The Treatment of Pain in Neonatal and Pediatric Patients
Constance S. Houck
Joseph D. Tobias
Mary Ellen Tresgallo
K. J. S. Anand
William S. Schechter
During the preceding 50 years, numerous changes, refinements, and advancements have occurred in the understanding and treatment of acute and chronic pain in neonates, infants, and children. This revolution in pediatric pain management began with the rejection of misconceptions and unfounded beliefs that neonates, infants, and children do not perceive and react to painful stimuli because of the immaturity of their peripheral and central nervous system (CNS). These false tenets, compounded by fears of addiction and adverse effects, led to the historical undertreatment of acute and chronic (including cancer-related) pain in children. This chapter focuses on the practical management of pediatric pain whether it be acute, chronic, or cancer-related—categories whose distinctiveness is useful from a didactic perspective, but that frequently overlap in clinical practice (e.g., acute pain associated with diagnostic and therapeutic procedures contributes substantially to cancer-related pain in children).
This chapter begins with an overview of the anatomy and development of the nociceptive system. It does not provide the detail given by Walker in Chapter 30 on developmental aspects of nociception, nor that by Dickenson and Yaksh in Chapters 32 and 33 on pharmacology and neuroanatomy, but aims simply to present a preclinical basis for the clinical approach presented later in the chapter. Next, we outline commonly used techniques for the assessment of pain in infants and children. Because many of the interventions used to treat acute pain are fundamental to the armamentarium for treatment of chronic pain and cancer-related pain, we next describe pharmacotherapy for acute pain along with relevant regional anesthetic techniques. Readers interested to learn more about the latter are referred to the comprehensive account by Dalens in Chapter 27.
Clinical studies have demonstrated that infants and children experience a similar severity of postoperative pain as adults and that even preterm infants demonstrate alterations in physiologic and biochemical markers of stress following painful stimuli (1,2,3,4,5,6,7,8). In fact, increases in biochemical measures of perioperative stress are frequently well in excess of those seen in the adult population (1,8). Inadequate treatment of pain during infancy may have long-lasting consequences including the development of chronic pain syndromes or a heightened sensitivity to subsequent painful stimuli, both of which may persist throughout childhood (2,5,9). The latter portion of the chapter addresses the spectrum of chronic and cancer-related pain in infants and children of all ages.
The Anatomy of Pain and Development of Nociception
Nociceptive systems develop during the second and third trimesters of gestation and continue to mature during the first 2 years of life. Part of the traditional reluctance to aggressively treat pain during the neonatal period and infancy arose from the belief that the pain system was immature during these times. Given the nonverbal state of neonates and infants, they are incapable of reporting and describing the subjective phenomenon of pain. Therefore, it was erroneously concluded that these age groups were also incapable of nociception.
Normal nociception begins in the peripheral nervous system (PNS). In contrast to touch, pressure, heat or cold, there are no specific pain sensors. Rather, pain is sensed by free nerve endings. Cutaneous sensory receptors appear in the perioral area at 7 weeks of gestation, spread to the hands and feet by 11 weeks, and are present throughout all cutaneous and mucous surfaces by 20 weeks of gestation. The sensation of pain is mediated via free nerve endings of Aδ and C fibers. These fibers do not demonstrate fatigue: repeated or continuous stimulation increases the ease of transmission of the impulse. Histologic studies show that the density of nociceptive nerve endings in newborn skin is similar to that in adult skin. More importantly, the neurophysiologic properties of the earliest nociceptors are also similar to those of adult skin. Rapidly adapting pressure receptors are the first to appear during fetal life, followed by the development of slowly adapting pressure receptors, and then rapidly adapting mechanoceptors. The depolarization responses of these receptors to mechanical injury, chemical irritants, and inflammatory mediators are similar to those of adult receptors.
Fetal sensory receptors are located on or close to the skin surface soon after development. As the stratum corneum develops, fetal sensory receptors gradually become subepidermal. Reflex movements to cutaneous perioral sensory stimulation occur as early as the seventh week of gestation; for the rest of the face, palms of the hands, and the soles of the feet by the eleventh week; for the trunk and proximal parts of the arms and legs by the fifteenth week; and for all cutaneous and mucosal surfaces by the twentieth week. The development of these sensory reflexes is preceded by synaptogenesis between afferent fibers and sensory neurons in the dorsal horn of the spinal cord. Myelinated fibers are the first to grow into the developing
spinal cord and form connections with deeper layers of the dorsal horn, with collaterals to neurons in the substantia gelatinosa. Following the ingrowth of C fibers (unmyelinated fibers) and synaptogenesis with superficial dorsal horn neurons, these collaterals undergo developmental degeneration. Nociceptive stimuli in fetal life (and in the extremely premature neonate) are transmitted by myelinated A fibers until the maturation of C-fiber connections.
spinal cord and form connections with deeper layers of the dorsal horn, with collaterals to neurons in the substantia gelatinosa. Following the ingrowth of C fibers (unmyelinated fibers) and synaptogenesis with superficial dorsal horn neurons, these collaterals undergo developmental degeneration. Nociceptive stimuli in fetal life (and in the extremely premature neonate) are transmitted by myelinated A fibers until the maturation of C-fiber connections.
In the first trimester of pregnancy, the development of the spinal cord and CNS begins with the closure of the neural canal. At this time, the dorsal horn begins to appear. Electron microscopic and immunochemical studies demonstrate development of the various neuronal cell types in the dorsal horn with their laminar arrangement, interneuronal connections, and the expression of their specific neurotransmitters and receptors, before 13 weeks of gestation and completion by 30 to 32 weeks of gestation. Initially, the receptive fields of dorsal horn neurons are very large, with extensive overlap between receptive fields of adjacent neurons. As maturation occurs, receptive fields of individual dorsal horn cells progressively shrink to become more precisely defined.
On a cellular level, the transmission of nociceptive impulses through the dorsal horn of the spinal cord is mediated via the release of multiple neurotransmitters, such as substance P, glutamate, calcitonin gene-related peptide (CGRP), vasoactive intestinal polypeptide (VIP), neuropeptide Y, and somatostatin. Modulation of this nociceptive transmission occurs by the release of met-enkephalin from local interneurons and norepinephrine, dopamine, and serotonin (5-HT) from descending inhibitory axons. These descending inhibitory axons originate in supraspinal centers and terminate at all levels of the spinal cord and brainstem. During the first and second trimesters up until the latter half of the third trimester, an imbalance exists between the mechanisms that facilitate and inhibit nociceptive input with the former being favored. Of the nociceptive neurotransmitters, substance P, CGRP, and somatostatin are expressed in the dorsal horn of 8- to 10-week-old human fetuses. Glutamate, VIP, and neuropeptide Y appear at 12 to 16 weeks of human gestation. Modulation of incoming noxious stimuli in extremely premature infants may occur through the local release of met-enkephalin, which is first expressed at 12 to 16 weeks of gestation. However, this mechanism is unlikely to be effective in diminishing the transmission of intensive painful stimuli. In the latter half of the third trimester, with the maturation of the descending inhibitory pathways from supraspinal centers, inhibition of incoming sensory stimuli can occur with the release of dopamine and norepinephrine in the dorsal horn of the spinal cord. These neurotransmitters are first expressed at 34 to 38 weeks of human gestation followed in the postneonatal period by 5-HT.
Conduction of nociceptive impulses to the supraspinal centers occurs via the spinothalamic, spinoreticular, and spinomesencephalic tracts located primarily in the anterolateral and lateral white matter tracts of the spinal cord. Delayed myelination in these tracts was proposed as an index of immaturity of the neonatal CNS and used to support the argument that neonates cannot feel pain or fail to react to nociception as do adults. This argument was widely supported despite the common knowledge that incomplete myelination does not imply lack of function, but merely slows conduction velocity. Additionally, any slowing of central conduction velocity would be completely offset by shorter interneuronal distances that must be traversed in infants, compared to much larger (and longer) adult axons. Nociceptive tracts to the brainstem and thalamus are completely myelinated by 30 weeks of human gestation, and thalamocortical pain fibers are fully myelinated by 37 weeks. The emergence of the thalamocortical connection is of crucial importance for cortical perception, since most sensory pathways to the neocortex have synapses in the thalamus. In the primate fetus, thalamic neurons produce axons that arrive in the cerebrum before midgestation. These fibers remain just below the neocortex until migration and dendritic arborization of cortical neurons are complete and finally establish synaptic connections at 20 to 24 weeks of gestation.
The functional maturity of the cerebral cortex is suggested by the presence of fetal and neonatal electroencephalographic (EEG) patterns and by the behavioral development of neonates. Intermittent EEG bursts in both cerebral hemispheres first seen at 20 weeks of gestation, become sustained at 22 weeks, and are bilaterally synchronous at 26 to 27 weeks of gestation. By 30 weeks, the distinction between wakefulness and sleep can be made on the basis of EEG patterns. Cortical components of somatosensory, auditory, and visually evoked potentials have been recorded in preterm babies before 26 weeks of gestation. Several forms of behavior imply cortical function during fetal life. Well-defined periods of quiet sleep, active sleep, and wakefulness occur even in utero, beginning at 28 weeks of gestation. In addition to specific behavioral responses to pain, neonates have various cognitive, coordinative, and associative capabilities in response to visual and auditory stimuli, attesting to the presence of cortical function. Several lines of evidence suggest that the nervous system as a whole is active during prenatal development and that detrimental or developmental changes in any part can affect the whole.
Recent evidence indicates that selective cortical activation occurs after painful stimuli in preterm neonates (10). Bartocci and colleagues (11), using near-infrared spectroscopy in preterm infants aged 28 to 36 weeks gestation, demonstrated increased blood flow in the somatosensory cortex but not the occipital cortex after venipuncture. In a similar study, Slater and colleagues recorded cortical activation after heel sticks in 18 infants between 25 and 45 weeks gestation (12). No cortical response was noted after tactile stimulation even when this stimulation was accompanied by reflex limb withdrawal. Taken together, these studies imply conscious sensory perception of painful stimuli in preterm newborns.
Thus, it is now well established that even premature human newborns have the functional components of a pain system and are capable of pain perception. In fact, the slow development of the pain inhibitory system suggests that the pain threshold may be lower in preterm neonates than term neonates or older infants. The cutaneous flexor reflex has a lower threshold in preterm neonates than in term neonates or adults (8). Thresholds for the flexor withdrawal reflex are decreased after repeated stimulation or local tissue injury in preterm neonates. Sensitization of this reflex may result from immature segmental or descending inhibition in the spinal cord, the immaturity of other spinal or supraspinal mechanisms, or factors associated with the intensive care environment (e.g., noise) and critical illness. Such sensitization is prevented by topical analgesia applied before local tissue injury (13).
Opioid receptor labeling in the fetal brainstem demonstrates very high densities in multiple supraspinal centers associated with sensory perception. These inhibitory opioid receptors may protect developing neuronal systems from constant overstimulation in the presence of the underdeveloped inhibitory gate-control mechanisms in the dorsal horn of the spinal cord. Brain development in neonatal rats can be significantly altered by exposure to naloxone, but is relatively unaltered after treatment with exogenous opioids.
The magnitude of endocrine-metabolic and other stress responses to invasive procedures or surgical operations is much
greater in neonates than in adults. Neonatal catecholamine and metabolic responses are three to five times those of adult patients undergoing similar types of surgery (2). Pharmacokinetic studies of anesthetic drugs show that higher plasma concentrations are required to maintain effective surgical anesthesia in preterm neonates than in older age groups (2). An additional manifestation of the decrease in pain threshold, known as the wind-up phenomenon, occurs in neonates after exposure to a painful stimulus (1,11,14,15). The wind-up phenomenon results from prolonged responses of neurons in the dorsal horn of the spinal cord (3). The temporal summation of these responses produces a condition of prolonged or recurrent hypersensitivity that is disproportionate to the extent of the original injury (5). During these prolonged periods of hypersensitivity, even non-noxious stimuli (such as those produced by handling, physical examination, checking vital signs, etc.) are perceived as noxious, evoking stress and stimulating the systemic neuroendocrine stress responses (see Chapters 31, 32).
greater in neonates than in adults. Neonatal catecholamine and metabolic responses are three to five times those of adult patients undergoing similar types of surgery (2). Pharmacokinetic studies of anesthetic drugs show that higher plasma concentrations are required to maintain effective surgical anesthesia in preterm neonates than in older age groups (2). An additional manifestation of the decrease in pain threshold, known as the wind-up phenomenon, occurs in neonates after exposure to a painful stimulus (1,11,14,15). The wind-up phenomenon results from prolonged responses of neurons in the dorsal horn of the spinal cord (3). The temporal summation of these responses produces a condition of prolonged or recurrent hypersensitivity that is disproportionate to the extent of the original injury (5). During these prolonged periods of hypersensitivity, even non-noxious stimuli (such as those produced by handling, physical examination, checking vital signs, etc.) are perceived as noxious, evoking stress and stimulating the systemic neuroendocrine stress responses (see Chapters 31, 32).
Pain Assessment
As with any type of treatment or therapy, a method of assessing response is the first step in achieving success. For pain management in infants and children, a means of assessing pain helps not only to determine the severity of pain, but also the child’s response to therapy. Tools for this purpose range from simple, bedside checklists with four to five components that require only 5 to 10 seconds to complete, to complex lengthy surveys that are too cumbersome and time-consuming for use in a busy office practice or hospital setting. Tools initially applied to assess pain in adult patients, which relied on self-reporting, are difficult if not impossible to apply to preverbal patients such as neonates and infants, or cognitively impaired children and adolescents. Although tools have been developed to assess pain in nonverbal or cognitively impaired populations (16,17,18), clinical practice that involves the routine assessment of pain in pediatric patients in a standardized fashion cannot do so in a consistent fashion in the majority of clinical scenarios. Nonetheless, the development and use of broadly applicable scales for pediatric use has recently been encouraged by the mandate of various hospital credentialing boards and regulatory agencies to use the presence of pain management protocols as a benchmark criterion for quality assessment.
Pain assessment tools can be categorized into five broad categories including self-report, observational, physiologic, neurophysiologic, and hormonal-metabolic (changes in stress hormones such as epinephrine, norepinephrine, or cortisol). Pain assessment in the verbal child most commonly relies on self-report of pain intensity by means of a visual analogue score (VAS), as is routinely done in adults. Such scales ask patients to indicate where their pain intensity falls on a straight line from 0 (no pain) to 10 (worst imaginable pain). These techniques rely on the patients’ ability to assess and report their own pain. Variations aimed at being more user friendly and usable in younger children (down to 3–5 years of age) include the use of poker chips, a ladder, colored crayons, or pictures of children in varying degrees of distress. With the poker chip scale, the child expresses pain as a number of red poker chips on a pile (1 to 4). Mild pain would be one poker chip, whereas four poker chips are “the most hurt” the child could have. The pain ladder is a picture of a ladder with nine steps or rungs. At the bottom of the ladder is “no hurt” and at the top of the ladder is “hurt as bad as it could be.” The child is asked to point at the place on the ladder that indicates the amount of pain he is experiencing. The severity of pain can also be expressed by selecting a crayon from a spectrum of colors, with red indicating severe pain and blue indicating little or no pain. However, the use of colors to express pain may have some variability among various ethnic groups, as the association of blue with calm or no pain and red with pain does not cross all ethnic groups. Alternatively, the child can use a Faces scale first described by Bieri and co-workers (17). The scale uses photographs of a child in various degrees of distress. One advantage of the Faces scale is that it is available in various versions for different ethnic groups. Although the Faces scale is meant to be used as a self-report type of scoring system, some centers have modified its use and used the Faces scale as an observational tool. In this application, the health care provider assesses the child and selects the face corresponding to the intensity of pain that they believe the patient is manifesting. A revised version of the Bieri Faces Scale (FPS-R) that has six computer-generated face drawings rather than the original seven (allowing an easier comparison with the most commonly used 0–10 VAS scale) was subsequently found to correlate very closely with VAS for acute pain in hospitalized children from 4 to 12 years of age (19) (Fig. 47-1).
![]() Figure 47-1. The Faces Pain Scale–Revised. From Hicks CL, von Baeyer CL, Spafford PA, et al. The Faces Pain Scale-Revised: Toward a common metric in pediatric pain measurement. Pain 2001;93:173–183. |
In the pediatric population acute illnesses, cognitive states, or very young age may preclude the use of self-report scales. Assessment tools have been described and validated for various patient populations including neonates (20,21), preterm infants (22,23,24,25), and patients with cognitive impairment. The latter group of patients, a growing subgroup of the pediatric patient population, has received significant attention in the past 5 to 10 years and now benefits from a number of options for pain assessment. The noncommunicative children’s pain checklist (NCCPC-PV) was developed specifically for use in this population (23). It includes the grading of several specific behaviors such as vocalization, socialization, facial expression, activity, body and limb positioning, and physiologic signs that have been shown to be indicative of pain in children with cognitive impairment. Alternatively, scales such as the Face Legs Activity Cry and Consolability (FLACC) scoring system, which is used for preverbal children, can be modified for cognitively impaired children by the addition of specific descriptors and parent-identified behaviors for each individual patient (24). These tools have been shown to have excellent interobserver reliability and are quick and easy to use even in a busy clinical practice (Table 47-1).
Physiologic parameters applied in pain assessment include heart rate, blood pressure, respiratory rate, oxygen saturation, palmar sweating, or changes in pupillary size. However, factors other than pain may alter these physiologic parameters or their responses to the painful stimulus. For example, the use of
blood pressure elevation as an indicator of pain in a critically ill infant or child in an intensive care unit (ICU) is precluded when blood pressure is low due to a comorbid disease process. Observational tools rely upon assessment of stereotypic behaviors that suggest pain: facial expression, body positioning, and the presence or absence of crying. One such tool is the Children’s Hospital of Eastern Ontario Pain Score (CHEOPS) developed by McGrath and colleagues (18). This scale assigns a score of 0 to 2 for six categories including cry, facial expression, verbal complaints of pain, position of the torso, whether the child is touching the painful site, and position of the legs.
blood pressure elevation as an indicator of pain in a critically ill infant or child in an intensive care unit (ICU) is precluded when blood pressure is low due to a comorbid disease process. Observational tools rely upon assessment of stereotypic behaviors that suggest pain: facial expression, body positioning, and the presence or absence of crying. One such tool is the Children’s Hospital of Eastern Ontario Pain Score (CHEOPS) developed by McGrath and colleagues (18). This scale assigns a score of 0 to 2 for six categories including cry, facial expression, verbal complaints of pain, position of the torso, whether the child is touching the painful site, and position of the legs.
Table 47-1 FLACC behavioral scale | |||||||||||||||||||||||||||||||
---|---|---|---|---|---|---|---|---|---|---|---|---|---|---|---|---|---|---|---|---|---|---|---|---|---|---|---|---|---|---|---|
|
The last category of pain assessment tools use neurophysiologic and metabolic-hormonal changes. Both are generally restricted to research protocols and have limited applicability for everyday pediatric pain management. Neurophysiologic monitoring is the least well known and least well studied of pain assessment tools. Potential neurophysiologic measures include ECG monitoring and waveform analysis, pupillary responses, brainstem evoked responses, galvanic skin changes, and perhaps even metabolic changes in the subcortical areas of the brain and brainstem. Hormonal-metabolic changes such as alterations in plasma levels of stress hormones including epinephrine and cortisol have been used to study pain management regimens for patients with acute illnesses or those undergoing major surgical procedures (1,4,8). However, blood cortisol levels may decline acutely in patients given opioids, regardless of whether analgesia is achieved. Such a decline is simply the manifestation of a well-defined endocrine feedback loop in which exogenous opioids inhibit pituitary-adrenal activation just as they inhibit pituitary-gonadal function (see Chapter 40).
The Pharmacologic Management of Acute Pain
Factors that influence the choice of regimens for pain management in infants and children include the health care provider’s assessment of the severity of pain, the patient’s age, the setting in which the pain will be managed (outpatient versus inpatient), and the presence of comorbid disease. Pain treatment regimens should incorporate a graded, stepwise approach similar to that applied for cancer-related pain (26). This graded or ladder approach includes the use of a nonsteroidal anti-inflammatory agent (NSAID), acetaminophen, or salicylate for the treatment of mild pain; addition of an opioid commonly used for moderate pain (oxycodone, hydrocodone, codeine) to one of the above agents; and the use of intravenous (IV) opioids or regional anesthesia for acute moderate to severe pain. An initial assessment of the severity of pain (mild, moderate, or severe) is made to guide initial therapy and the treatment escalated as needed. A second consideration in the treatment of acute pain must be the setting in which the pain is treated. Non-IV routes (e.g., oral) are needed for outpatient management, whereas the inpatient setting offers an infrastructure appropriate for administration of parenteral opioids.
An example of this graded approach to the treatment of pain includes treatment with a nonopioid analgesic agent (acetaminophen, acetylsalicylic acid, or an NSAID such as ibuprofen) for mild pain such as that following a surgical procedure on soft tissue or mild pain from a medical illness such as pharyngitis or otitis media. Moderate pain such as that following a fracture or an orthopedic procedure on bone can usually be controlled in the outpatient using a combination of acetaminophen, acetylsalicylic acid, or an NSAID such as ibuprofen together with a weak opioid (e.g., an acetaminophen with codeine preparation). Severe pain such as that accompanying a sickle cell vaso-occlusive crisis, major burns, or after a major surgical procedure (e.g., thoracotomy or an exploratory laparotomy), generally requires either a regional anesthetic technique and/or parenteral opioids.
Mild to Moderate Pain in the Outpatient
With tissue disruption and lysis of cell membranes, fatty acids are released and metabolized to prostaglandins, which results in local inflammation and pain through the stimulation of the free nerve endings of Aδ and C fibers. NSAIDs, acetaminophen, and salicylates inhibit the enzyme cyclooxygenase (COX), blocking prostaglandin formation. In contrast to opioids, prostaglandin synthesis inhibitors demonstrate a
ceiling effect, so that once a therapeutic plasma concentration is achieved, increasing the dose does not improve analgesia but may increase the incidence of adverse effects. The majority of such agents are available as over-the-counter medications and represent an effective and relatively safe means of controlling mild to moderate pain. When evaluated in a cohort of pediatric patients with recent musculoskeletal injury, ibuprofen (10 mg/kg) was shown to be superior to either acetaminophen (15 mg/kg) or codeine (1 mg/kg) in reducing pain scores from baseline and in providing effective analgesia, defined as a VAS less than 30 (0–100 scale) (27).
ceiling effect, so that once a therapeutic plasma concentration is achieved, increasing the dose does not improve analgesia but may increase the incidence of adverse effects. The majority of such agents are available as over-the-counter medications and represent an effective and relatively safe means of controlling mild to moderate pain. When evaluated in a cohort of pediatric patients with recent musculoskeletal injury, ibuprofen (10 mg/kg) was shown to be superior to either acetaminophen (15 mg/kg) or codeine (1 mg/kg) in reducing pain scores from baseline and in providing effective analgesia, defined as a VAS less than 30 (0–100 scale) (27).
Table 47-2 Oral dosing guidelines for commonly used nonopioid analgesics | ||||||||||||||||||||||||||||||||||||
---|---|---|---|---|---|---|---|---|---|---|---|---|---|---|---|---|---|---|---|---|---|---|---|---|---|---|---|---|---|---|---|---|---|---|---|---|
|
Recent pharmacokinetic and pharmacodynamic data have provided the clinician with ample information to suggest appropriate dosing guidelines for the pediatric population (Table 47-2). Although salicylate use in pediatrics has declined following recognition of its association with Reye syndrome, choline magnesium trisalicylate combines the analgesic properties of a salicylate with limited effects on platelet function, thereby allowing its use in patients with qualitative and quantitative platelet issues.
Given acetaminophen’s safety profile and potential concerns with both salicylates and the NSAIDs in the pediatric population, it is the most frequently used medication from this group. Recent studies in preterm neonates and infants have provided useful guidelines for treatment with acetaminophen in neonates as young as 28 weeks of gestational age (Table 47-3) (28).
Several potential concerns with the NSAIDs, in particular ibuprofen, have recently been raised but the supporting evidence is conflicting. Although NSAID use has been reported to result in an increased incidence of invasive group A streptococcal disease (necrotizing fasciitis) with varicella infections, other investigations have failed to support this association (29,30). Additional concerns with the NSAIDs include alterations in renal blood flow resulting in acute renal failure in the setting of dehydration (29), as well as the longstanding concern that NSAIDs are hazardous in patients with asthma. In a prospective, randomized trial, the need for an outpatient visit for asthma was less frequent with ibuprofen than acetaminophen when used to treat fever in children with underlying asthma who had no known sensitivity to salicylates (31). Further evidence for the safety of ibuprofen is provided by Lesko and colleagues in a prospective, randomized trial of 27,065 febrile children younger than 2 years of age. The children were randomized to receive acetaminophen (12 mg/kg), ibuprofen (5 mg/kg), or ibuprofen (10 mg/kg) for the treatment of fever. The authors reported no statistical difference in adverse effects, including acute GI bleeding, acute renal failure, anaphylaxis, Reye syndrome, asthma, bronchiolitis, and vomiting/gastritis among the three groups. Although not statistically significantly different from acetaminophen, three patients in the ibuprofen group were hospitalized for GI bleeding, giving a risk of 17 per 100,000. The authors caution that although they noted no difference in the adverse effect profile of these two agents, the study did not evaluate long-term administration nor examine the use of both agents simultaneously. (See below for an expanded discussion of the adverse effect profile of the NSAIDs.)
The role of COX inhibitors in the treatment of acute pain includes their use as the sole agent for mild pain, their combination with opioids for oral administration in moderate pain, and their addition to parenteral opioids and regional anesthetic
techniques for severe pain. In the latter situation, their use does not eliminate the need for opioids, but rather provides adjunctive analgesia and a reduction of opioid requirement. As the majority of opioid-related adverse effects are dose-related, modalities that decrease total opioid consumption significantly may decrease or prevent opioid-associated adverse effects.
techniques for severe pain. In the latter situation, their use does not eliminate the need for opioids, but rather provides adjunctive analgesia and a reduction of opioid requirement. As the majority of opioid-related adverse effects are dose-related, modalities that decrease total opioid consumption significantly may decrease or prevent opioid-associated adverse effects.
Table 47-3 Recommendations for administration of acetaminophen to preterm and full-term infants* | ||
---|---|---|
|
Although oral administration is used most frequently, this route of administration can be difficult in young children, who may refuse or spit out medication whose taste they find objectionable. Oral administration also has a slower onset and lower bioavailability than parenteral administration, nor can it be used effectively in children with gastrointestinal symptoms such as vomiting, ileus, or abdominal pain. Because they are available in several preparations suitable for use in pediatric patients of all ages including tablets, capsules, chewable tablets, elixirs, and infant drops, acetaminophen and ibuprofen are the most commonly prescribed medications of this group. Acetaminophen is also available in suppository form and sustained-release tablets. Intravenous acetaminophen products (the prodrug, Propacetamol which is metabolized to acetaminophen and recently released pure acetaminophen form) are available outside of the United States.
Recent studies in children older than 1 year have demonstrated that the initial dose for rectal administration of acetaminophen should be 40 mg/kg to achieve an analgesic plasma concentration of 10 to 20 μg/mL (32). Anderson and colleagues published recommendations for dosing of acetaminophen in neonates and infants by analyzing individual data from six pediatric acetaminophen dosing studies (28). Based on these studies, the route of administration, and the type of product (oral elixir, suppository, etc.), the authors formulated a helpful table that provides suggested initial doses, subsequent doses, and a dosing interval based on the infant’s gestational and chronological age (Table 47-3).
Several options are available for the timing of the administration of these agents. In the perioperative setting, acetaminophen (15 mg/kg) or ibuprofen elixir (10 mg/kg) can be combined with the oral premedication midazolam (33). This technique allows the medication to achieve a plasma concentration that will provide effective analgesia by the time of awakening, and also masks the unpleasant taste of the IV preparation of midazolam when it is given orally. An alternative to preoperative administration is placement of an acetaminophen suppository (40 mg/kg) following anesthetic induction. A third option is postoperative administration of either ibuprofen or acetaminophen once the child complains of pain in the recovery room. This combination technique can also be used for outpatients with acute pain. This latter option is less desirable in the perioperative setting, since the onset of activity of any of these agents following oral or rectal administration is 20 to 30 minutes. Acetaminophen remains a cornerstone in the perioperative setting due to its lack of platelet effects, raising no additional concerns of increased intraoperative or postoperative bleeding.
When administering or prescribing acetaminophen, the health care provider must ensure that the infant or child is not receiving acetaminophen in other forms, such as in over-the-counter cold medicines. Additionally, given that several different acetaminophen preparations are available from numerous manufacturers, it is equally important to ensure that parents and health care providers know the amount of acetaminophen or ibuprofen in the specific product. The most common cause of acetaminophen toxicity in patients younger than 10 years of age remains inadvertent parental overdosing (34).
For outpatient treatment of moderate pain or when the first step of the pharmacotherapy ladder fails to control mild pain, the NSAIDs, aspirin or acetaminophen can be combined with an opioid (codeine, oxycodone, or hydrocodone). Several such combination preparations are available in both liquid and tablet formulations, offering a wide range of choices for the pediatric patient of all ages. For younger patients, acetaminophen with codeine elixir containing 120 mg of acetaminophen and 12 mg of codeine per 5 mL is a frequently chosen option, with dosing based on the codeine component (0.5–1.0 mg/kg every 4–6 hours). Tablet preparations are also available that contain 325 mg of acetaminophen with 15 mg of codeine (Tylenol #2, Ortho-McNeil Pharmaceutical, Raritan, NJ), 30 mg of codeine (Tylenol #3, Ortho-McNeil Pharmaceutical, Raritan, NJ), or 60 mg of codeine (Tylenol #4, Ortho-McNeil Pharmaceutical, Raritan, NJ).
Recent investigations have questioned the efficacy of codeine for analgesia, as codeine is dependent on hepatic metabolism to morphine for a significant part of its analgesic effect. Williams and colleagues reported that 47% of pediatric patients had genotypes associated with a reduction of the activity of the enzymes necessary for the conversion of codeine to morphine (35). In 36% of the patients, neither morphine nor its metabolites could be detected after codeine administration. The authors concluded that diminished ability to metabolize codeine may be more common than previously reported and result in inadequate analgesia. Codeine is also available as a parenteral formulation, which although marketed for subcutaneous administration, has also been used for IV administration—a practice that is not recommended due to the potential for allergic and anaphylactoid reactions. Although health care practitioners may choose codeine over other opioids because of a misconception that it has less sedative and/or respiratory depressant effects, opioids, when used in equipotent doses, produce equivalent degrees of sedation and respiratory depression.
Alternatives to codeine for oral administration include oxycodone or hydrocodone preparations. As these agents are not dependent on hepatic microsomal enzyme activity for metabolism to active moieties, there should be less genetic contribution to variation in their pharmacokinetics. These opioids are also available in both liquid and tablet forms in combination with either acetaminophen or acetylsalicylic acid. Newer products include a tablet preparation including ibuprofen with either hydrocodone or oxycodone. The dose of these preparations should be based on their oxycodone or hydrocodone component, starting at 0.1 to 0.15 mg/kg (maximum starting dose 5 mg) every 4 to 6 hours. Regardless of the preparation used, with dose escalations, the amount of acetaminophen may exceed the maximum recommended daily dose of 60 to 90 mg/kg. Therefore, if higher doses of the opioid component are needed, preparations that contain codeine, oxycodone, or hydrocodone without the acetaminophen or aspirin should be used to avoid toxicity. A sustained-release formulation of oxycodone (OxyContin, Purdue Pharma LP, Stamford, CT) is also available. Its sustained release maintains an analgesic plasma concentration during a dosing interval of 8 to 12 hours. Although used occasionally to achieve a baseline steady-state plasma concentration of opioid to provide analgesia following major operative procedures, the inability to titrate a long-acting medication to match fluctuating analgesic requirements, and a lack of data regarding its pharmacokinetics in pediatric-aged patients, the risk–benefit ratio of such regimens must be considered (36).
Another option to control mild to moderate pain in the outpatient setting is Tramadol (Ultram, Ortho-McNeil, Raritan, NJ) (37,38,39,40). Tramadol’s analgesic effect results from several potential mechanisms including opioid agonism at the μ opioid
receptor, antagonism of the N-methyl-D-aspartate (NMDA) receptor, and inhibition of norepinephrine and 5-HT reuptake in the dorsal horn of the spinal cord. Despite the initial suggestion that there was limited abuse potential, clinical experience has demonstrated an abuse potential that may be similar to that of other “weak opioids.” Tramadol’s potency is roughly equivalent to that of meperidine. Worldwide, it is available as an injectable solution, suppository, liquid, and tablet. Tablets containing 50 mg of tramadol or the combination of 37.5 mg of tramadol with 325 mg of acetaminophen (Ultracet) are the only two preparations available in the United States. The 50-mg tablet is scored and can be cut in half, thus allowing its administration to smaller pediatric patients. The manufacturer’s recommendations for dosing include 0.5 to 1.0 mg/kg (initial maximum dose of 50 mg) every 3 to 4 hours as needed. However, doses up to 2 mg/kg have been used in some pediatric trials. To date, there have been a limited number of reported clinical trials of tramadol in the pediatric population. Viitanen and Annila compared the analgesic efficacy of an IV intraoperative dose of tramadol (2 mg/kg) with placebo in 80 children following outpatient surgery (adenoidectomy) (40). There was a decrease in the need for rescue opioid analgesia in the recovery room in patients receiving tramadol. Forty-five percent of children receiving tramadol required no supplemental postoperative analgesia, compared with 15% of children receiving placebo (p = 0.003). There were no differences in adverse effects and recovery times. Rose and co-workers evaluated the efficacy of oral tramadol in a cohort of 113 children, ranging in age from 7 to 16 years (38). The study was limited to patients who weighed at least 20 kg and were expected to require analgesia for 7 to 30 days. Dosing was initiated with 1 mg/kg and increased up to 2 mg/kg as needed. Tramadol analgesia was rated as very good or excellent by 69% of parents and 70% of patients. The authors concluded that tramadol was well tolerated and provided effective pain relief. Adverse events were rated as mild or moderate, and similar in incidence to that seen with other oral opioids.
receptor, antagonism of the N-methyl-D-aspartate (NMDA) receptor, and inhibition of norepinephrine and 5-HT reuptake in the dorsal horn of the spinal cord. Despite the initial suggestion that there was limited abuse potential, clinical experience has demonstrated an abuse potential that may be similar to that of other “weak opioids.” Tramadol’s potency is roughly equivalent to that of meperidine. Worldwide, it is available as an injectable solution, suppository, liquid, and tablet. Tablets containing 50 mg of tramadol or the combination of 37.5 mg of tramadol with 325 mg of acetaminophen (Ultracet) are the only two preparations available in the United States. The 50-mg tablet is scored and can be cut in half, thus allowing its administration to smaller pediatric patients. The manufacturer’s recommendations for dosing include 0.5 to 1.0 mg/kg (initial maximum dose of 50 mg) every 3 to 4 hours as needed. However, doses up to 2 mg/kg have been used in some pediatric trials. To date, there have been a limited number of reported clinical trials of tramadol in the pediatric population. Viitanen and Annila compared the analgesic efficacy of an IV intraoperative dose of tramadol (2 mg/kg) with placebo in 80 children following outpatient surgery (adenoidectomy) (40). There was a decrease in the need for rescue opioid analgesia in the recovery room in patients receiving tramadol. Forty-five percent of children receiving tramadol required no supplemental postoperative analgesia, compared with 15% of children receiving placebo (p = 0.003). There were no differences in adverse effects and recovery times. Rose and co-workers evaluated the efficacy of oral tramadol in a cohort of 113 children, ranging in age from 7 to 16 years (38). The study was limited to patients who weighed at least 20 kg and were expected to require analgesia for 7 to 30 days. Dosing was initiated with 1 mg/kg and increased up to 2 mg/kg as needed. Tramadol analgesia was rated as very good or excellent by 69% of parents and 70% of patients. The authors concluded that tramadol was well tolerated and provided effective pain relief. Adverse events were rated as mild or moderate, and similar in incidence to that seen with other oral opioids.
Tramadol has a longer half-life (6–7 hours) than other previously discussed oral opioids agents, and its active metabolite has a half-life of 10 to 11 hours. The active metabolite is renally excreted and can accumulate in patients with renal insufficiency or failure, thereby making it a poor choice in that setting. Despite tramadol’s longer half-life, Viitanen and Annila did not observe a decrease in analgesic needs after discharge when tramadol was compared with placebo. The adverse effect profile of tramadol is similar to that of other opioids; however, as with meperidine, seizures may occur with large doses or in patients with renal failure (41).
One advantage of tramadol over other “weak opioids” suggested by preliminary data is that there may be less respiratory depression with equipotent doses of tramadol (42). In a randomized double-blind, placebo-controlled study, Bosenberg and Ratcliffe evaluated the respiratory effects of IV tramadol (1 or 2 mg/kg) with 1 mg/kg of pethidine (meperidine) during halothane anesthesia in children, ranging in age from 2 to 10 years. Respiratory rate decreased by 1.7 ± 1.8 breaths/minute in the placebo group, by 7.3 ± 3.6 breaths/minute after 1 mg/kg of tramadol, and by 11.4 ± 4.9 breaths/minute after 1 mg/kg of pethidine. The decreases in respiratory rate were accompanied by an increase in end-tidal carbon dioxide (CO2) concentration. Thirteen episodes of apnea occurred with pethidine, 11 of which required naloxone, whereas none were noted after either dose of tramadol. In addition to its limited effect upon respiratory function, there appeared to be an analgesic advantage for the larger dose of tramadol during the first 6 postoperative hours, as the proportion of patients requiring supplemental analgesia was greater for pethidine than 2 mg/kg of tramadol.
Moderate to Severe Pain in the Inpatient
In the inpatient setting, the options for acute pain management expand to include the use of parenteral prostaglandin synthesis inhibitors and the strong opioids. Even when the choice is made to escalate pain therapy to include parenteral opioids, the prostaglandin synthesis inhibitors can be used to lower postoperative opioid requirements and thereby decrease opioid-related adverse effects. Although there has been a significant amount of favorable literature regarding the use of the parenteral NSAID, ketorolac, it may be that less expensive alternatives including oral ibuprofen and acetaminophen may provide equal benefit. These potential benefits were demonstrated by Maunuksela and co-workers following inpatient surgery in a cohort of pediatric patients (43). Children who received rectal ibuprofen (40 mg/kg/day) had lower pain scores; decreased opioid requirements in the recovery room, during the day of operation, and 72 hours following the procedure; and a decreased incidence of opioid-related adverse effects. Although rectal preparations of ibuprofen are not available in the United States, similar results have been reported in both children and adults for the use of rectal indomethacin or acetaminophen (44).
When oral or rectal administration is not feasible, IV administration using the parenteral NSAID ketorolac should be considered. Although initial clinical trails suggested that ketorolac was as effective as opioids in treating acute pain, its practical clinical role is similar that of other NSAIDs as an adjunct to opioid analgesia, as demonstrated by Vetter and Heiner (45). A single IV dose of ketorolac was administered just prior to the completion of a surgical procedure as a supplement to morphine patient-controlled analgesia (PCA). Patients receiving ketorolac had decreased morphine requirements, lower pain scores, and a decreased incidence of adverse effects during the initial 12 postoperative hours.
Dsida and colleagues evaluated the pharmacokinetics of a single dose of ketorolac (0.5 mg/kg) in 36 children, stratified into four age groups (1–3 years, 4–7 years, 8–11 years, and 12–16 years) (46). No differences in pharmacokinetics were noted among the various age groups. The authors concluded that in patients ranging in age from 1 to 16 years, the pharmacokinetic properties of ketorolac were similar to those reported in adults, and a plasma concentration in the adult therapeutic range can be maintained for 6 hours in the majority of patients. Although Dsida and colleagues recommended a dosing regimen of 0.5 mg/kg every 6 hours, recent information from the adult literature has suggested the need to reevaluate these practices. In the adult population, a ceiling effect upon opioid sparing was noted following lumbar spine surgery for doses greater than 7.5 mg (47).
To date, there are limited data regarding ketorolac use in patients younger than 1 year of age (48,49). In a retrospective analysis of ketorolac use in infants younger than 6 months of age, daily morphine requirements (0.04 ± 0.05 versus 0.15 ± 0.06 mg/kg/day, p <0.01) were significantly decreased in 10 infants who received ketorolac (1–1.5 mg/kg/day for up to 48 hours) when compared with eight control patients who did not receive ketorolac (48). No difference in pain scores were noted between the two groups, and no adverse effects related to ketorolac were noted. Moffett and colleagues reported no adverse renal or hematologic effects in their retrospective review of ketorolac use in 53 children younger than 6 months
of age who received at least one dose of ketorolac following surgery for congenital heart disease (49). The greatest increase in serum creatinine from baseline was 0.3 mg/dL. Four patients had minor episodes of bleeding while receiving ketorolac.
of age who received at least one dose of ketorolac following surgery for congenital heart disease (49). The greatest increase in serum creatinine from baseline was 0.3 mg/dL. Four patients had minor episodes of bleeding while receiving ketorolac.
A major issue with any of the NSAIDs is their effect on platelet function and the potential for bleeding, particularly given ketorolac’s relative selectivity in inhibiting COX-1. These concerns are illustrated by the study of Rusy and co-workers, who randomized 50 children undergoing tonsilloadenoidectomy to receive either ketorolac (1 mg/kg) or acetaminophen (35 mg/kg rectally) following anesthetic induction. Patients receiving ketorolac had significantly more blood loss (2.67 versus 1.44 mL/kg) and required more surgical interventions to control bleeding. Despite the concerns illustrated by the study of Rusy and co-workers, increased bleeding with the use of ketorolac has not been demonstrated in two other types of potentially high-blood loss procedures including surgery for congenital heart surgery and spinal fusion (50,51,52,53). In a retrospective analysis, there was no difference in the need for surgical reexploration in infants and children following surgery for congenital heart disease who received ketorolac compared to case-matched controls (50). In a prospective, randomized trial in the same population, there was no difference in median chest tube output (13.3 versus 16.5 mL/kg/day) when comparing patients randomized to ketorolac or placebo (53).
The adverse effects of NSAIDs and acetylsalicylic acid result from the inhibition of homeostatic prostaglandins that regulate normal physiologic processes, including renal blood flow and the protection of the gastric mucosa. Alterations in glomerular filtration and the development of renal insufficiency or failure is uncommon except in patients with preexisting renal dysfunction, during concomitant administration of other nephrotoxic agents, in the presence of hypovolemia, or with prolonged administration. A review of the short-term use (48 hours) of IV ketorolac in over 1,700 children at Children’s Hospital Boston demonstrated a low rate of complications. Four children (0.2%) demonstrated hypersensitivity reactions (urticaria and/or bronchospasm), two children (0.1%) had evidence of renal impairment (although both had other underlying problems that could account for the renal insufficiency) and one child (0.05%) had melena at the completion of a 48-hour course of the drug (54).
A concern with ketorolac or other NSAIDs is the potential for inhibition of new bone formation. In an adult population, a retrospective review of 288 patients revealed the incidence of nonunion to be five of 121 in patients who received no NSAIDs compared to 29 of 167 in patients receiving ketorolac following lumbar spinal fusion (55). Therefore, some health care providers avoid the use of ketorolac in patients undergoing spinal fusion and other procedures in which bone grafts are used. An anecdotal report also describes the temporal association of the intraoperative administration of ketorolac with the development of bradycardia in two children whose heart rates decreased from 98 to 48 beats/minute and from 138 to 32 beats/minute (56). Although no physiologic explanation was provided in this report, the authors cautioned against the use of ketorolac without electrocardiogram (EKG) monitoring and suggested premedication with an anticholinergic agent.
Efforts to provide analgesia while diminishing the incidence of adverse effects of NSAIDs have focused on the use of specific isomers of the NSAIDs or agents that selectively inhibit COX-2 versus COX-1. Although NSAID isomers are still in the investigational phase, experience with other medications has demonstrated the potential for decreasing adverse effects while maintaining efficacy by separation of the two enantiomers of a chiral compound. Ibuprofen is a chiral mixture of its two optical isomers and animal data suggest that the S(+) isomer may provide analgesia while having limited effects on the homeostatic COX, thereby limiting its adverse effect profile (57). COX-1, referred to as the homeostatic COX, controls renal blood flow, protects the gastric mucosa, and maintains normal platelet function. COX-2, referred to as inducible COX, is responsible for the inflammatory process. Celecoxib and rofecoxib were the first selective COX-2 inhibitors introduced into clinical use. Like nonspecific NSAIDs and acetaminophen, when used as an adjunct to opioid analgesia, they decrease opioid requirements and opioid-related adverse effects (58). However, the enthusiasm for these agents and their clinical use has decreased dramatically following several investigations demonstrating their association with an increased risk of cardiovascular events including myocardial infarctions (59,60,61). Currently, celecoxib is the only COX-2 inhibitor marketed in the United States, and it is only available in tablet form, limiting its use to older children.
In the inpatient setting, management strategies to control severe pain generally include the use of the strong opioids. The clinically relevant differences between the opioids are their potency, duration of action, and their metabolic fate, including the presence or absence of active metabolites (Table 47-4). Opioids interact with specific opioid receptors (μ or κ) in the PNS and CNS. They may act as either pure agonists (binding to and activating μ and κ receptors) or agonist/antagonists (binding to and activating κ receptors while binding to, but not activating, or even antagonizing, μ receptors). The agonist/antagonists in common clinical use include nalbuphine, butorphanol, and pentazocine (Table 47-4). The major caveat with the use of these agents in patients of any age is that they should not be administered to patients chronically receiving opioids, as their μ-antagonism can precipitate withdrawal. One of the cited potential clinical advantages of these agents is a decreased potential to cause respiratory depression. Given this property, these agents may be chosen to provide analgesia in patients with comorbid disease processes that place them at risk for opioid-related adverse effects. The potency and efficacy of agonists/antagonists for severe pain is less than that of pure agonists, and a ceiling dose exists for their analgesic effects, above which dose escalations do not augment analgesia. Given these restrictions, they may also be useful for mild to moderate pain when oral administration of other agents, such
as acetaminophen with codeine, is not feasible or when the IV route is preferred to provide a more rapid onset of action. An additional benefit of a drug such as nalbuphine is that it clinically provides more sedation than other opioids because of its effects on the κ opioid receptor. Therefore, in addition to providing analgesia, it may also provide sedation for the agitated postoperative patient. Nalbuphine has also been shown to be effective in the treatment of emergence agitation following inhalational anesthesia in children (62). The agonist/antagonists should also be considered when supplemental IV analgesia is required in patients who are receiving or have received neuraxial (epidural or intrathecal) opioids within the last 24 hours. There may be a diminished risk of respiratory depression if an agonist/antagonist is used intravenously to supplement analgesia after neuraxial morphine rather than a pure agonist such as morphine. Additionally, these agents may effectively control adverse effects such as pruritus following neuraxial opioids.
as acetaminophen with codeine, is not feasible or when the IV route is preferred to provide a more rapid onset of action. An additional benefit of a drug such as nalbuphine is that it clinically provides more sedation than other opioids because of its effects on the κ opioid receptor. Therefore, in addition to providing analgesia, it may also provide sedation for the agitated postoperative patient. Nalbuphine has also been shown to be effective in the treatment of emergence agitation following inhalational anesthesia in children (62). The agonist/antagonists should also be considered when supplemental IV analgesia is required in patients who are receiving or have received neuraxial (epidural or intrathecal) opioids within the last 24 hours. There may be a diminished risk of respiratory depression if an agonist/antagonist is used intravenously to supplement analgesia after neuraxial morphine rather than a pure agonist such as morphine. Additionally, these agents may effectively control adverse effects such as pruritus following neuraxial opioids.
Table 47-4 Potency and half-life of Parenteral opioids | ||||||||||||||||||||||||||||||||||||||||||||||||||||||||
---|---|---|---|---|---|---|---|---|---|---|---|---|---|---|---|---|---|---|---|---|---|---|---|---|---|---|---|---|---|---|---|---|---|---|---|---|---|---|---|---|---|---|---|---|---|---|---|---|---|---|---|---|---|---|---|---|
|
When potent opioids are chosen for postoperative analgesia, three choices must be made: the type of opioid, the mode of administration, and the route of administration. There is limited data concerning the optimal opioid for postoperative analgesia in the pediatric patient. Several acceptable alternatives are available, any of which will provide equivalent analgesia provided that equipotent doses are administered. In the critically ill ICU patient, in the presence of comorbid diseases such as a compromised cardiovascular status or when there is a risk for pulmonary hypertension, the synthetic phenylpiperidine opioids (fentanyl, sufentanil, alfentanil, remifentanil) offer cardiovascular stability, the ability to modulate pulmonary vascular resistance, and the ability to blunt the sympathetic stress response. As the synthetic opioids have short plasma half-lives (<30 minutes), they need to be administered by a continuous infusion to maintain a plasma concentration adequate to provide analgesia. Anecdotal experience has also described the use of fentanyl-PCA in the pediatric patient (63,64). However, this practice remains the exception rather than the rule and is indicated only in selected clinical scenarios when other opioids fail or are associated with debilitating adverse effects.
There seems to be no inherent advantage to one synthetic opioid over the others except that fentanyl is the least expensive. Remifentanil, metabolized by plasma esterases, has the shortest half-life of any of the synthetic opioids (approximately 10 minutes) and, unlike the other opioids that undergo hepatic metabolism, there is no difference in its half-life across age ranges beginning with neonates. Kinder-Ross and colleagues evaluated the pharmacokinetics of remifentanil (bolus dose of 5 μg/kg) in 42 pediatric patients undergoing elective surgical procedures (65). The patients were divided into six groups according to age, including young infants (younger than 2 months), older infants (2 months to 2 years), young children (2–7 years), older children (7–13 years), adolescents (13–16 years), and young adults (16–18 years). The volume of distribution was largest in infants who were 2 months of age or younger (452 mL/kg) and decreased to 223 and 308 mL/kg in the two oldest groups of patients. There was a more rapid clearance in the infants younger than 2 months of age (90 mL/kg/min) and infants 2 months to 2 years of age (92 mL/kg/min) than in the older groups (46 to 76 mL/kg/min). The authors concluded that remifentanil, as in adults, is eliminated extremely rapidly in all pediatric age groups. The fast clearance rates observed in neonates and infants, as well as the lack of age-related changes in half-life, are in sharp contrast to the pharmacokinetic profile of other opioids. Given these properties, it has become a popular agent for intraoperative use. Unlike the other synthetic opioids, remifentanil does not demonstrate changes in its context-sensitive half-life, so that prolonged infusions do not result in increases in the plasma half-life (66). Given this property, it may be used to transition from other sedatives/analgesics prior to extubation to allow for elimination of other medications or to allow for periods of deep sedation/analgesia during mechanical ventilation in the pediatric ICU while providing a rapid wake-up following its discontinuation (67).
Aside from its cost, an issue with remifentanil that may preclude its long-term use in the ICU setting is the rapid development of tolerance, an effect attributed to the avidity with which it binds to the μ opioid receptor. The potential impact of this effect can be illustrated by the study of Crawford and co-workers, who randomized adolescents (12–17 years of age) undergoing posterior spinal fusion to an anesthetic regimen that included either intermittent doses of morphine or a remifentanil infusion (68). Postoperative pain scores were similar between the two groups, but patients given remifentanil required 30% more morphine during the initial 24 postoperative hours (1.65 ± 0.41 versus 1.27 ± 0.32 mg/kg, p <0.001).
Although the synthetic opioids maintain stable hemodynamics and are frequently used in patients with compromised cardiovascular function, alternative agents such as morphine are acceptable to treat most other types of acute severe pain in the pediatric population. In a cohort of 44 children following surgery for congenital heart disease, Lynn and co-workers demonstrated that morphine in doses of 10 to 30 μg/kg/hr provided effective analgesia without impeding weaning from mechanical ventilation (69). This morphine infusion resulted in plasma concentrations of less than 30 ng/mL and was not associated with elevated PaCO2. Five extubated patients breathed spontaneously and 35 patients were weaned from assisted to spontaneous ventilation with normal PaCO2 values while receiving morphine. In the 12 patients who were old enough to provide verbal pain scores, pain was relieved when serum morphine levels exceeded 12 ng/mL. The same group of investigators have shown that there is no difference in morphine clearance or its effects on ventilation when comparing infants with cyanotic and acyanotic congenital heart disease (70). However, they did note significant interpatient variability (two- to threefold) in morphine clearance in infants of similar ages.
In addition to its efficacy, outcome studies of patients treated with morphine during the neonatal period have not demonstrated adverse effects on neurocognitive development (71). MacGregor and colleagues assessed 87 children who had been enrolled into neonatal sedation studies. The authors reported no effect from exposure to morphine given in the neonatal period to facilitate mechanical ventilation upon measures of intelligence, motor function, or behavior when these children are assessed at 5 to 6 years of age. When compared with fentanyl, morphine offers the advantage of the less rapid development of tolerance and less withdrawal issues following prolonged administration. Franck and co-workers reported that infants sedated with morphine during extracorporeal membrane oxygenation (ECMO) required fewer supplemental doses, had a lower incidence of withdrawal (1 of 11 versus 13 of 27, p <0.01), and had a shorter hospital stay (31.1 ± 14 versus 21.5 ± 7.0 days, p = 0.01) when compared to patients receiving fentanyl (72). Given these findings and the vast clinical experience with its use, morphine is generally the opioid of choice for the treatment of acute severe pain. As with any medication that is dependent on hepatic metabolism, the pharmacokinetics of morphine are significantly different in neonates and infants compared to older children and adults. Additionally, significant interpatient variability occurs when comparing patients of comparable gestational and chronological ages (69,70). Lynn and Slattery provided preliminary data regarding morphine
pharmacokinetics in 10 infants born at a gestational age of 36 to 41 weeks who were less than 10 weeks of age (73). Infants younger than 10 days of age showed longer morphine elimination half lives than older infants (6.8 versus 3.9 hours), and morphine clearance in these younger infants was less than one-half that found in older infants (6.3 versus 23.8 mL/min/kg). The authors also suggested that the half-life of morphine approaches adult values at about 1 month of age, although they cautioned that this conclusion was based on findings in only 3 infants. Due to decreased hepatic metabolism and a suggestion that differences in the permeability of the blood–brain barrier in infants may lead to an increased risk of respiratory depression (74), dosing for the control of acute pain is started at 50% of the usual doses for infants younger than 3 months of age (Table 47-5). Furthermore, monitoring of respiratory function with continuous pulse oximetry is recommended when opioids are used in this age group or in patients with compromised cardiorespiratory status. Morphine’s predominant hemodynamic effect is peripheral vasodilatation, which may decrease preload and result in hypotension. Clinically significant hemodynamic effects are uncommon in patients with normal cardiovascular function, but may be accentuated with comorbid cardiac disease or in the setting of hypovolemia. Preterm neonates may be especially susceptible to these effects. A recent review of a large group of ventilated preterm infants (n = 898) who received morphine boluses and infusions as preemptive treatment in the neonatal ICU found that morphine can cause significant hypotension in preterm neonates between 23 and 26 weeks of gestational age (75). The authors of this study suggest close monitoring and cautious use in these particularly vulnerable infants.
pharmacokinetics in 10 infants born at a gestational age of 36 to 41 weeks who were less than 10 weeks of age (73). Infants younger than 10 days of age showed longer morphine elimination half lives than older infants (6.8 versus 3.9 hours), and morphine clearance in these younger infants was less than one-half that found in older infants (6.3 versus 23.8 mL/min/kg). The authors also suggested that the half-life of morphine approaches adult values at about 1 month of age, although they cautioned that this conclusion was based on findings in only 3 infants. Due to decreased hepatic metabolism and a suggestion that differences in the permeability of the blood–brain barrier in infants may lead to an increased risk of respiratory depression (74), dosing for the control of acute pain is started at 50% of the usual doses for infants younger than 3 months of age (Table 47-5). Furthermore, monitoring of respiratory function with continuous pulse oximetry is recommended when opioids are used in this age group or in patients with compromised cardiorespiratory status. Morphine’s predominant hemodynamic effect is peripheral vasodilatation, which may decrease preload and result in hypotension. Clinically significant hemodynamic effects are uncommon in patients with normal cardiovascular function, but may be accentuated with comorbid cardiac disease or in the setting of hypovolemia. Preterm neonates may be especially susceptible to these effects. A recent review of a large group of ventilated preterm infants (n = 898) who received morphine boluses and infusions as preemptive treatment in the neonatal ICU found that morphine can cause significant hypotension in preterm neonates between 23 and 26 weeks of gestational age (75). The authors of this study suggest close monitoring and cautious use in these particularly vulnerable infants.
Table 47-5 Morphine dosing guidelines* | ||
---|---|---|
|
Histamine release from morphine may lead to pruritus, an adverse effect that tends to be particularly common in adolescents and young adults or patients with skin symptoms from other disease processes. Morphine is metabolized in the liver to morphine-6-glucuronide (M6G), which is significantly more potent that the parent compound. As it is water soluble, M6G penetrates the CNS poorly and, in most circumstances, is of little consequence. However, M6G is cleared by the kidneys and can accumulate in patients with renal failure or insufficiency and lead to respiratory depression. In such patients, alternative opioids such as hydromorphone, which has no comparably active metabolites, should be considered.
Other opioids used in the treatment of acute pain include hydromorphone (Dilaudid), meperidine (Demerol), and methadone (Dolobid). Hydromorphone may be advantageous when adverse effects related to histamine release, such as pruritus, occur with morphine. Hydromorphone’s potency is five to eight times that of morphine and therefore one-fifth to one-eighth of the morphine dose is used. Pediatric PCA solutions can be prepared so that an equipotent amount of the opioid is present in each milliliter of the solution (1 mg/mL for morphine, 1 mg/mL for nalbuphine, 0.15 mg/mL for hydromorphone, and 10 mg/mL for meperidine).
Meperidine (pethidine in Europe and the United Kingdom) is approximately one-tenth as potent as morphine. It is associated with a relatively high incidence of adverse CNS effects, including dysphoria, agitation, and seizures. Meperidine’s CNS toxicity, including its epileptogenic potential, results from the accumulation of normeperidine, a metabolite produced by the hepatic N-methylation of the parent compound. Normeperidine has a long half-life (15–20 hours) and is dependent on renal clearance. High or toxic levels occur more commonly in the setting of renal failure/insufficiency, with the coadministration of drugs such as phenobarbital that stimulate hepatic microsomal enzymes, and with large doses (greater than 2 g/day in an adult). Since meperidine offers no particular advantage over other opioids and, in fact, may be less efficacious than morphine in controlling acute pain (76), its use is discouraged in favor of morphine or hydromorphone.
Methadone has a potency similar to that of morphine; however, its serum half-life is 12 to 24 hours, thereby providing a prolonged therapeutic serum concentration following a single bolus dose. This effect can be used to provide prolonged postoperative analgesia without the need for a continuous infusion or the use of a PCA device. Intraoperatively, a single IV methadone dose of 0.2 mg/kg resulted in lower pain scores and a decreased need for supplemental opioid analgesic agents during the initial 36 postoperative hours (77). Although effective, the use of IV methadone in the acute pain setting remains investigational and is therefore limited to institutions involved in ongoing clinical trials or in the hands of investigators with significant experience with its use. The IV preparation may not be readily available in many institutions.
Once the specific drug is selected, the second decision to be made regarding opioid analgesia for infants and children is the mode of administration. Options include on demand (“as needed” or “p.r.n.” dosing), fixed interval administration, continuous infusion, or nurse-controlled analgesia (NCA). To provide optimal analgesia, opioids should be administered in a manner that maintains a steady-state serum concentration. For moderate to severe pain, “on demand” administration generally does not provide adequate analgesia, as a significant delay can occur from the time that it is recognized that the child is in pain until the medication is drawn up, administered, and has time to take effect. Patient-controlled analgesia minimizes such delays by allowing the patient to administer a pre-set amount of opioid at specific intervals. These devices may be used in children as young as 5 or 6 years of age (78).
Appropriate education for the patient, family, nurse, pharmacist and the entire care team is required prior to instituting a pediatric PCA program to minimize potential adverse effects and ineffective use of this technique. Decisions regarding PCA include the opioid to be used, the bolus dose, the lockout interval (the minimum time that must elapse between doses), whether a continuous or basal infusion will be used in
addition to the intermittent bolus doses, and the maximum total hourly dose. Although any opioid can be used with PCA, the majority of experience is with morphine. A common starting pointing for the bolus dose is 20 μg/kg every 7–10 minutes as needed. Dose adjustments may be needed based on the patient’s previous exposure to opioids, comorbid disease processes, and interpatient variability. Prior to starting PCA for acute pain, titration of opioid to achieve an effective plasma concentration to provide analgesia must be achieved. To accomplish this, sequential bolus doses of morphine (20 μg/kg every 5–10 minutes) are titrated until the desired level of analgesia is obtained. Once this is accomplished, the PCA device is started. A frequent problem is that patients are provided adequate analgesia in the recovery room, the emergency department, or the inpatient ward, followed by a delay until the PCA pump arrives, is programmed, and hooked up. By that time, patients may need to repeatedly push the PCA button to reestablish an analgesic plasma concentration of the opioid.
addition to the intermittent bolus doses, and the maximum total hourly dose. Although any opioid can be used with PCA, the majority of experience is with morphine. A common starting pointing for the bolus dose is 20 μg/kg every 7–10 minutes as needed. Dose adjustments may be needed based on the patient’s previous exposure to opioids, comorbid disease processes, and interpatient variability. Prior to starting PCA for acute pain, titration of opioid to achieve an effective plasma concentration to provide analgesia must be achieved. To accomplish this, sequential bolus doses of morphine (20 μg/kg every 5–10 minutes) are titrated until the desired level of analgesia is obtained. Once this is accomplished, the PCA device is started. A frequent problem is that patients are provided adequate analgesia in the recovery room, the emergency department, or the inpatient ward, followed by a delay until the PCA pump arrives, is programmed, and hooked up. By that time, patients may need to repeatedly push the PCA button to reestablish an analgesic plasma concentration of the opioid.
One controversial issue regarding PCA is whether to include a low basal infusion rate in addition to patient-administered bolus doses. The use of a basal infusion rate is thought by some to oppose the inherent safety factor of PCA that patients who are somnolent cannot push the button and administer additional opioid. With a basal infusion rate, opioid is infused regardless of the patient’s demands. Different outcomes have been reported in pediatric patients depending on the dose used for the basal infusion rate. Doyle and co-workers compared PCA (morphine 20 μg/kg every 5 minutes as needed) with and without a basal infusion rate of 20 μg/kg/hour (79). Although there was no difference in the pain scores, there were more adverse effects including nausea, sedation, and hypoxemia in the patients who received the basal infusion. In a follow-up study, the same investigators compared three different regimens of PCA that included morphine 20 μg/kg every 5 minutes as needed with no basal infusion rate (group 1), a basal infusion of 10 μg/kg/hour (group 2), or a basal infusion rate of 4 μg/kg/hour (group 3) (80). Although pain scores were equivalent in all three groups, there was increased time spent asleep during the first two postoperative nights in groups 2 and 3 with no difference in time asleep during the day. There was an increased incidence of nausea and vomiting in the group 2. The authors concluded that a low basal infusion of 4 μg/kg/hour improved the sleep pattern when compared with no basal infusion.
When used in classic fashion, PCA requires an awake, cooperative patient who is able to comprehend its purpose and push the button to initiate delivery of the medication. Therefore, its use may be limited in certain patient populations due to age, underlying illness, or cognitive capabilities. A second controversy regarding PCA is whether to allow the use of nurse and/or family controlled PCA (PCA by proxy) in these patient populations. When used in this fashion, the PCA device eliminates the delay in opioid administration that may occur with traditional as-needed dosing when the nurse signs out the medication and draws it up. Murphy and colleagues demonstrated equivalent levels of analgesia and equivalent opioid consumption when comparing PCA with NCA in an adult population (81). They noted no difference in the incidence of adverse effects or level of analgesia achieved in their study cohort. Recent studies in infants and children have established NCA, provided according to a standardized protocol, to be a generally safe and effective alternative to intermittent dosing or continuous infusions of opioids. Closer monitoring of respiratory status (i.e., cardiorespiratory monitoring and/or pulse oximetry) is essential during NCA, since younger children remain at somewhat higher risk of respiratory depression whenever opioids are administered.
Monitto and colleagues recently reviewed their experience with NCA in 212 infants and children from newborn to 6 years of age (82). They noted a 1.7% incidence of respiratory depression requiring treatment with naloxone and a need for supplemental oxygen in 25% of patients to maintain oxygen saturation greater than 95% in the first 24 hours after surgery. This incidence of respiratory depression is significantly higher than the 0.2% to 0.7% reported for PCA in adults. As with other studies of opioids in children, the youngest children appeared to be at the highest risk. All of the episodes of respiratory depression occurred in children 3 years of age or younger, and most (56%) were in infants under 1 year of age. This increased risk in younger patients during NCA was also found in the review of Lloyd-Thomas and Howard (83). They reported a 1% incidence of respiratory depression in pediatric patients receiving NCA and no significant respiratory depression in the older children who self-administered their own doses. Although practices vary, many pediatric institutions currently use NCA in patients who are unable to activate the device, but for safety purposes most do not permit family members to activate the PCA device for acute postoperative pain.
The Nonintravenous Administration of Opioids for Acute Pain
In the majority of clinical scenarios, moderate to severe acute pain is treated with IV opioids. However, certain situations may arise in which venous access is limited, drug incompatibilities are present, or other factors preclude the use of the IV route. In these situations, non-IV routes of administration may become necessary to ensure adequate analgesia. Non-IV routes employed to deliver opioids include subcutaneous, oral, transdermal, and transmucosal (sublingual, buccal, intranasal, rectal, inhaled) administration (84). Some of these techniques are considered investigational and therefore likely to have a limited role in the day-to-day management of acute pain in children. The intramuscular route is strictly avoided as variability in uptake and absorption leads to erratic serum levels and ineffective analgesia. Additionally, children will deny pain to avoid a “shot.”
The simplest and cheapest of the non-IV routes remains oral administration. Although this route is frequently chosen for outpatients, its use remains limited in hospitalized patients. Oral administration of the “weak opioids,” including codeine and oxycodone has been previously discussed in this chapter. This technique is a viable option even in hospitalized patients, provided their pain is mild to moderate, or when transitioning from IV opioids or regional anesthetic techniques. When compared with IV administration, issues with oral administration include a delay in onset of action, the need for larger doses due to decreased bioavailability and first-pass hepatic metabolism, and underlying medical/surgical problems that preclude the use of the gastrointestinal tract. Litman and Shapiro reported their experience with a novel technique that they termed “oral PCA” using either hydromorphone or morphine to treat acute pain related to medical illnesses in a cohort of four pediatric patients (85). When no IV access is available, oral methadone can also be used for acute postoperative pain. Methadone is available in an elixir form and has excellent bioavailability via the oral route, ranging from 60 to 90% (86). A sliding scale regimen has been used at Children’s Hospital, Boston for many years utilizing “reverse p.r.n.” dosing. Patients are assessed by the nursing staff at intervals no less frequent than every 4 hours. A “sliding scale” adjusts the methadone dose according to the nurse’s
assessment of the child’s pain as severe (0.07 mg/kg), moderate (0.05 mg/kg), or mild (0.03 mg/kg). This method is convenient, inexpensive, and permits easy adjustment of dosing to patients’ variable requirements. Subcutaneous administration has generally been reserved for the terminal cancer patient. However, preliminary experiences outside of the cancer population suggest its efficacy for controlling acute pain of various etiologies. Doyle and colleagues investigated the efficacy of PCA by the subcutaneous route in children following appendectomy (87). Patients were randomized to either IV or subcutaneous administration with a standard PCA regimen that included morphine (bolus doses of 20 μg/kg every 5 minutes as needed, plus a basal infusion of 5 μg/kg/hour). The subcutaneous PCA was delivered through a 22-gauge IV cannula that was placed into the subcutaneous tissue over the deltoid muscle during the operative procedure. There was no difference in the pain scores at rest or with activity between the two groups. There were significantly more hypoxemic events, defined as an oxygen saturation less than 90%, with IV compared with subcutaneous administration. Additional experience with use of subcutaneous opioids for the management of acute pain in children was reported by Lamacraft and colleagues in a cohort of 220 pediatric patients (88). Morphine was administered via an indwelling catheter that was placed into the subcutaneous tissue after the induction of anesthesia. No patient complained of infusion site pain during subcutaneous administration of morphine. When compared with the intermittent intramuscular administration of opioids, 95% of the nurses preferred the subcutaneous route, and 74% of them stated that they would give morphine more readily via the subcutaneous route compared to the intramuscular route. Dietrich and co-workers reported similarly encouraging efficacy with the use subcutaneous fentanyl in a cohort of 24 children (89). Unlike the two previous reports, their cohort included a more heterogeneous population. Opioid administration was required for the gradual weaning of opioid administration following prolonged ICU care, for acute pain problems, and during terminal care. Reasons for using subcutaneous administration included either lack of venous access or drug incompatibilities that precluded IV administration.
assessment of the child’s pain as severe (0.07 mg/kg), moderate (0.05 mg/kg), or mild (0.03 mg/kg). This method is convenient, inexpensive, and permits easy adjustment of dosing to patients’ variable requirements. Subcutaneous administration has generally been reserved for the terminal cancer patient. However, preliminary experiences outside of the cancer population suggest its efficacy for controlling acute pain of various etiologies. Doyle and colleagues investigated the efficacy of PCA by the subcutaneous route in children following appendectomy (87). Patients were randomized to either IV or subcutaneous administration with a standard PCA regimen that included morphine (bolus doses of 20 μg/kg every 5 minutes as needed, plus a basal infusion of 5 μg/kg/hour). The subcutaneous PCA was delivered through a 22-gauge IV cannula that was placed into the subcutaneous tissue over the deltoid muscle during the operative procedure. There was no difference in the pain scores at rest or with activity between the two groups. There were significantly more hypoxemic events, defined as an oxygen saturation less than 90%, with IV compared with subcutaneous administration. Additional experience with use of subcutaneous opioids for the management of acute pain in children was reported by Lamacraft and colleagues in a cohort of 220 pediatric patients (88). Morphine was administered via an indwelling catheter that was placed into the subcutaneous tissue after the induction of anesthesia. No patient complained of infusion site pain during subcutaneous administration of morphine. When compared with the intermittent intramuscular administration of opioids, 95% of the nurses preferred the subcutaneous route, and 74% of them stated that they would give morphine more readily via the subcutaneous route compared to the intramuscular route. Dietrich and co-workers reported similarly encouraging efficacy with the use subcutaneous fentanyl in a cohort of 24 children (89). Unlike the two previous reports, their cohort included a more heterogeneous population. Opioid administration was required for the gradual weaning of opioid administration following prolonged ICU care, for acute pain problems, and during terminal care. Reasons for using subcutaneous administration included either lack of venous access or drug incompatibilities that precluded IV administration.
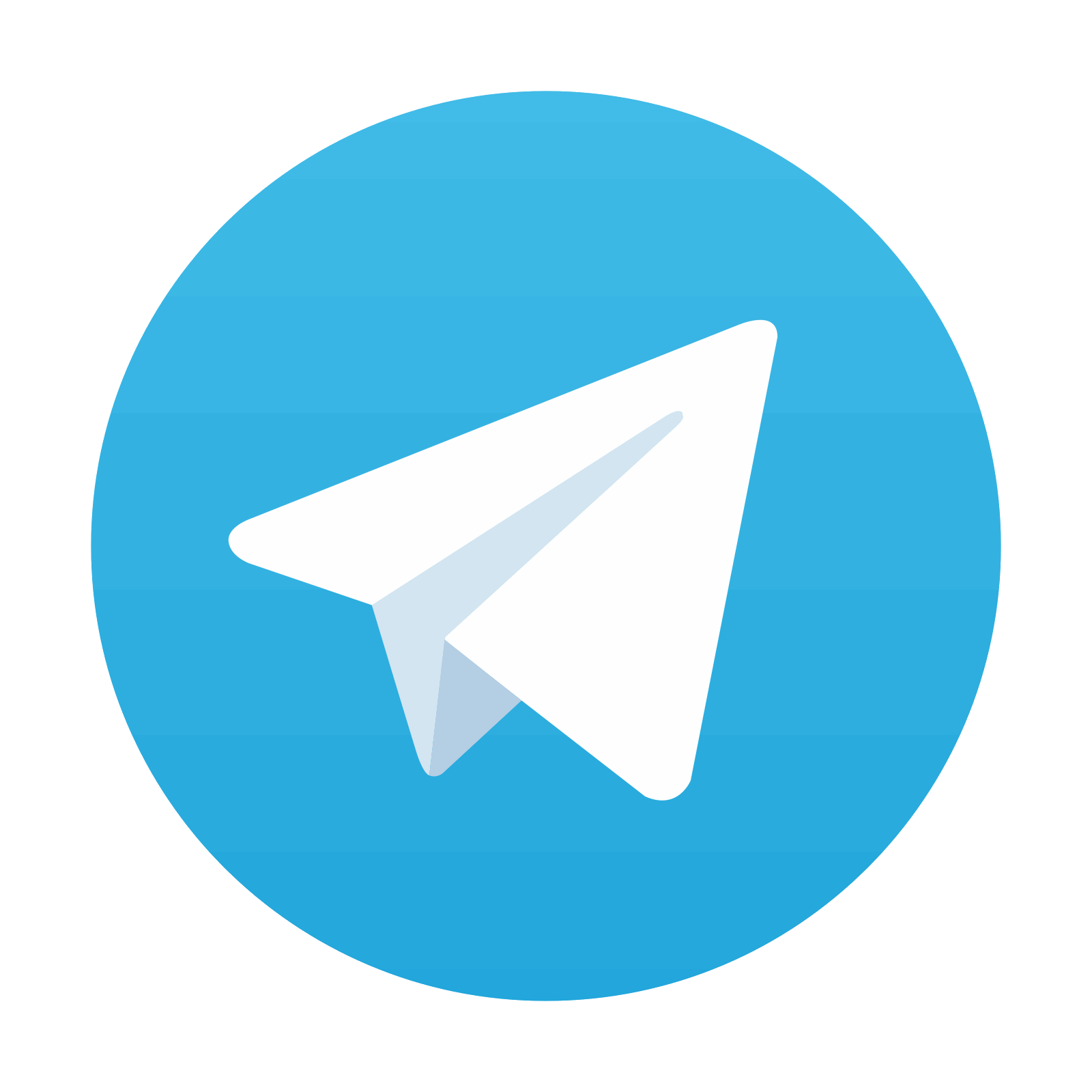
Stay updated, free articles. Join our Telegram channel

Full access? Get Clinical Tree
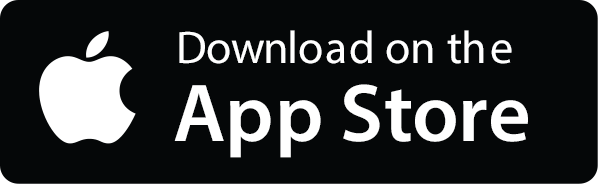
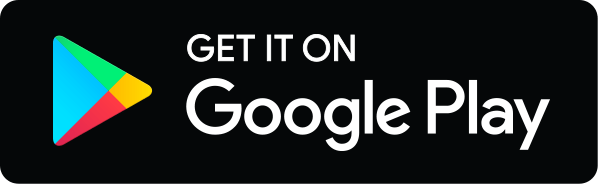