The Respiratory System
Mark Burno
Casey A. Harper
Matthew Chao-Ben Chia
Christine M. Stock
▪ INTRODUCTION
The respiratory system, which consists of the upper and lower airways, lungs, and respiratory muscles, provides oxygen to the blood for delivery to cells and removes carbon dioxide that is generated by cell metabolism. Anesthesia and surgery may cause perturbations in lung function that may limit the ability of the lungs to perform their job successfully. Several monitors exist that allow monitoring of lung function, identification of abnormalities, and initiation of interventions to limit the potential detrimental effects caused by anesthesia and surgery. When the patient is subjected to mechanical ventilation, other monitors provide surveillance to ensure that the ventilator is properly supporting oxygen delivery and carbon dioxide elimination without causing harm to the lungs. By understanding the anatomy, physiology, and pharmacology relating to the respiratory system, the anesthesia technician can better help an anesthesia provider care for a patient undergoing surgery. This chapter introduces the technician to the respiratory system including anatomy, physiology, monitoring, commonly used medications that affect the respiratory system, and ventilator characteristics. This knowledge will ultimately provide a better understanding of the physiologic changes that occur during surgery and what must be done to support lung function.
▪ ANATOMY
Gases from the atmosphere, such as oxygen, enter the body through the mouth or nose and flow into the lungs where oxygen is delivered to the blood. At the same time, carbon dioxide moves from the blood into the lungs and during expiration it is expelled into the atmosphere or anesthesia circuit. This section presents the anatomy and structures of the upper airway, lower airway, lungs, and muscles involved in breathing.
Upper Airway
The upper airway consists of the nose, mouth, pharynx, and larynx (Fig. 11.1). The nose consists of the nasal septum, cribriform plate, lateral walls, and curved structures called conchae, which are also called turbinates. The conchae in the nasal cavity help to filter particles and also help to warm and humidify inspired gases. Olfactory and respiratory epithelia line the nose, forming a mucus blanket to further aid in filtering particles. The blood supply to the nose comes from the internal and external carotid arteries branching into the ethmoid arteries, sphenopalatine artery, and septal branch of the superior labial artery. Venous drainage occurs through the facial, ophthalmic, and sphenopalatine veins. Arterial supply and venous drainage in the nose are important because bleeding may occur during nasotracheal intubation. Because of the tendency to cause bleeding during insertion of a nasotracheal tube, medication to cause constriction of blood vessels in the nose is commonly used to decrease the risk of bleeding.
The mouth consists of the upper and lower dentition, tongue, and hard and soft palates. A layer of squamous epithelium helps protect the mouth from injury during chewing. Glands emptying into the mouth keep the mouth moist and help to humidify inspired gases. Gases moving through the mouth are heated, humidified, and filtered to a lesser degree than gases passing through the nasal passages. The blood vessels supplying the mouth are deeper than those supplying the nasal cavity, lowering the risk of significant bleeding. In addition, saliva helps to lubricate the mouth, which further decreases the risk of tissue injury and bleeding when equipment is inserted into the mouth.
The pharynx runs from the posterior nasal cavity passing posteriorly to the oral cavity to the superior portion of the esophagus. It is a tube
composed of muscle and connective tissue that helps to stent open the airway during inhalation and exhalation. The pharynx is further divided into the nasopharynx, oropharynx, and laryngopharynx. The nasopharynx is posterior to the nasal cavity. It consists of the posterior portion of the soft palate and muscles that form the posterior wall of the nasopharynx. Gases move through the nasopharynx to enter the oropharynx. The oropharynx begins at the posterior section of the mouth and consists of the posterior tongue, salivary glands, tonsillar pillars, and soft palate. Gases from the oropharynx pass into the laryngopharynx, which begins inferior to the oropharynx at the superior border of the epiglottis and extends inferiorly to the superior junction of the esophagus. It is posterior to the larynx and is in continuum with the esophagus.
composed of muscle and connective tissue that helps to stent open the airway during inhalation and exhalation. The pharynx is further divided into the nasopharynx, oropharynx, and laryngopharynx. The nasopharynx is posterior to the nasal cavity. It consists of the posterior portion of the soft palate and muscles that form the posterior wall of the nasopharynx. Gases move through the nasopharynx to enter the oropharynx. The oropharynx begins at the posterior section of the mouth and consists of the posterior tongue, salivary glands, tonsillar pillars, and soft palate. Gases from the oropharynx pass into the laryngopharynx, which begins inferior to the oropharynx at the superior border of the epiglottis and extends inferiorly to the superior junction of the esophagus. It is posterior to the larynx and is in continuum with the esophagus.
![]() ▪ FIGURE 11.1 Sagittal section of the head and neck, showing the relationships of the nasal cavity, mouth, pharynx, and larynx. (From Snell RS. Clinical Anatomy by Regions. 8th ed. Philadelphia, PA: Lippincott Williams & Wilkins; 2008, with permission.) |
The oropharynx and laryngopharynx contain muscles that aid in stenting open the pharyngeal passage during inspiration and expiration. Relaxation of these muscles during sedation, after the induction of general anesthesia, or during muscle paralysis, increases the chance of airway obstruction and difficulty with mask ventilation. The tongue also relaxes during sedation and may contribute significantly to airway obstruction by preventing gases from moving past the tongue and into the trachea. Extrinsic muscles of the tongue anchor it to different bones, allowing movement of the tongue in multiple directions. Relaxation of these muscles allows the tongue to move posterior and obstruct the pharynx.
The larynx is composed of muscle and cartilage and contains the epiglottis, the arytenoid cartilage, which borders the posterior entrance to the trachea, vocal cords, cricoid cartilage, hyoid bone, thyroid cartilage, and cricoid membrane (Fig. 11.2). It is located anterior to the laryngopharynx and superior to the trachea and may be divided into three sections called the supraglottic, glottic, and subglottic larynx. The vocal cords form the glottic opening and the entrance to the subglottic larynx and the trachea. The muscles and cartilage of the larynx are intricately associated in order to accomplish its functions. With elevation of the larynx, the epiglottis folds over the vocal cords to protect the trachea from
aspirating food or other material that enters the pharynx from the mouth or from the stomach. The thyroid cartilage is easily palpable in most people and is visible in many men as an anterior protrusion in the neck referred to as the Adam’s apple. The cricoid cartilage is a ring of cartilage just below the thyroid cartilage. It circles the larynx and is separated from the tracheal cartilage by the cricothyroid membrane. The cricoid cartilage may be important during induction of general anesthesia when the risk of aspiration of stomach contents is increased. The esophagus travels posterior to the cricoid cartilage; thus, direct posterior pressure to the cricoid cartilage can occlude the esophagus. This action decreases the chance of stomach contents passing passively through the esophagus and into the laryngopharynx to descend through the trachea and into the lungs. During intubation, a laryngoscope is inserted into the mouth past the tongue and into the posterior pharynx (Fig. 11.3). The epiglottis is identified and lifted to expose the arytenoids and vocal cords. An endotracheal tube can then be passed through the mouth and vocal cords into the trachea.
aspirating food or other material that enters the pharynx from the mouth or from the stomach. The thyroid cartilage is easily palpable in most people and is visible in many men as an anterior protrusion in the neck referred to as the Adam’s apple. The cricoid cartilage is a ring of cartilage just below the thyroid cartilage. It circles the larynx and is separated from the tracheal cartilage by the cricothyroid membrane. The cricoid cartilage may be important during induction of general anesthesia when the risk of aspiration of stomach contents is increased. The esophagus travels posterior to the cricoid cartilage; thus, direct posterior pressure to the cricoid cartilage can occlude the esophagus. This action decreases the chance of stomach contents passing passively through the esophagus and into the laryngopharynx to descend through the trachea and into the lungs. During intubation, a laryngoscope is inserted into the mouth past the tongue and into the posterior pharynx (Fig. 11.3). The epiglottis is identified and lifted to expose the arytenoids and vocal cords. An endotracheal tube can then be passed through the mouth and vocal cords into the trachea.
It is useful to be familiar with the nerves that gather sensory information from the nose, mouth, nasopharynx, oropharynx, laryngopharynx, and larynx. This knowledge will be helpful to understand how these nerves may be anesthetized when a patient requires a breathing tube to be inserted through the nose or mouth while awake. The internal nasal cavity is innervated by branches of the trigeminal nerve, which is named for its branches V1, V2, and V3. The branches continue further to form even smaller nerves. The internal nasal cavity is innervated by the V1 and V2 branches of the trigeminal nerve. Anesthetizing these nerves can be accomplished by applying topical anesthetics to the nasal cavity, which allows an endotracheal tube to be passed painlessly through the nasal cavity and into the nasopharynx.
The nerves that gather sensory information from the mouth include the trigeminal nerve V3 branch and the glossopharyngeal nerve.
Anesthetizing these nerves allows an endotracheal tube to be placed through the mouth into the oropharynx. The endotracheal tube may then be passed through the laryngopharynx after the mucosa of the laryngopharynx has been anesthetized.
Anesthetizing these nerves allows an endotracheal tube to be placed through the mouth into the oropharynx. The endotracheal tube may then be passed through the laryngopharynx after the mucosa of the laryngopharynx has been anesthetized.
![]() ▪ FIGURE 11.3 Direct laryngoscopy and endotracheal intubation. A: Direct view of upper airway; B: Side view of upper airway. (With permission from Nettina SM. The Lippincott Manual of Nursing Practice. 7th ed. Philadelphia, PA: Lippincott Williams & Wilkins; 2001.) |
Innervation of the larynx is provided by the superior laryngeal nerve and recurrent laryngeal nerve. Sensation from above the vocal cords (supraglottic and glottic) travels through the superior laryngeal nerve, while sensation from the subglottic larynx travels through the recurrent laryngeal. The laryngopharynx may be anesthetized by applying topical anesthetic on the lining of the laryngopharynx. The superior laryngeal nerve passes close to the skin near the hyoid bone before it supplies the lining of the laryngopharynx. This nerve can be easily anesthetized at this location with a small injection of local anesthetic (superior laryngeal nerve block). After anesthetizing the nerve by application of topical anesthesia or by nerve block, an endotracheal tube may be passed more comfortably through the upper airway, including the laryngopharynx and larynx, and into the trachea.
Lower Airway
Gases pass through the larynx and enter the trachea to flow into the lungs. The lower airway begins at the level of the trachea, just below the larynx. It includes the trachea, bronchi, bronchioles, respiratory bronchioles, alveoli, pulmonary arteries, pulmonary arterioles, and capillaries. The lungs consist of five lobes, which are distributed two on the left and three on the right. The two left lobes permit room in the thorax to accommodate the heart’s location in the left side of the chest. The lower airway is composed of tubes for airflow (conducting airways) and structures to allow gas exchange between the lungs and blood (respiratory airways).
The trachea branches into two mainstem bronchi. The branch point between the trachea and mainstem bronchi is called the carina. Visual
identification of the carina during intubation with a fiberoptic bronchoscope is helpful because the ideal location of the endotracheal tube is a few centimeters above the carina, allowing both lungs to receive gas from the tracheal tube. If the endotracheal tube is placed into one of the mainstem bronchi, only that lung will be ventilated. This is referred to as a mainstem intubation and usually results in decreased blood oxygen levels within minutes.
identification of the carina during intubation with a fiberoptic bronchoscope is helpful because the ideal location of the endotracheal tube is a few centimeters above the carina, allowing both lungs to receive gas from the tracheal tube. If the endotracheal tube is placed into one of the mainstem bronchi, only that lung will be ventilated. This is referred to as a mainstem intubation and usually results in decreased blood oxygen levels within minutes.
The trachea is a muscular tube reinforced by C-shaped partial rings of cartilage and is a conducting airway. The trachea terminates at the carina where the trachea divides into two bronchi called the left and right mainstem bronchi. The right mainstem bronchus branches from the trachea at a less acute angle than the left mainstem bronchus (Fig. 11.4). This difference in branching angles explains why liquid entering the trachea is more likely to enter the right lung. If a patient has stomach contents accidentally enter the trachea and descend into the bronchi, those contents are more likely to flow into the right mainstem bronchus. It is more difficult
for stomach contents to enter the left mainstem bronchus because the left bronchus diverges at a more acute angle from the trachea.
for stomach contents to enter the left mainstem bronchus because the left bronchus diverges at a more acute angle from the trachea.
![]() ▪ FIGURE 11.4 The trachea, carina, and bronchi. (From Snell RS. Clinical Anatomy by Regions. 8th ed. Philadelphia, PA: Lippincott Williams & Wilkins; 2008, with permission.) |
Identifying the right mainstem bronchus may be helpful in identifying the carina, which, in turn, facilitates ideal placement of the endotracheal tube. The right mainstem bronchus has a branch that occurs shortly after the trachea terminates at the carina to form the right and left mainstem bronchi. The first branch off of the right mainstem bronchus is called the right upper lobe bronchus. The right upper lobe bronchus is directed posteriorly, and identifying this bronchus during fiberoptic bronchoscopy helps to confirm that the scope is in the right mainstem and that a right double lumen endotracheal tube is properly positioned.
The trachea, bronchi, and bronchioles are conducting airways, which means that they only direct airflow into the lungs and out of the lungs. They do not participate in gas exchange between the airway and blood. The conducting airways are lined with respiratory epithelium and goblet cells that produce mucus and help to filter particles. The respiratory epithelium has cilia, which are tiny hair-like structures that beat in a coordinated motion to help move the mucus toward the larynx and into the laryngopharynx to be cleared into the esophagus. During general anesthesia with an endotracheal tube, the cilia are hindered from expelling the mucus into the esophagus resulting in mucus buildup that may need to be suctioned before removal of the endotracheal tube.
Respiratory bronchioles and alveoli participate in exchanging oxygen and carbon dioxide with the blood and are able to do so because the alveoli bring the inspired gas in close proximity to the blood in the capillary vessels. The alveoli are sacs that have very thin membranes to facilitate gas exchange with the capillaries (Fig. 11.5). Alveoli are the main sites where exchange of oxygen and carbon dioxide between the blood and lungs occurs. Groups of alveoli are normally clustered together like a bunch of grapes with a single grape termed an alveolus.
The tissue that makes up the alveoli and surrounding supportive tissue has an elastic component that gives the lungs a tendency to collapse if removed from the thorax. The lungs do not collapse under normal circumstances because they are adherent to the interior chest wall by a layer of fluid in the space between the lungs and the chest wall, the pleural space. The pressure in the pleural space is subatmospheric. This tendency for the lungs to remain adherent to the chest wall is similar to how two pieces of glass stick together when a thin layer of water is placed between the glass pieces. The lungs can slide along the chest wall during inspiration and expiration, but the lungs do not pull away from the chest wall or collapse under normal circumstances. If air enters the thorax through a defect in the chest or the lung, it may enter the pleural space, raising the pressure in the space and separating the lungs from the chest wall (the lungs collapse). Air in the pleural space is referred to as a pneumothorax.
The lungs and interior chest wall are both lined by a thin layer of tissue called pleura. The pleura lining the chest wall is the parietal pleura, while the pleura lining the lungs is the visceral pleura. Under normal circumstances, the parietal and visceral pleura meet and are only separated by a thin layer of fluid.
Elastic tissue in the lungs creates a force that has a tendency to collapse alveoli that counteracts the force exerted on the lungs by the chest wall and negative pressure in the pleural space to hold the lungs open. Furthermore, surface tension created by liquid lining the inner surface of alveoli adds to the forces collapsing alveoli. Surface tension occurs because the molecules in the liquid are attracted more strongly to themselves than they are to the gases in the alveoli and occurs where liquids meet gas. The entire interior surface of the sphere-shaped alveoli is coated in liquid; thus, the surface tension within the alveoli is significant. Since alveoli are spherical and liquid lines all alveoli, surface tension occurs throughout the alveoli. This force is disadvantageous to the alveoli because it could cause collapse of the alveoli. Surface tension would be a greater force causing alveoli to collapse if it were not for a substance called surfactant that also lines alveoli. The surfactant decreases the surface tension by decreasing the attractive forces between the water molecules. Decreasing the surface tension decreases the tendency for alveoli to collapse.
While the lungs have a tendency to collapse, the chest wall has the opposite tendency—to expand and thereby increase the intrathoracic volume. An equilibrium is established where the tendency of the lungs to collapse is balanced by the tendency of the chest wall to expand. The equilibrium normally occurs after exhaling a normal volume breath. If a patient has lung
disease or an abnormality of the chest wall, the equilibrium intrathoracic volume may be different. A lung or chest wall abnormality may cause this by changes in lung compliance or elasticity.
disease or an abnormality of the chest wall, the equilibrium intrathoracic volume may be different. A lung or chest wall abnormality may cause this by changes in lung compliance or elasticity.
Compliance of the chest wall and lungs determines at what lung volume the forces will be in balance. Compliance is how easily something changes volume when subjected to a pressure. For instance, some balloons inflate quite easily when air is injected. They have a high compliance and low elasticity. Other balloons, due to the thickness of their walls or composition of their material, require much more pressure to inflate and have low compliance and high elasticity.
Anatomy of the Thorax
The thorax is a cone-shaped cavity supported by vertebrae and protected by ribs. It houses the lungs, heart, and blood vessels. The volume of the thorax may be increased in three axes: the anterior-posterior direction, the superior-inferior direction, and the lateral-medial axis. The thoracic volume increases in the anterior-posterior and medial-lateral axes by elevating the ribs
because the ribs are set at a downward angle, much like the handle of a bucket, so that elevation of the ribs increases the diameter of the thorax and thus, the thoracic volume. The diaphragm creates the floor of the thorax and when it contracts it helps to increase the thoracic volume, and thus decrease the intrathoracic pressure (Fig 11.6). When a patient is face down, or prone, thoracic excursion is limited due to the weight of the patient on the thorax. Thus, anesthesia providers allow space for displacement of abdominal contents by the diaphragm during inspiration to allow lung volume expansion.
because the ribs are set at a downward angle, much like the handle of a bucket, so that elevation of the ribs increases the diameter of the thorax and thus, the thoracic volume. The diaphragm creates the floor of the thorax and when it contracts it helps to increase the thoracic volume, and thus decrease the intrathoracic pressure (Fig 11.6). When a patient is face down, or prone, thoracic excursion is limited due to the weight of the patient on the thorax. Thus, anesthesia providers allow space for displacement of abdominal contents by the diaphragm during inspiration to allow lung volume expansion.
Lung Volumes
People breathe in different volumes of gases depending on whether they are resting or engaged in vigorous physical activity. The volume of gases that is inspired each minute is called the minute volume. Different conditions and diseases may change the typical minute volume. A volume is a quantity of gas that can be inspired or expired and is not divided further into smaller quantities. A capacity is the combination of two or more volumes.
The volume of gas the lungs can hold after the deepest inspiratory effort that one could muster is referred to as the total lung capacity (Fig. 11.7). If a person were then to expel as much gas as possible there would still be some gas left in the lungs. The remaining gas volume is called the residual volume. When the person returns to breathing normally, the volume of gas inspired and expired is called the tidal volume. At end expiration while breathing quietly the tendency of the chest wall to expand is matched with the tendency of the lungs to collapse and equilibrium exists. This is what makes it quite comfortable to cease expiration at the end of expelling a tidal volume. If one were to continue to exhale, the process becomes active and muscle contraction is necessary. The functional residual capacity (FRC) is the volume of gas in the lungs at the end of a normal exhalation. The FRC correlates with the patient’s ability to tolerate apnea because it serves as a reserve source of oxygen.
Due to the importance of reserve oxygen, anesthesia providers often talk about FRC when discussing patients who are to receive anesthesia. The FRC contains oxygen that can be absorbed into the blood during a period of apnea. A lower FRC means that the patient does not have as much oxygen reserve; thus, there is less time between when the patient becomes apneic and when the oxygen saturation of hemoglobin in the blood begins to fall.
The thoracic volume at which equilibrium exists between the chest wall’s tendency to expand and the lung’s tendency to collapse may vary with certain patient conditions or diseases. For instance, in chronic obstructive pulmonary disease (COPD), the elastic component of the lungs is degraded, so lung compliance is increased. This means the lung volume at end expiration is larger than the lung volume in a person with healthy lungs. Alternatively, a patient with restrictive lung disease, where the lungs are stiffer, less compliant, and more elastic, has a smaller lung volume at end expiration. The smaller end-expiratory lung volume is due to the lungs having a greater force shrinking the size of the lungs that resists the chest wall’s tendency to want to expand and increase the lung volume.
Blood Supply to the Lungs
Blood flows from the right ventricle through the pulmonic valve and into the main pulmonary artery where it branches into the right and left pulmonary arteries and further branches into smaller and smaller arteries. The small arteries branch into even smaller arterioles and finally into capillaries (where gas exchange occurs). The capillary blood is collected by venules, which empty into veins that drain into the four main pulmonary veins. These veins empty into the left atrium.
The blood supply to the lungs comes from two sources. The first source is the pulmonary arterial system. The pulmonary arterial supply carries deoxygenated blood from the right side of the heart to the alveoli to be oxygenated and to unload carbon dioxide. Pulmonary arteries come from the heart and branch into arterioles before branching further into capillaries. Capillaries form networks around the alveoli, which facilitates gas exchange between the two so that oxygen may more easily diffuse into the blood and carbon dioxide may more easily diffuse into the alveoli. The second source of blood to the lungs is from bronchial arteries arising from the aorta that carry oxygenated blood to the lungs to supply the conducting lower airway structures that do not benefit from being in close proximity to gases inspired from the atmosphere.
▪ PHYSIOLOGY
Nose, Mouth, Pharynx, and Larynx
In this section we discuss the physiologic functions of the nose, mouth, pharynx, larynx, and lungs as they relate to air conditioning, respiration, and maintenance of homeostasis in the blood. The lungs are exposed to an enormous quantity of gases each day and contain delicate tissue in the alveoli that need to be protected. Protection occurs through filtering, humidifying, and heating the inspired gases to decrease the risk of damaging the airways and the alveoli. The lungs are also responsible for delivering oxygen to the blood and removing carbon dioxide; therefore, ventilation must be adequate to deliver inspired gas to the alveoli, while blood flow to the lungs must be adequate to absorb the oxygen and deliver carbon dioxide. A related concept is how the body matches gas delivery to the alveoli (ventilation) with blood supply to the capillaries surrounding those alveoli (perfusion) and is termed ventilation-perfusion matching. For optimum gas exchange to occur, ventilation and perfusion must be matched at the alveolar level.
Ambient temperature and humidity vary markedly, and the alveoli must be protected from cold and dry gases. Both the nose and mouth are able to heat, humidify, and filter inspired gases. The nose is more effective than is the mouth at these functions because the nose has a larger surface area over which the gases may flow. This surface area, created by the nasal mucosa and conchae, allows plenty of contact to transfer heat and humidity to the inspired gases. The hairs near the nares filter the gases and trap particles. The abrupt change in direction of airflow in the nasopharynx also traps particles. Due to their momentum, the moving particles do not abruptly change direction 90 degrees to descend into the oropharynx and instead impact in the nasopharynx. Gas flow through the mouth also abruptly changes direction in the oropharynx, which allows some particles to be filtered.
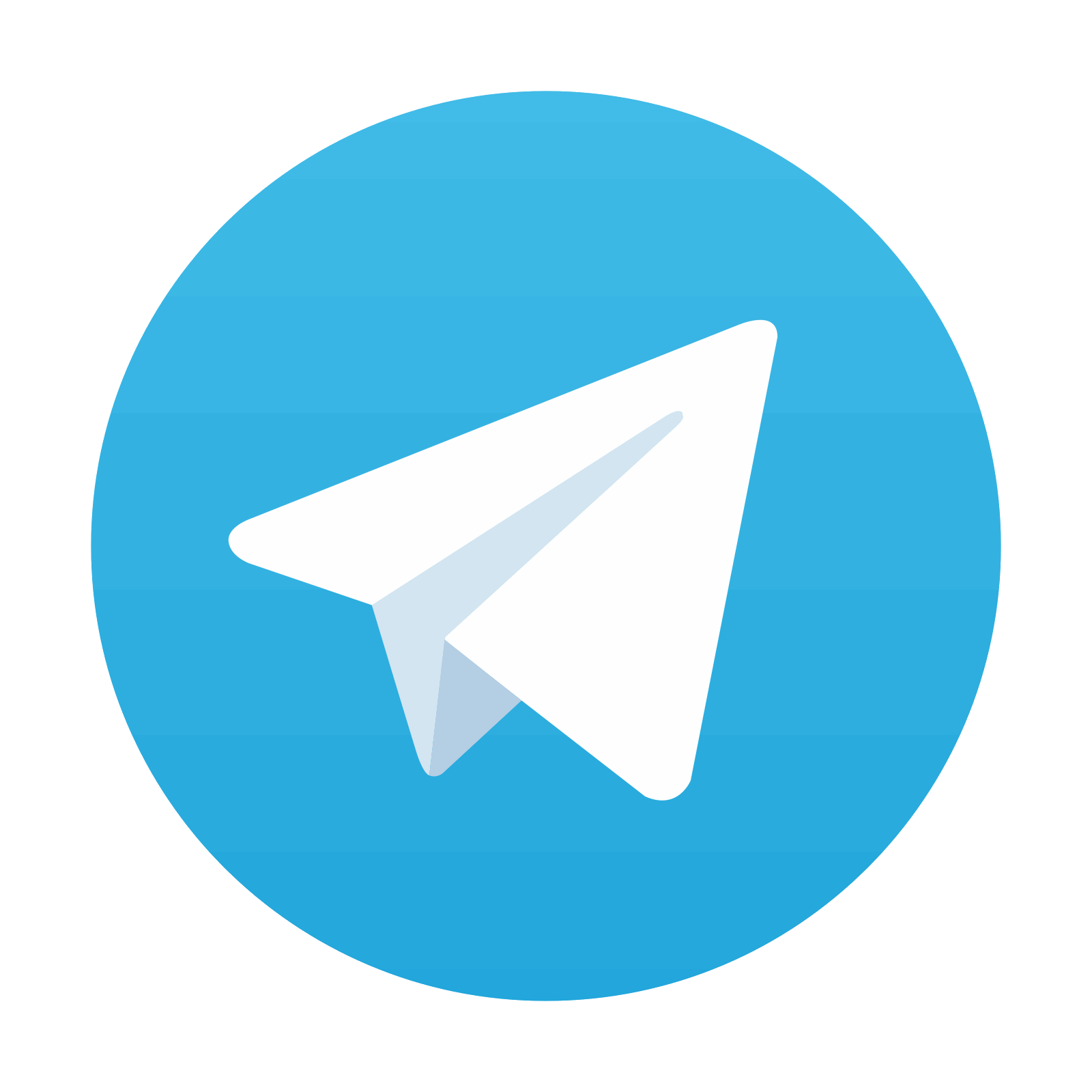
Stay updated, free articles. Join our Telegram channel

Full access? Get Clinical Tree
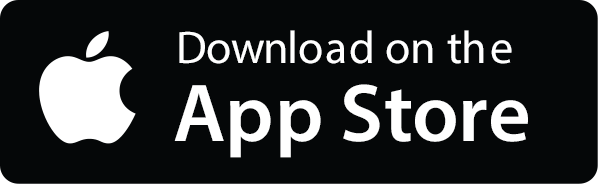
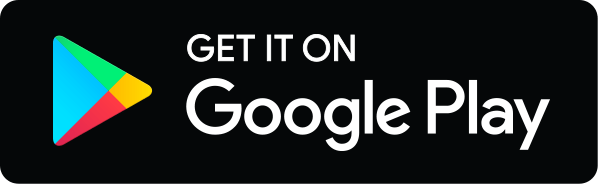