KEY POINTS
Advancements in immunosuppression, transplant techniques, antimicrobials, postoperative management and support, bridging techniques, and extracorporeal life support have had an enormous impact on morbidity and mortality of transplant recipients over the past few decades.
Although some generalizations can be made regarding the management of transplant patients, organ-specific considerations based on the particular allograft transplanted are critically important.
Infections can reactivate in an immunocompromised recipient who has been previously exposed. Alternatively, a naïve recipient may acquire an infection following the transplant of an organ from a seropositive donor. Infections in transplant recipients can progress rapidly and hence must be promptly recognized and appropriately treated.
Risks and benefits of sustained immunosuppressive therapy must be balanced in transplant recipients. Though immunosuppressive drugs are essential to prevent allograft rejection, they also increase the risk of infection and neoplasm.
Immunosuppressive drugs have significant side effects and many have important drug-drug interactions that must be recognized by the intensivist.
INTRODUCTION
Organ transplantation has become a cornerstone in the management of end-stage organ dysfunction. Since the 1960s, important scientific advances have greatly improved our understanding of transplant immunology. Innovations in transplant techniques have allowed for a remarkable change in survival of this population. Immunosuppressive and antimicrobial therapies have markedly decreased the incidence and severity of allograft rejection and overwhelming infection.
In the early decades of transplant, all forms of organ transplantation necessitated intensive postoperative monitoring; however, in recent years, some organ transplants (eg, kidney and pancreas) no longer require routine postoperative admission and monitoring in an intensive care unit. Lung, liver, and heart transplantation, however, remain challenging from a surgical and anesthesia perspective with risk for hemodynamic and respiratory complications intraoperatively and in the perioperative period. Their long-term outcome depends on the immediate postoperative management in the intensive care unit and recent advances in survival have been directly due to improvements in early postoperative care.
Given the burden of immunosuppression that these patients face throughout their posttransplant lives, they are at increased risk of developing severe standard and opportunistic infections prompting admission to an intensive care unit. Their lives are further complicated by acute and chronic rejection that could necessitate intensive care support. Knowledge of unique presentations of common illnesses or unique illnesses that present with common clinical syndromes is imperative for the early recognition and timely initiation of appropriate treatment. The consequences of missing rejection or infection include death, or graft failure and return of the patient back to a state characterized by the sequelae of end-organ dysfunction.
This chapter focuses on the indications, outcomes, postoperative management, and postoperative complications of lung, liver, and heart transplant. For the transplant intensivist, optimization of the postoperative care and knowledge of the potential complications are necessary to enhance outcome. For the nontransplant intensivist, knowledge of indications for transplant, supportive care, and optimization of function prior to transplant is important. An understanding of bridging techniques and having an approach to the management of immunosuppressed transplant patients and their unique presentations are imperative to optimizing their care and long-term outcome.
IMMUNOSUPPRESSION
To avoid rejection of the allograft by the recipient’s immune system, therapy with immunosuppressive agents is required. More than one agent is employed to allow for the targeting of different immunologic effector pathways (Fig. 115-1). Multiple advances in immunology have led to the creation of a variety immunosuppressive agents allowing for different combinations to achieve a desired level of immunosuppression. Therefore, each center has specific transplant protocols. However, as a consequence of the resultant modulation of the immune system’s surveillance and response functions, the transplant recipient is placed at risk of developing infections and neoplasms.
FIGURE 115-1
Sites of action of important immunosuppressive agents used to prevent allograft rejection in transplant recipients (see text for explanation). IL-1, interleukin-1; IL-2, interleukin-2; IL-2R Abs, anti–interleukin-2-receptor antibodies; LAK cells, lymphokine-activated killer cells; MMF, mycophenolate mofetil; NK cells, natural killer cells; PMNs, polymorphonuclear neutrophils.
Immunosuppressive therapy can be divided into three phases: induction, maintenance, and the treatment of acute rejection. The induction phase commences in the immediate postoperative period and involves intense immunosuppression that is usually continued for 2 to 4 weeks. Although the regimens used at different centers vary, most typically include the use of systemic corticosteroids in conjunction with a calcineurin inhibitor and a proliferation inhibitor (see below).1 These agents may be started intravenously, but are switched to oral formulations to minimize toxicity once the patient is tolerating and absorbing enteral medications and feeds. To avoid some of the renal toxicity associated with the calcineurin inhibitors, antithymocyte immunoglobulin or anti–interleukin-2-receptor antibodies (IL-2R Abs) may be substituted in the early postoperative period.
The maintenance phase follows the induction period and is heralded by a reduction in the intensity of immunosuppression. Typically the maintenance phase incorporates components of the planned regimen that will be used to suppress the immune response to the allograft and thus prevent rejection over a more prolonged period. Most patients will be maintained on either oral prednisone and cyclosporine or tacrolimus, although it appears that in some patients receiving tacrolimus for maintenance the steroid component may be discontinued completely.2 Azathioprine or mycophenolate mofetil is often used during the maintenance phase to permit dose reduction of the other immunosuppressive drugs in order to minimize side effects.
The remaining phase of immunosuppressive therapy is not planned but it is anticipated and involves the treatment of acute rejection. Despite improvements that have been made in immunosuppressive strategies, episodes of acute rejection still commonly occur in the postoperative period, typically between 1 week and 1 year following the operation. Early recognition and prompt treatment of acute rejection is essential if long-term graft function is to be preserved. Treatment involves an intensification of the immunosuppressive regimen, which is usually accomplished through the use of high-dose steroids, short courses of antithymocyte globulin, and increased doses of the other immunosuppressive therapies.
The introduction of cyclosporine was one of the most revolutionary events in the field of transplant medicine,3 and the drug remains an important component of immunosuppressive regimens used by many institutions. In recent decades, some centers have switched to tacrolimus, which has surpassed cyclosporine in certain centers as the agent of choice given evidence of greater efficacy in certain organ transplants. Sirolimus, a newer immunosuppressive, is generally not used as commonly given its side-effect profile. All three agents provide effective inhibition of T-cell activation by interfering with a receptor on T cells that involves a calcium-dependent signal transduction pathway.
Cyclosporine binds to intracellular cyclophilin, and this complex interacts with calcineurin. Calcineurin is crucial for normal lymphokine gene activation, and its inhibition by cyclosporine consequently interferes with the production of interleukins-2, -3, and -4 (IL-2, IL-3, and IL-4), tumor necrosis factor-α (TNF-α), and other important mediators of inflammation. In turn, specific and potent inhibition of T-cell activation (especially CD4 cells) is achieved. The most frequently encountered problem is nephrotoxicity, and in many patients cyclosporine causes a rise in serum creatinine level and a reduction of glomerular filtration rate (GFR). The mechanism of this toxicity is felt to be related to vasoconstriction of the afferent glomerular arteriole.3 Neurologic complications may be encountered in up to 20% of patients, and include tremor, paresthesias, headache, confusion, seizures, and even coma. Other complications include hypertension (which often requires treatment), hyperkalemia, tremor, hirsutism, gingival hyperplasia, and glucose intolerance. Cyclosporine is metabolized by cytochrome P450-3A4 (CYP450-3A4) enzymes. Therefore inhibitors of cytochrome P450 (eg, erythromycin, fluconazole, diltiazem, and verapamil) will increase serum cyclosporine levels, whereas inducers of these enzymes (eg, phenobarbital, phenytoin, rifampin, and trimethoprim) will decrease the serum levels. Previously, trough drug concentrations were used to guide therapy. However, more recent recommendations have focused on the use of peak cyclosporine levels to monitor the adequacy of immunosuppression.4 Ideally the dose of cyclosporine should be titrated using a biological assay or an assessment of the downstream effect of the drug on immune effector function. Such assays are under investigation.
Tacrolimus (formerly FK506) is a macrolide antibiotic with powerful immunosuppressive properties. Its mechanism of action is similar to cyclosporine, but with enhanced potency. Tacrolimus binds to a receptor known as FK-binding protein (FKBP), and suppresses calcineurin-dependent T-cell signaling.5 Two controlled trials comparing tacrolimus to cyclosporine in liver transplant recipients demonstrated that tacrolimus reduces the incidence of acute rejection, refractory rejection, and chronic rejection6,7 with similar patient and graft survival. However, the drug has important toxicities. As with cyclosporine, nephrotoxicity is the most troublesome side effect. Important neurotoxicity (seizures, tremor, psychoses, posterior reversible encephalopathy syndrome, and coma) may also occur with its use. Both tacrolimus and cyclosporine decrease insulin secretion and can lead to hyperglycemia.8,9 Interestingly, however, in renal transplant recipients who were converted from cyclosporine to tacrolimus, there was an improvement in other cardiovascular risk factors such as blood pressure and lipid profile.10 The gingival hyperplasia and hirsutism commonly seen with cyclosporine do not occur with tacrolimus. Tacrolimus is also metabolized by CYP450-2A4 enzymes, and the clinician must be cognizant of the effects other drugs will have on its metabolism.
Sirolimus (rapamycin) is a drug related to tacrolimus that binds to FKBP. However, in contrast to tacrolimus it does not interfere with calcineurin activity and consequently does not interfere with the activation of cytokine genes. Its main effects are the inhibition of IL-2 production and the suppression of T-cell proliferation. Side effects include thrombocytopenia, hypercholesterolemia, and hypertriglyceridemia. Compared to cyclosporine and tacrolimus, the drug does not appear to have the same potential to cause renal failure. However, sirolimus has been associated with an increased risk of hepatic artery thrombosis and subsequent allograft loss11 in liver transplant recipients. There have also been reports of interstitial pneumonitis associated with sirolimus.12-15 Sirolimus is not recommended in the early postoperative period following lung transplantation, as it has been associated with bronchial anastomotic dehiscence.16,17
The proliferation inhibitors block DNA and RNA synthesis by interfering with normal purine metabolism. Since lymphocytes are unable to salvage purine as efficiently as most other cells, these drugs effectively inhibit lymphocyte proliferation and clonal expansion of lymphocytes.
Azathioprine is a purine analogue that is converted in the liver to 6-mercaptopurine and compromises synthesis of DNA and RNA. Its most important side effect is marrow suppression, which may result in a profound leukopenia. Other important toxicities include hepatotoxicity and rarely fulminant hepatic failure, acute pancreatitis, skin malignancies, and increased susceptibility to infections.
Mycophenolate mofetil (MMF) interferes with purine metabolism as a reversible inhibitor of inosine monophosphate dehydrogenase. It also blocks clonal expansion of T lymphocytes. Important side effects of MMF include marrow depression (especially leukopenia and thrombocytopenia) and diarrhea. Less common problems include esophagitis, gastritis, and gastrointestinal bleeding. MMF may have an advantage over azathioprine in preventing acute rejection.18
Both azathioprine and MMF are now used predominantly as an adjunct to corticosteroid therapy in the maintenance phase of immunosuppression. The major advantage of these drugs is that their use will often enable the doses of corticosteroids and even of the calcineurin inhibitor to be reduced, thus avoiding some of the potential dose-related toxicity associated with these other agents.
Antibodies directed against lymphocytes were first introduced as an immunosuppressive therapy as antilymphocyte globulin (ALG). This therapeutic agent was created by immunizing animals with human lymphocytes and subsequently purifying the antibody-containing globulin fraction. ALG is very effective at depleting lymphocytes in the peripheral circulation, and quickly became an important component of regimens used for induction of immunosuppression and for the treatment of acute rejection. However, it was subsequently largely replaced by OKT3, a murine monoclonal preparation directed against T lymphocytes, which could be used in much smaller doses because of its increased purity. OKT3 binds to the CD3-ε chain present on all human T cells, and causes a rapid clearance of peripheral T cells. Interestingly, it initially causes activation of T cells and the subsequent release of several lymphokines, including IL-2, IL-6, γ-interferon, and TNF. Rabbit antithymocyte globulin and to a lesser extent equine antithymocyte globulin are currently the antilymphocyte antibody preparations used most frequently by most centers.1
The antilymphocyte preparations are used primarily in the induction phase, and they have been shown to have higher success rates than steroids for the treatment of acute rejection.19 However, these preparations have significant toxicity. Fever is almost always observed at the time of infusion. Nonetheless, the importance of careful surveillance for infectious complications cannot be understated, as these therapies significantly attenuate the normal host immune response. The inflammatory response due to lymphokine production that is often seen with the initiation of therapy usually responds to corticosteroids, antihistamines, and acetaminophen.20 Serum sickness has been observed with repeated use of these preparations. Furthermore, when these agents (especially OKT3) are administered more than once, the patient may develop antibodies directed against the globulin preparation that can substantially limit its efficacy. Long-term use is also associated with increased risk of viral infections (particularly cytomegalovirus) and posttransplant malignancy. Other less common complications of therapy include seizures (especially when concomitant hypocalcemia is present) and the acute respiratory distress syndrome (ARDS), possibly due to increased pulmonary capillary permeability arising from lymphokine stimulation.
Systemic corticosteroids remain a key component of each phase of immunosuppression, and are received by over 95% of patients with pancreas, heart, lung, and heart-lung transplants.1 They have multiple effects on the immune response. Importantly, steroids block T-cell proliferation and IL-2 synthesis. The phagocytic function of macrophages is inhibited, and in high doses, corticosteroids prevent neutrophil degranulation.
Though they effectively attenuate the immune response and help prevent allograft rejection, corticosteroids also significantly increase the recipient’s susceptibility to infection. In particular, viral and fungal infections appear to be associated with the use of corticosteroids. An important consideration is that steroids can also mask the typical signs of infection. For example, patients on high doses of these medications may not present with typical signs of peritonitis despite having intra-abdominal infections. As transplant patients typically remain on steroids chronically, the possibility of adrenal suppression and even overt adrenal insufficiency must be considered if these medications are discontinued. Despite their widespread use and important role in most immunosuppressive regimens, corticosteroids are associated with many of the significant side effects encountered by transplant patients. Insulin resistance is common, and the use of oral hypoglycemic agents and often insulin may be required. Both cyclosporine and tacrolimus affect insulin release and can induce insulin resistance, and may contribute to the hyperglycemia.21-24 Increased sodium and water retention induced by steroids may aggravate posttransplant hypertension. Impaired wound healing has been well documented with the use of corticosteroids, and consequently these drugs may contribute to the development of postoperative surgical complications. Steroids are associated with increased risk of gastrointestinal hemorrhage, and thus most patients will be placed on routine stress ulcer prophylaxis, usually with either a histamine (H2)-antagonist or a proton-pump inhibitor. Long-term use of steroids often leads to osteopenia, overt osteoporosis, and fractures. Aseptic necrosis is a dreaded complication of steroid use that can occur at any time following the institution of therapy. Skin changes and fat redistribution are common. Steroids are also associated with mood changes and rarely frank psychoses. Because of the significant side effects associated with steroid use, most maintenance immunosuppressive regimens now include antiproliferation inhibitors such as azathioprine or MMF to facilitate the early reduction of the corticosteroid dose.
Recently, there has been increased use of anti–interleukin-2 receptor antibodies (basiliximab, daclizumab) during the induction phase.1 Both agents bind to the α-chain of the IL-2 receptor which is only expressed on activated T lymphocytes, and competitively inhibit IL-2–induced proliferation of active T cells. The advantage of these compounds is that they are not associated with the toxicity produced by antilymphocyte preparations. Their use has been shown to decrease the rate of acute rejection following kidney transplant25,26 without any increase in infectious complications or malignancy. Success has also been seen when used in liver and heart transplantation.27
LUNG TRANSPLANTATION
Since the first successful lung transplant in 1983, lung transplantation has been established as a viable option for a wide range of end-stage lung disease, with increasing evidence that it can improve survival and quality of life across a range of lung conditions. The number of procedures reported annually by the International Society of Heart and Lung Transplant (ISHLT) has reached 2100.28 The fifth decade of the procedure celebrates significant advancements in the technique and in organ preservation, bridging patients prior to transplantation, minimizing complications immediately posttransplantation and optimizing immunosuppression and surveillance in the longer term. Despite these advances, early postoperative mortality remains high at 12%.29 In addition, these patients can present significant challenges to the intensivist. We are entering an era where previous limits are being revisited in order to provide this potential lifesaving technique to more patients. As a result, we are transplanting an older population and using methods to salvage grafts that would have previously been deemed unacceptable in order to accommodate the expanding recipient pool. These two extremes heighten the challenges that are inherent to the care of the lung transplant recipient. This section will provide an overview of the common critical care issues that face this unique patient population.
Indications: The ISHLT maintains the most complete database of lung transplant volumes and performance. The major indications for lung transplantation according to the ISHLT are listed in Table 115-1 and Figure 115-2. Chronic obstructive pulmonary disease (COPD) and interstitial pulmonary fibrosis (IPF) remain the two most common indications. Improvements in transplant techniques and medications have facilitated the transplantation of older individuals, hence the significant increase in proportion of transplants for IPF over the past two decades. Bilateral lung transplantation occurs in 62% and is a necessity for septic lung diseases such as cystic fibrosis as a single transplant would become contaminated by the numerous potential drug resistant organisms that reside in the native lung.30,31 In contrast, single lung transplant can be performed in patients with COPD and IPF. Both single lung and double lung transplant has been performed in patients with IPAH; however, survival has been shown to be superior in those who undergo double lung transplant in this population.32
Major Indications for Lung Transplantation (1995-2012)
Chronic obstructive pulmonary disease (COPD) | 34% |
Interstitial pulmonary fibrosis (IPF) | 24% |
Cystic fibrosis (CF) | 17% |
α-1-Antitrypsin deficiency emphysema (A1AD) | 6% |
Idiopathic pulmonary arterial hypertension | 3% |
Bronchiectasis | 3% |
Sarcoidosis | 2.5% |
Retransplant (obliterative bronchiolitis) | 1.5% |
Lymphangioleiomyomatosis | 1% |
Other | 8% |
FIGURE 115-2
Changing trends in lung transplant indication since 1990 (International Society for Heart and Lung Transplantation30).
Few data currently exist in the form of randomized control trials to support the current guidelines for lung transplantation. Decisions are therefore based on the consensus opinion of experts. The evaluation of potential recipients attempts to identify those with greater prospects of favorable outcomes given the scarcity of lungs available. Broadly, the indication for transplant is a patient who deteriorates despite optimal medical and surgical therapies. Table 115-2 outlines the various types of lung processes that could progress to end-stage disease and the guidelines for transplantation. Absolute contraindications include malignancy in the previous 2 years (with the exception of some cutaneous subtypes), untreatable and advanced dysfunction of another major organ system (liver, kidney, or heart if not amenable to percutaneous coronary intervention/bypass/transplant), noncurable extrapulmonary infections such as HIV and chronic active hepatitis B and C, significant chest wall and spinal deformities, documented nonadherence to medications, untreatable psychiatric conditions that could interfere with ability to comply with medical therapy, and substance addiction (alcohol, tobacco, narcotics) in the prior 6 months.
Guidelines for Lung Transplantation
Pulmonary Condition | Guidelines for Referral/Transplantation | Median Survival Without Transplant |
---|---|---|
COPD |
| 3 years (once disease refractory to medical/surgical management) |
IPF and nonspecific interstitial pneumonia |
| |
CF and bronchiectasis |
| 2-3 years (once disease refractory to medical management) |
IPAH |
| Less than 1 year when NYHA IV and refractory to therapy |
Sarcoidosis |
A Lung Allocation Score (LAS) created in 2005 in order to address the problems of high wait list mortality as well as unnecessary early placement on the wait list directs lung allocation in the United States. The score takes into consideration the expected number of days lived without transplant during an additional year on the wait list (transplant urgency), as well as the expected number of days lived in the first year posttransplant (transplant benefit). Transplant urgency is subtracted from benefit to give a score that is normalized on a scale from 0 to 100, which is the final LAS. The higher the score, the greater is the need for transplant. Since the implementation of the LAS, organ allocation has seen a shift to transplant based on urgency as opposed to wait time duration. As a result, the United States has seen a decrease in wait list times reflecting more appropriate placement and allocation of lungs.33,34 Wait list mortality has decreased for all indications except IPAH.35 No change in posttransplant survival has been seen compared to survival rates pre-LAS.34
Outcomes: Survival rates are 88% at 3 months, 79% at 1 year, 63% at 3 years, 52% at 5 years, and 29% at 10 years. More recent improvements in survival have been driven by improved management immediately posttransplant and in the first year.29 Three-month survival is highest for CF (90%) and COPD (91%) and lowest for IPF (85%) and IPAH (76%). These differences can be attributed to immediate complications postoperatively such as primary graft dysfunction (see “Postoperative Complications”). Long-term survival for those who survive at least 1 year changes in that CF, IPAH, and sarcoidosis have a greater 10-year survival (48%, 45%, and 41%, respectively) while COPD and IPF have the worst rates (28%, 30%, respectively) given that these patients are older and harbor more comorbid conditions (see Fig. 115-3).
FIGURE 115-3
Kaplan-Meier survival by diagnosis for adult lung transplants performed from January 1990 through June 2011. (Reproduced with permission of Christie JD, Edwards LB, Kucheryavaya AY, et al. The Registry of the International Society for Heart and Lung Transplantation: Twenty-seventh official adult lung and heart-lung transplant report—2010. J Heart Lung Transplant. 2010 Oct;29(10):1104-1118.)
Interestingly, long-term survival is most influenced by cytomegalovirus (CMV) serologic status of the donor. The underlying reason for this association is not completely understood, however, may be the immunogenic effect of CMV (see Chap. 68) on rejection and the development of bronchiolitis obliterans. Risk factors significantly associated with survival in the immediate postoperative period and at 1 year are outlined in Table 115-3.
Risk Factors Associated With Early and 1-Year Mortality
Early Postoperative Mortality | 1-Year Mortality |
---|---|
Bronchial dehiscence | Higher recipient age |
Severe pulmonary hypertension | Bilirubin |
Need for cardiopulmonary bypass | Supplemental oxygen requirements at rest |
Air leak | Lower cardiac output |
Primary graft dysfunction | Transplant center volume |
Recipient percentage predicted FEV1 | |
Donor-recipient height difference (larger donors do better) |
Demographic Shifts in practice: Lung transplant recipient demographic has changed with time. The median age of recipients in the most recent report from the ISHLT evaluating the 2006-2012 lung transplant era was 55, which has been increasing over the past few decades. The most striking increases have been in recipients older than 65 years of age. In the early 2000s, 2.8% of recipients were over the age of 65, compared to almost 10% from 2006 to 2012.30 With advancing age of recipients, intensivists need to appreciate that these patients carry more comorbid conditions and increasingly are undergoing concomitant procedures such as percutaneous coronary interventions or even combined transplants (heart-lung, lung-liver, lung-kidney).
Obesity continues to be a relative contraindication to lung transplantation (BMI >30). ISHLT has noted an association between higher BMIs and greater risk of death at 1 and 5 years.36 However, this notion is being challenged with more recent conflicting reports.37 Underweight and deconditioned individuals have also been shown to be at risk for poor outcomes.36
Given the critical shortage of lungs available, options to improve donor availability include extended criteria donors, non-heart beating donors, live lobar lungs, size reduced grafts, and the use of ex vivo lung perfusion for further evaluation and management of marginal lungs.38 Extended criteria donors do not meet the criteria outlined above as they may have atelectasis on their chest x-ray, infiltrates or moderate secretions on bronchoscopy. While some studies report equivalent early outcomes, others have demonstrated longer ICU stays and lower pulmonary function with the use of extended donor lungs.39 Currently, non-heart beating donors (NHBD) account for about 20% of all deceased organ donors. This shift in practice in addition to the adoption of strategies that include ex vivo lung perfusion technology and the use of extended criteria donors have significantly expanded the donor pool. Another strategy to increase transplant opportunities is lobar transplantation from live or deceased donors. However, there has been a decreasing trend for lobar lung transplants from living donors over the past decade. Many recipients who have small thoracic cavities have a prolonged wait time to find a size matched donor. Size reduced grafts help provide lungs to these patients and if the mismatch is significant, lobar transplants from the donor can be performed. While mortality and early function has been found to be equivalent, there is a trend toward more perioperative complications such as bleeding.
Since the first human lung transplant in the early 1980s, there has been considerable modification and refinement to the procedure. En bloc double lung transplant, which required cardiopulmonary bypass (CPB) and cardioplegia, has been replaced by bilateral sequential lung transplant with CPB limited largely to the pulmonary hypertension population. Both lungs are initially freed from the pleural space, the pulmonary arteries and veins are dissected and the bronchus is exposed at the hilum. The lung with lesser physiologic contribution, based on preoperative ventilation perfusion scans, is transplanted first as the other lung will more likely support single lung ventilation and avoid the need for CPB. End-to-end bronchial anastomosis is completed first followed by arterial and then venous anastomoses. The pulmonary arteries are trimmed in size to avoid unnecessary kinking and then anastomosed. The pulmonary veins (and corresponding preserved donor atrial attachment) undergo anastomosis with the left atrium of the recipient. After the second lung is transplanted, controlled reperfusion with leukocyte filtration is done slowly to minimize the incidence of primary graft dysfunction. Minimization of intravenous fluids without compromising end-organ perfusion has been used to reduce postoperative respiratory insufficiency. The pleural spaces are drained with chest tubes and the chest, muscle, and skin are closed. A flexible bronchoscopy is performed at the end of the transplant after exchanging the double lumen tube for a single lumen tube and the airway anastomoses are inspected.
Cardiopulmonary bypass may be indicated in patients with either isolated or coexisting pulmonary hypertension in the setting of ILD or COPD. These patients often have marginalized right ventricles that cannot withstand increases in pulmonary artery pressures that they are subjected to during the single lung ventilation. Unfortunately, conventional CPB increases the risk of bleeding, primary graft dysfunction and creates a more pronounced systemic inflammatory response syndrome (SIRS) postoperatively. The perioperative use of extracorporeal life support (ECLS) during double lung transplant has been explored with benefits being reported in a few small trials reporting lower incidents of ischemia reperfusion injury, anticoagulation, and SIRs.40
Ventilation: The early postoperative period may be characterized by an ischemia reperfusion injury in the lungs—known as primary graft dysfunction (PGD) (see “Postoperative Complications”). As a form of acute lung injury, many of the approaches that have been used to minimize lung injury and support gas exchange have been applied to PGD; however, few have been systematically evaluated. A lung-protective ventilator strategy (see Chaps. 51 and 52) is recommended in the postoperative period to minimize ventilator-induced lung injury. Often these patients have a pulmonary artery catheter to assist in following their pulmonary artery pressures in the setting of worsening hypoxia. Most patients can be weaned from mechanical ventilation rapidly. High airway pressures should be avoided as this leads to compression of collateral flow that the new lung depends on to perfuse the bronchial anastomosis. Some centers are adopting noninvasive positive pressure ventilation (NIPPV) as an option to shorten weaning time and to help minimize the risk of developing ventilator-associated pneumonia. Rapid extubation followed by prompt NIPPV may become a useful strategy in those who do not completely fulfill criteria for safe extubation.41
Fluid Management: Many risk factors can precipitate SIRS in these patients including cardiopulmonary bypass, primary graft dysfunction, and sepsis. As a result, these patients may succumb to noncardiogenic pulmonary edema from profound capillary leak. The new transplanted lungs have an impaired ability to clear edema given their severed lymphatic drainage at the time of transplant. It is necessary, therefore, to avoid substantial transplant lung edema by minimizing fluid and blood products wherever possible. While recognizing the inherent limitations associated with studies of fluid balances in the intensive care unit, a retrospective review that evaluated the associations between central venous pressure and outcome following transplant demonstrated a significant improvement in duration of mechanical ventilation and survival in the population of patients who had central venous pressures maintained below 7 mm Hg following transplant.42 Whether this association was causal needs to be validated in a prospective trial.
Chest Tubes: Chest tubes often remain in situ until air leaks have resolved and the patient has been extubated. Basal chest tubes may remain until postoperative days 5 to 7 given the high incidence of pleural effusions that could affect lung compliance. It is suggested that they are removed when draining less than 150 cc/24 hours.43
Bronchoscopy: A series of surveillance bronchoscopies are carried out in the first 2 years posttransplant. At our center, the first routine surveillance bronchoscopy is performed at 2 weeks, preferably when the patient is extubated. Bronchoscopies prior to that period are generally performed if clinically indicated.
Immunosuppression—Special Considerations for Lung Transplant: The mainstay of immunosuppressive management includes induction, maintenance, and antirejection therapy. The majority of transplant patients are maintained on lifelong immunosuppressive medications that are typically comprised of triple therapy with a calcineurin inhibitor (CNI), an antiproliferative agent, and a corticosteroid. In a meta-analysis of cyclosporine and tacrolimus, mortality at 1 year was comparable between the two medications, with the tacrolimus group experiencing fewer incidences of acute rejection, a trend toward lower chronic rejection, but a higher rate of diabetes.44 Both groups had an equal incidence of hypertension, renal dysfunction, and infection.
Azathioprine and mycophenolate mofetil (MMF) are the most common antiproliferatives used in lung transplantation. Based on data from other organ transplants, there is a suggestion that mammalian target of rapamycin inhibitors (mTOR) such as sirolimus and everolimus could result in less chronic rejection; however, large-scale studies in the lung transplant populations are pending. Sirolimus is associated with airway dehiscence and therefore is not recommended in the early posttransplant management.45
Corticosteroids remain the mainstay of immunosuppressive therapy. They are initiated in all lung transplant patients. While heart and liver transplant patients undergo aggressive steroid withdrawal within the first year, this has not been attempted in the lung transplant population given the dramatic consequences of graft loss.
Induction therapy use has increased to 62% in recent years; however, there remains no consensus about choice of agents. At present, polyclonal and monoclonal anti-T cell antibodies are used as induction agents and exert their effect by cytoreducing alloreactive T cells during a time when there exists a high donor leukocyte load (in the immediate posttransplant period).46 The two preparations of polyclonal antibodies that exist are equine antithymocyte globulin (ATG) and rabbit-derived ATG. Muromonab cd-2 (OKT3) is the only monoclonal antibody currently available. The clinical utility of these agents is limited by the occasional development of cytokine release syndromes. Preliminary studies evaluating the use of induction therapy have shown an improvement in 14-day survival.31 Approximately one half of transplant centers use some form of induction therapy. However, their long-term use is limited by the development of neutralizing antibodies. Induction therapy with IL-2R antagonists (daclizumab and basiliximab) is gaining popularity and has been shown to be associated with further reduced rates of acute rejection compared to no induction or the use of polyclonal agents.31
Table 115-4 summarizes routine postoperative management of the lung transplant patient.
Postoperative Management of Lung Transplant Recipient
Ventilation | Lung-protective ventilation: |
6 cc/kg, plateau pressures <30 mm Hg | |
Minimization of high airway pressures | |
Fluid | Judicious fluid administration |
Attempt to achieve dry weight without compromising end-organ function | |
Immunosuppressive medications | Maintenance: triple drug therapy with a calcineurin inhibitor, an antimetabolite, and a corticosteroid; avoid mTOR inhibitors in early posttransplant phase (risk of airway dehiscence) |
Induction: some centers adopted use of polyclonal antibodies | |
Antimicrobial prophylaxis | See section Infectious Complications |
Antimicrobial prophylaxis in CF | See section Infectious Complications |
Reducing complications within the first-year posttransplantation has had a significant impact on long-term survival. Multiple complications exist in the immediate posttransplant period that can lead to significant long-term morbidity and mortality. As a result, knowledge of the common complications is imperative to enhance long-term outcomes in this population. Below are the more common complications that can arise posttransplant.
Primary Graft Dysfunction: Primary graft dysfunction (PGD) is one of the most common complications after lung transplantation. It represents a forme fruste of noncardiogenic pulmonary edema and is characterized by progressive lung injury, with both epithelial and endothelial damage. PGD can develop as a result of an accumulation of insults on the lungs from donor management/ventilation, retrieval, storage, and implantation of the lungs. PGD typically occurs in the first few hours to 72 hours posttransplantation. PGD needs to be differentiated from other etiologies of poor gas exchange in the immediate posttransplant period (see Table 115-6). The ISHLT Working Group on PGD developed a standardized definition of PGD based on PaO2/FiO2 (P/F) ratio and chest infiltrates (see Table 115-5). It is a spectrum of injury that occurs to some degree in most patients but manifests clinically in 10% to 20% of patients.39 At its worst, it can progress to severe ARDS requiring maximum ventilatory support, and in some cases severely impairing pulmonary artery pressures due to refractory hypoxia necessitating inhaled NO, intravenous epoprostenol, or even ECLS. In less severe cases, it may resolve within 24 to 48 hours. The severity of PGD has been linked to both ICU outcomes and long-term graft function.47,48
Primary Graft Dysfunction
|
PGD is responsible for approximately 30% of early mortality postlung transplant (30 day) and is one of the greatest risk factors associated with a prolonged ICU stay.28 There are multiple risk factors for PGD. These include prolonged ischemic time, speed of reperfusion of the lungs, and unsuspected donor lung pathology such as aspiration or lung contusions (Table 115-6).
Risk Factors Independently Associated With Primary Graft Dysfunction
Donor Risk Factors | Recipient Risk Factors |
---|---|
Donor age >45 | Recipient BMI >25 |
Head trauma | Recipient female sex |
EuroCollins preservation solution | Recipient diagnosis: primary or secondary pulmonary fibrosis |
Single lung transplant | |
Increased ischemic time | |
Elevated recipient PAPa at time of transplant |
Given that PGD is largely transient and reversible, management is mainly supportive with the implementation of lung-protective ventilator strategies to minimize secondary injury from ventilator-induced lung injury. In the case of severe PGD, more advanced and, as yet, unproven treatments are often used to treat hypoxemia.
Fluids: Maintaining a negative fluid balance with diuresis, but without compromising end-organ function may help minimize the duration of PGD given the new lungs’ impaired ability to mobilize pulmonary edema. Whether the use of colloidal solutions or albumin offers an advantage in this situation has not been systematically evaluated.
Inhaled nitric oxide (iNO): In severe PGD, the role of iNO has been controversial with much of its support being extrapolated from ARDS studies. Similar to ARDS, it has been shown to improve oxygenation through enhancing ventilation/perfusion matching; however, this has not translated to an impact on mortality.48 While a randomized controlled trial showing survival benefit is lacking, a trial of iNO may be justified in select cases of severe hypoxemia or elevated pulmonary artery pressures. Although early preclinical and clinical evaluations suggested benefit in preventing PGD, an RCT did not show any benefit of iNO relative to placebo gas in reducing either the incidence or severity of PGD.49
Prostaglandin E1 (PGE1): In experimental models, the use of a PGE1 has been shown to ameliorate reperfusion injury and has the potential to be a promising option. It is often implemented in severe PGD refractory to mechanical ventilation and iNO.39 However, owing to its nonselective vasodilation it has the potential to contribute to both systemic hypotension and/or deterioration in ventilation/perfusion matching in the lung.
Extracorporeal life support (ECLS): Occasionally patients with severe PGD will be refractory to mechanical ventilation, iNO, and PGE1. ECLS has promise as a lifesaving alternative at this stage. Early initiation of ECLS (in the OR or within 24 hours) has led to survival rates of 50% to 80%.50 The evaluation of the Extracorporeal Life Support Organization (ELSO) registry of any patients receiving ECLS posttransplant report hospital survival rates of 42%.51 However, the effect of early ECLS on intermediate and long-term outcomes has not been systematically evaluated. Uniform criteria on when to initiate ECLS are needed. Improved experience in ECLS, better patient selection and timing, and refinements in technology have the potential to have a great impact on future outcomes of patients with PGD. Currently, an international multicenter trial is underway evaluating the use of ECLS for acute respiratory distress syndrome. While not directly related to primary graft dysfunction, given the similarities between the two entities, the results may help develop more concrete indications for initiation.
Endobronchial surfactant: The use of exogenous surfactant has been shown to reduce PGD in animal models and in some preliminary studies in humans. Further research is underway to determine if surfactant has any effect on patient-relevant outcomes.52-55
Acute Rejection: The incidence of hyperacute rejection, acute rejection, and chronic rejection has declined since the improvement in immunosuppressive regimens. Hyperacute rejection is currently a rare complication due to improvements in detecting HLA antibodies. Thirty six percent of patients will experience at least one episode of rejection.31 While chronic rejection could cause complications at a later time in the life of a transplant recipient, it is not commonly seen in the ICU as the primary etiology for presentation; however, beyond the first year of transplant, chronic rejection accounts for 25% of deaths.56
Hyperacute Rejection Hyperacute rejection occurs when the recipient has preformed antibodies to antigens on donor tissue (alloantigens). Fulminant rejection occurs within minutes of reperfusion. It manifests as acute respiratory failure, gross pulmonary edema with airway hemorrhage, and thrombosis within the graft. With improvement in screening techniques for preformed antihuman leukocyte antigen antibodies before transplant and the use of plasma exchange and thymoglobulin in a highly sensitized patient, the incidence has decreased. It is uncommon as it is only encountered when there is ABO incompatibility or if the recipient is sensitized to alloantigens from previous blood transfusions. Management includes urgent plasma exchange followed by agents to modify B-cell response such as rituximab.57
Acute Rejection Acute rejection is commonly seen after the first week to the first year posttransplant with the highest prevalence in the first 100 days after transplant. It is mediated by a recipient T-cell response against the graft. It is uncommon that an isolated episode of acute rejection would require ICU care; however, it has the potential to complicate the weaning process of a newly transplanted patient as it could develop early after transplant. Perivascular and interstitial infiltration of mononuclear cells are found on transbronchial or open lung biopsy. Clinically, acute rejection manifests as perihilar infiltrates, leukocytosis, hypoxia, airway inflammation, and low-grade fever in the absence of cultured organisms. Treatment includes pulse steroids (methylprednisolone 10-15 mg/kg) for 3 to 5 days followed by a 2- to 3-week steroid taper to the usual dose.
Patients noted to have a positive panel of reactive antibodies (PRA) are considered sensitized and are at greater risk of acute rejection posttransplant. PRAs screen for antihuman leukocyte antibodies, and a score (between 0 and 99—based on the percent of the population that recipient’s blood would react with) is assigned. However, controversy exists surrounding how to manage the patient with an elevated PRA. In the Toronto Lung Transplant program, if a patient has a positive PRA, virtual donor–specific antibody test, as well as positive cross match, we proceed with the administration of plasma exchange intraoperatively and on days 1, 2, 3, and 5 followed by administration of intravenous immunoglobulin and MMF (in lieu of azathioprine) as consolidation therapy. Antithymocyte globulin follows intravenous immunoglobulin in the absence of evidence of infection.
Bleeding: One of the most common complications requiring a return to the OR is postoperative bleeding. An unexplained drop in hemoglobin even in the absence of blood loss through thoracostomy tube drainage should raise this as a consideration. Fortunately, it has not been associated with an increase in perioperative mortality. Risk factors for postoperative hemorrhage include the presence of pleural adhesions in the recipient (more commonly seen in patients with cystic fibrosis, eosinophilic granuloma, or lymphangioleiomyomatosis) and the use of cardiopulmonary bypass.
Airway Complications: Six different airway complications of post-lung transplant include bronchial dehiscence, endobronchial infections, exophytic excessive granulation tissue formation, tracheobronchomalacia, bronchial fistulas, and bronchial stenosis. Bronchial dehiscence and endobronchial infections can arise in the immediate posttransplant period and will be the focus of this section. Table 115-7 provides more detailed information on the airway complications that may challenge the management of patients postoperatively. Bronchial complications were a significant source of morbidity and mortality in the early era of transplant being present in up to 80% of patients; however, with improved surgical techniques and newer immunosuppressive agents, they now occur in only 7% to 18% of patients.58
Airway Complications Following Lung Transplant
Complication | Time to Presentation | Definition | Management |
---|---|---|---|
Bronchial dehiscence | First 1-5 weeks |
|
|
Anastomotic infections | First 1-5 weeks |
|
|
Exophytic excessive granulation tissue formation | Weeks-months |
|
|
Tracheobronchomalacia | 4 months |
|
|
Bronchial fistulae | Weeks-months |
|
|
Bronchial stenosis | 2-9 months |
|
|
Bronchial necrosis and dehiscence is a potentially devastating consequence of transplant and is associated with a high mortality. Ischemia of the donor bronchus in the immediate posttransplant period is often the etiology of bronchial dehiscence. The dual blood supply to the bronchus in normal lungs arises from the pulmonary artery and from bronchial arteries originating from the intercostals off of the descending aorta. The bronchial arterial circulation to the anastomosis is disrupted during surgery and not reestablished until 2 to 4 weeks posttransplant. Therefore, the new lung’s airway anastomosis is dependent on the relatively low pressured, poorly oxygenated collateral blood flow from the pulmonary artery. Hypotension, compression from high airway pressures, and reperfusion injury leading to airway edema may also compromise flow and can contribute to ischemia. Ultimately this can lead to an environment for infection, extensive necrosis and then dehiscence. Late complications of stenosis can arise as healing and remodeling takes place. Necrosis and dehiscence is suspected in the setting of persistent air leak, lung collapse, difficulty weaning from the ventilator, subcutaneous emphysema, pneumomediastinum, and pneumothorax. The anastomosis must be carefully examined with flexible bronchoscopy. In addition, dehiscence may be further complicated by peribronchial abscess formation; therefore, a CT scan to rule out mediastinal air or surrounding infection/abscess should also be performed. Management includes minimizing high airway pressures, initiating appropriate antimicrobials if suspicion of secondary infection exists and either conservative management, endobronchial repair, or open repair depending on the severity.
Endobronchial infections occur due to the impairment of regional defense mechanisms (from ischemia, decreased mucociliary clearance, minimized cough reflex) and influenced by the ongoing use of high-dose immunosuppressant medications. This regional and systemic effect on immunological integrity fosters a rich environment for bacterial and fungal overgrowth. Saprophytic infections are the most frequently seen organisms as they are airborne and maintain nourishment from nonliving organic material in ischemic and necrotic debris. Aspergillus is the most frequently seen organism. Treatment includes a combination of bronchoscopic drainage, debridement, and systemic as well as inhaled antifungals. Antifungal prophylaxis has been advocated to minimize the risk of fungal anastomotic infections and is used in greater than 70% of the transplant programs within 24 hours after the procedure.58
Arrhythmias: Atrial fibrillation is seen in 20% of patients in the postoperative period with the peak incidence at 2 to 4 days.59
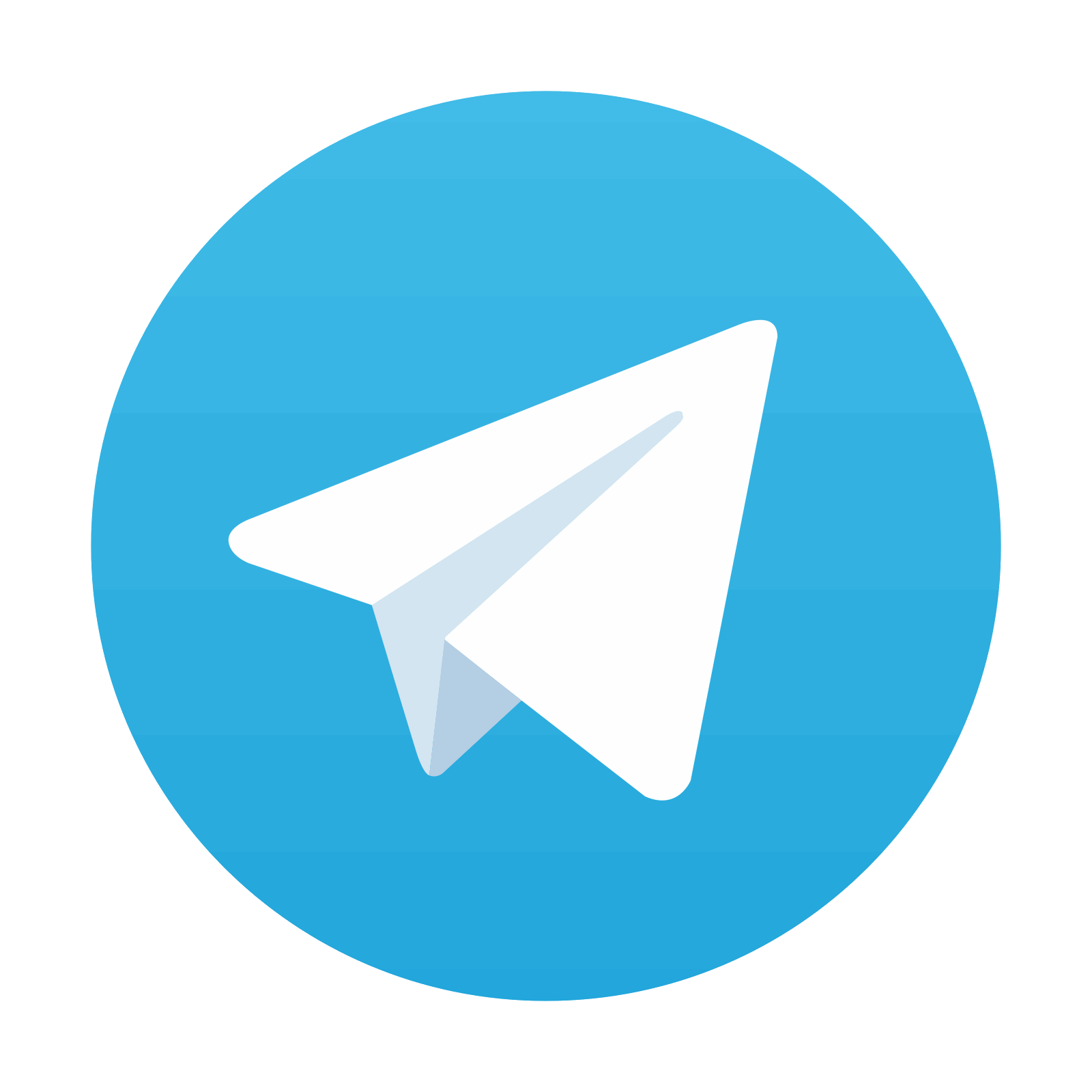
Stay updated, free articles. Join our Telegram channel

Full access? Get Clinical Tree
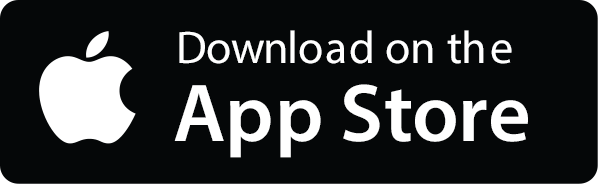
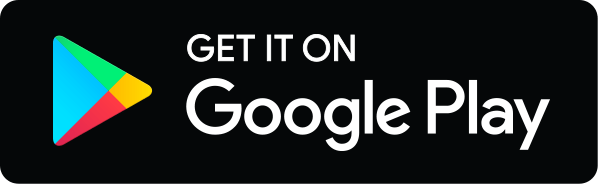
