops when physiologic mechanisms to maintain normal perfusion pressure are overwhelmed. Mean arterial pressure (MAP) < 65 mm Hg, systolic blood pressure (SBP) < 90 mm Hg, or MAP > 20 mm Hg below baseline are important signs even in the absence of overt clinical hypoperfusion. Peri-intubation cardiac arrest rates complicated up to 15% of patients undergoing emergency intubation in the context of hypotensive shock. Efforts should focus to establish improved hemodynamic stability prior to induction of patients in shock unless immediate intubation is absolutely required.
Unfortunately, SBP is an imperfect single indicator of cardiovascular status, and normal or elevated blood pressure should not be interpreted as an unequivocal sign of adequate perfusion. Shock index (SI), calculated as HR/SBP, is a simple marker of cardiac efficiency that helps identify vulnerable patients despite deceptively normal blood pressure. Elevated SI is associated with cardiovascular deterioration across a range of clinical conditions including emergency intubation. Preintubation SI ≥ 0.8 independently forewarns of peri-intubation hemodynamic deterioration. However, one-third of patients develop peri-intubation deterioration below this threshold, and all patients undergoing emergency intubation should be considered at risk.
Inadequately oxygenated patients are at extremely high risk of desaturation during intubation, which increases the risk of hemodynamic decompensation. Medications and positive-pressure ventilation may also reduce cardiovascular performance and precipitate irreversible decompensation. Both must be titrated carefully to the patient’s cardiovascular status.
Inadequate spontaneous breathing is a late sequela of shock. Respiratory failure in patients with shock, particularly sudden hypoventilation (i.e., bradypnea or apnea), often signifies impending cardiac arrest and requires immediate attention. Prompt respiratory support is indicated but must be coordinated with immediate cardiovascular support. Less severely ill patients may benefit from supplemental oxygen or bag-valve-mask support to optimize preoxygenation, whereas improvement of cardiovascular status is gained through administration of crystalloid and vasopressor support.
• What is the anticipated clinical course?
Many critically ill patients demonstrate a biphasic course wherein early resuscitation slows the spiral of shock and organ dysfunction, only to be followed by deterioration hours later. In most instances, hypotension and malperfusion are improved but not entirely reversed by initial therapy. Tissue edema stemming from volume resuscitation, the progression of end-organ dysfunction (including acute lung injury), cumulative work of breathing, and metabolic debt combine to exhaust physiologic reserve leading to respiratory failure minutes or hours following initial “successful” resuscitation. Frequent reassessment of critically ill patients is required, with particular attention to respiratory status. Increasing the respiratory work or oxygen requirement signals worsening of acute lung injury. Hemodynamic status may also subtly, but progressively, deteriorate, indicated by malperfusion or escalating vasopressor support. Intubation should occur early when this downward cycle is identified, rather than wait for overt cardiovascular or respiratory failure.
Preoxygenation Considerations in the Unstable Patient
Optimizing preoxygenation is more difficult in critically ill patients. Ineffective spontaneous ventilation, decreased pulmonary and systemic perfusion, high systemic oxygen extraction, shunt physiology, and equipment limitations all compromise preoxygenation. Although saturated hemoglobin accounts for the majority of blood oxygen content, systemic oxygenation is regulated (and limited) by cardiac performance. Even with optimal preoxygenation, the rate of desaturation is dependent on cardiovascular status and systemic oxygen extraction. The clinical repercussion is a marked reduction in the period of apneic normoxia to complete intubation. Hypercapnia during intubation also has the potential to exacerbate acidemia, further increasing this risk.
Standard methods of preoxygenation are often inadequate in critically ill patients. Preoxygenation with a non-rebreather (NRB) mask for 3 minutes or eight vital capacity breaths is extrapolated from data in the operating room, where NRB masks create a tight seal and patients are on a closed circuit. Outside of the operating room, NRB masks are rigid, poor fitting without a seal, and rely on filling an attached reservoir with oxygen to increase FIO2 close to 100%. Without the mask seal, room air is entrained around the mask and dilutes the oxygen content provided from the reservoir. High minute ventilation can also easily outstrip oxygen flow, resulting in NRB preoxygenation approximating 50% to 65% FIO2. When oxygen flow is increased to the “flush flow” rate of 40 to 70 L per minute, an FIO2 of nearly100% can be maintained with a standard NRB mask. In addition, patients with shunt physiology exhibit hypoxemia refractory to increased FIO2. For these reasons, preoxygenation with NIPPV is advocated to provide higher FIO2 (via high flow rate to meet demand and tight-fitting mask) and promote alveolar recruitment with positive end-expiratory pressure (PEEP). Newer HFNC devices that provide heated and humidified oxygen at flow rates of 30 to 70 L per minute are useful for preoxygenation in patients who cannot tolerate NIPPV. Approximately 1 cm H2O pressure of PEEP is estimated for every 10 L per minute of HFNC flow, but correlation with PEEP of NIPPV is unclear. Data comparing HFNC devices to standard methods of preoxygenation in critically ill patients have been mixed. Lastly, low-dose inhaled vasodilators such as inhaled nitric oxide or inhaled prostaglandins may decrease ventilation–perfusion mismatch and improve preoxygenation.
Oxygen provided to the upper airway during the apneic period may prolong the safe duration of apnea during laryngoscopy. Continuous oxygen extraction by the pulmonary circulation during apnea creates a gradient by which oxygen delivered to the upper airway diffuses into alveoli. Data on apneic oxygenation in critically ill patients are mixed. However, this low-cost intervention is minimally disruptive during emergency airway management and is advocated in high-risk population.
Systemic oxygenation during intubation is critical. Hypodynamic cardiovascular performance results in significant lag in peripheral SpO2 compared to central arterial oxygenation. The delay is exacerbated by signal averaging of pulse oximetry (see Chapter 8) and accentuated during hypoxia (i.e., starting at the inflection point of the oxyhemoglobin dissociation curve). During acute arterial desaturation, the repercussion of this delay is that central arterial oxygenation can lag the peripheral SpO2 monitor output for up to 60 to 90 seconds. Conversely, SpO2 response may be disconcertingly delayed following successful intubation and 100% oxygen delivery following intubation. Forehead and ear probes are closer to the heart and respond more quickly than distal extremity probes. Forehead reflectance probes are often preferred in critically ill patients for this reason, and they provide more reliable signal detection during hypotension. Limited detection of cutaneous arterial pulsatility generally reduces accuracy of pulse oximetry with SBP < 80 mm Hg.
Hemodynamic Optimization
Most critically ill patients have a disturbance in volume balance, exaggerating the response to induction agents and positive intrathoracic pressure. These volume balance disturbances are precipitated by volume depletion (e.g., hemorrhagic shock), volume overload (e.g., nephrogenic pulmonary edema), vascular compliance abnormalities (e.g., decreased systemic vascular resistance in sepsis), or cardiac dysfunction (cardiogenic pulmonary edema, right ventricular (RV) failure). A thoughtful approach to resuscitation is necessary to guide targeted therapies specifically at the underlying pathophysiology.
Adequate intravenous access should be established to allow for aggressive resuscitation. Second, determination of volume responsiveness should be performed. There are several dynamic assessments of volume responsiveness available to determine if a patient’s cardiac output is likely to respond to a fluid challenge. Although most patients will respond to an empiric fluid challenge, assessment of volume responsiveness should be performed in unstable patients to avoid the untoward effects of volume overload. In patients who have been fluid resuscitated, or are not responsive on dynamic assessment, vasopressor support should be initiated. For patients who are unstable prior to intubation, continuous infusions are preferred over bolus infusions. In patients who are at high risk of PIH, vasopressors should be prepared and readied for rapid initiation.
Given the risk associated with intubation of patients in hemodynamic crisis, close hemodynamic monitoring is indicated. Continuous cardiac monitoring with frequent noninvasive blood pressure recording at least every 3 to 5 minutes should be performed in the peri-intubation period. Continuous monitoring should be considered during the preintubation period to facilitate monitoring of high-risk patients. Regardless of the measurement tool, it should be remembered that blood pressure is not equivalent to blood flow (i.e., cardiac output and oxygen delivery). Progressive bradycardia not associated with hypoxia or laryngoscopy is a frequent sign of severe shock and impending cardiac arrest.
Induction
Drugs commonly used for intubation can be a double-edged sword in the critically ill. They facilitate intubation but can have severe adverse cardiovascular consequences including precipitation of shock and cardiac arrest. Patients with reduced physiologic reserve because of hypovolemia, vasodilation, or abnormal cardiac function are at higher risk of adverse events during airway management. Patients with hypotensive shock represent the extreme example. Most shock states are associated with high sympathetic tone, which serves a compensatory mechanism to maintain critical cardiac output.
Induction agents induce potent sympatholysis and attenuate reflex sympathetic discharge during laryngeal manipulation. Opioids, often given as a preintubation sympatholytic agent to patients with hypertensive emergencies, are contraindicated in patients with compromised cardiovascular status including compensated shock. Any drug that extinguishes endogenous catecholamine response, including sedative-hypnotic agents and neuroleptics, can have similar deleterious impact. Reduction of endogenous sympathetic tone leads to venous and arterial vasodilation with decreased venous return and hypotension. Some anesthetic agents also induce direct myocardial depression.
Drug choice must be considered carefully. Even at reduced doses, sedative-hypnotic induction obliterates endogenous catecholamines with subsequent arterial and venous vasodilation. The reduced pressure gradient for venous return induced by systemic venodilation is compounded by positive intrathoracic pressure upon initiation of mechanical breathing. In some critically ill patients, awake intubation with preservation of spontaneous respiration is the best course of action because of predicted difficulty either with intubation or with mechanical ventilation after intubation. Sedative induction drugs exhibit similar sympatholysis but are essential for facilitation of RSI. Adverse cardiovascular effects are both agent- and dose-dependent. Commonly recommended doses are based on patients with normal hemodynamics and cardiovascular reserve and therefore can be hazardous to critically ill patients. Frank hypotension or compensated shock requires a dose reduction of one-half to one-third of the standard dose. Reasonable sedation and amnesia is ensured with the recommended agents, particularly with proper management of sedation and analgesia in the immediate postintubation period.
Cardiovascular effects vary with sedative-hypnotic agent. Etomidate and ketamine are widely regarded as the most hemodynamically stable induction agents; however, despite their improved cardiovascular effects, both etomidate and ketamine require dose adjustment for administration to shocked patients (e.g., etomidate 0.1 to 0.15 mg per kg or ketamine 0.5 to 0.75 mg per kg). It is better to err on the side of too little rather than too much. Airway managers should anticipate delay in drug onset resulting from dose adjustment and the prolonged circulation time.
Neuromuscular blocking agents (NMBAs) pose little hemodynamic risk and should be dosed normally. Succinylcholine and rocuronium are both hemodynamically stable NMBAs. In patients with identified difficult airway attributes, awake intubation using a flexible endoscope, facilitated by topical anesthesia and limited (or no) sedation, addresses the difficult airway and also avoids the potential hypotension of induction agents. Intubation with succinylcholine alone is unusual but might be required for the obtunded patient with severe shock or impending cardiac arrest who necessitated muscular relaxation for intubation and is unable to tolerate a small dose of induction agent. Intubation without any medications is reserved for arrested or moribund patients (see Chapter 8). However, neither of these situations obviates the need for attention to safe ventilation to minimize the negative cardiovascular impact of positive-pressure breathing.
Postintubation Management
Mechanical Ventilation
Following intubation, positive-pressure ventilation should be initiated with caution. Positive intrathoracic pressure limits venous return to the right heart, which is accentuated during hypovolemia. Pathologic states of tension pneumothorax and auto-PEEP exacerbate intrathoracic pressure and negative hemodynamic effects. Although most clinicians recognize the risk associated with tension pneumothorax, dynamic hyperinflation is much more common. Intentional or inadvertent hyperventilation leads to dynamic hyperinflation if expiratory time limits complete tidal volume (TV) elimination. Dynamic hyperinflation results in retained intrathoracic volume, which ultimately results in positive intrathoracic pressure known as auto-PEEP and impedes venous return. The risk of dynamic hyperinflation is increased with obstructive lung disease, but any patient can develop auto-PEEP under positive-pressure breathing. Unrecognized auto-PEEP can lead to irreversible hypotension and cardiac arrest.
Immediately after intubation, hyperventilation with an inappropriately high rate and TV is particularly common during manual bag ventilation. This is a vulnerable period given the simultaneous action of induction anesthesia. Recognize that most resuscitation bags have 1,500 mL reservoirs and require single-hand ventilation to provide TV approximating 500 mL. Similarly, rapid reexpansion of the bag following breath delivery is not a cue for the next breath, and a clock second hand or counting cadence should be used to ensure that the rate is not excessive. The airway manager needs to be particularly attentive to the risk of manual hyperventilation when other staff provide this support. Specific instruction is required regarding both volume (extent of bag squeeze) and rate (counting cadence, such as “1, 2, 3, 4, 5, breath, 1, 2, 3, 4, 5, breath. . .”). That sufficient time is allowed for complete expiration can be ascertained by simply listening to the patient’s chest during ventilation. The chest should be quiet, representing completion of expiratory airflow, before initiation of the subsequent breath. Interrogation of the ventilator time-flow graphics to confirm return to zero flow at the completion of each breath cycle is a more sophisticated analysis of the same issue.
Mechanical ventilation strategy depends mainly on the underlying pathophysiology. Lung-protective ventilation should be provided with TV ≤ 7 mL per kg of ideal body weight (IBW) for all patients. See Chapter 7.
Postintubation Sedation
Postintubation sedation holds the same potential to induce sympatholysis-related hypotension as induction agents. The first priority is patient comfort and patient-ventilator synchrony. Sedation requirements are frequently overestimated, and analgesia and hypnotics should be titrated to patient condition. Although many prefer benzodiazepines (e.g., lorazepam and midazolam) to propofol because of perceived hypotension risk, appropriately titrated dose of the selected agent is likely most important. Intermittent or continuous opioid infusions are a growing first choice for postintubation sedation. In the absence of painful invasive procedures, light sedation that maintains patient tolerance of tracheal intubation is preferred over deeper sedation, which may worsen hypotension or increase vasopressor requirements. Despite its favorable hemodynamic properties, etomidate should not be used for postintubation sedation because of the risk for severe adrenal suppression.
PIH is a common clinical situation but should not be interpreted as an innocuous consequence of intubation. PIH occurs in one-quarter of normotensive patients undergoing emergency intubation and is severe (SBP < 70 mm Hg) in up to 10% of cases. PIH is independently associated with increased risk of in-hospital death. Whether PIH directly contributes to worse outcome or merely represents a high-risk marker of severe disease is unclear. In either case, the risk associated with PIH warrants an early and organized hemodynamic resuscitation response similar to systemic hypotension (uncompensated shock) unrelated to airway management.
SUMMARY
The ultimate goal with airway management is to maintain adequate systemic oxygenation, ventilation, and perfusion. Unstable patients have altered physiology that makes this goal complicated. Although intubation is an essential part of the resuscitation of a critically ill patient, preintubation management, the intubation technique, medications, and postintubation mechanical ventilation strategy impact peri-intubation hemodynamics that are associated with patient outcomes (Box 32-2). Careful planning, coordinated resuscitation, and peri-intubation management seek to optimize safe intubation and outcomes.
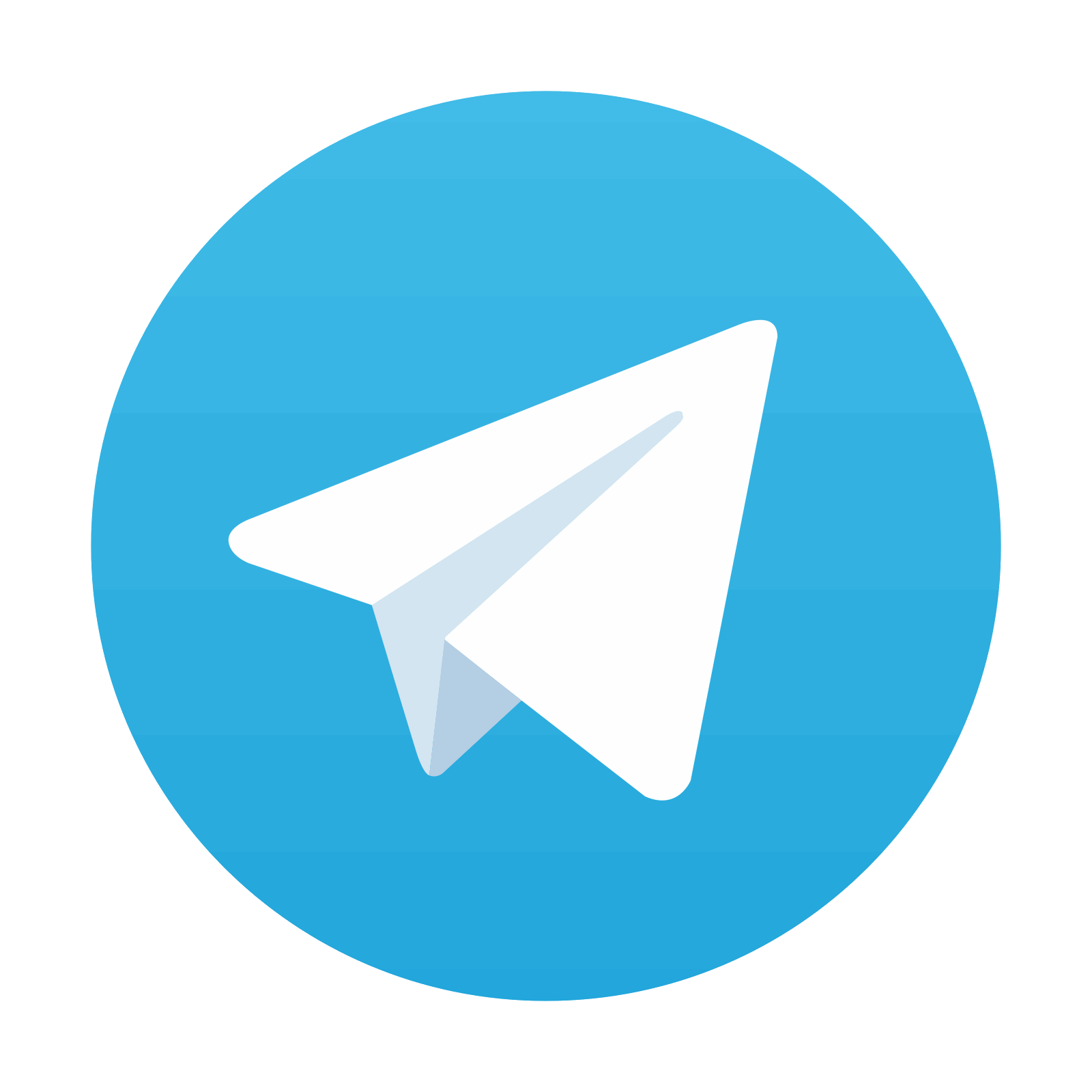
Stay updated, free articles. Join our Telegram channel

Full access? Get Clinical Tree
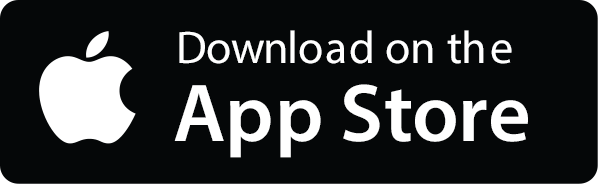
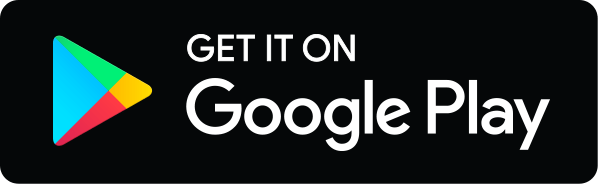