The rib cage undergoes structural changes during pregnancy (18). Progressive relaxation of the ligamentous attachments of the ribs causes the subcostal angle of the rib cage to increase early in pregnancy, persisting for months into the postpartum period. The increased elasticity of the rib cage is mediated by the polypeptide hormone, relaxin, which is increased during pregnancy and is responsible for the softening of the cervix and relaxation of the pelvic ligaments (19,20). Changes in pulmonary function during pregnancy are summarized in Figure 77.1.
Changes in Arterial Blood Gases
The hormonal changes of pregnancy lead to remarkable respiratory changes throughout its course. The resulting changes of arterial blood gas values have been measured by Sheldon (21) and Templeton and Kelman (22), who obtained serial measurements of maternal blood gases during pregnancy. The same investigators also measured serial alveolar-to-arterial oxygen tension differences (PAO2 – PaO2), and calculated the pulmonary venous admixture (physiologic shunt), dead space-to-tidal volume ratio (VD/VT), and respiratory minute volume (Table 77.3). The mean arterial PO2 was found to be consistently greater than 100 mmHg throughout pregnancy, with no alterations of dead space-to-tidal volume ratio (VD/VT) and shunt.
Cardiovascular System
Management of pregnancy, especially for women with heart disease, requires an understanding of gestational hemodynamic stress. The most important hemodynamic change in the maternal circulation during pregnancy is an increase in the cardiac output up to 45% (23), which can be primarily attributed to an increase in stroke volume, while heart rate and blood pressure do not change significantly (Fig. 77.2).
This alteration has several unique features: (a) the augmentation occurs relatively early in pregnancy (20 to 24 weeks); (b) it cannot be explained entirely on the basis of fetal needs; and (c) fluctuations in cardiac output occur with changes in body position as the gravid uterus impinges, to varying degrees, on the inferior vena cava, thus altering systemic venous return (25). Electrocardiographic (ECG) changes observed, but are of no clinical significance, include: sinus tachycardia, left axis deviation, ectopic beats, inverted or flattened T waves, and a Q wave in lead III (26).
TABLE 77.2 Indirect Maternal Deaths, 2000–2002a |
![]() |

FIGURE 77.1 Pulmonary changes of pregnancy (9). FVC, forced vital capacity; FEV1, forced expiratory volume in 1 second; RV, plethysmographic residual volume; FRC, plethysmographic functional residual capacity; TLC, plethysmographic total lung capacity.
Red Blood Cell, Plasma, and Blood Volume
An increase of plasma volume is evident by the sixth week of gestation, reaching a value by the end of the first trimester of 15% above that of the nongravid state. There is subsequently a steep increase of this parameter until 28 to 30 weeks of gestation, followed by a more gradual rise, to a final volume at term of 55% above the nonpregnant level (27). Red blood cell mass decreases during the first 8 weeks of gestation due to a decrease in the life span of erythrocytes (23), but increases to nearly 30% above the nonpregnant level at term. These physiologic changes result in a 45% increase of total blood volume and a reduction of the hemoglobin concentration and hematocrit to values of approximately 11.6 g/dL and 35.5 volume %, respectively (Fig. 77.3). Estrogens, progesterone, and placental lactogen elevate aldosterone production either directly or indirectly, and are responsible for the increase of plasma volume during pregnancy. The hyperaldosteronism of pregnancy can result in retention up to 500 to 900 mEq of sodium and an increase of 6,000 to 8,000 mL of total body water, 70% of which is extracellular. The elevated red blood cell volume after 8 to 12 weeks can be attributed to increased serum erythropoietin. Erythropoiesis may also be stimulated by prolactin, progesterone, and placental lactogen. Changes of blood counts during pregnancy are summarized in Figure 77.3.
Plasma Proteins, Colloid Osmotic Pressure, and GFR
The total serum protein concentration decreases from the nonpregnant value of 7.3 to 6.5 g/dL at term gestation. The change is due primarily to a decline of the albumin concentration, which decreases from a nonpregnant level of 4.4 to 3.4 g/dL at term. Although the concentration of globulins declines by 10% during the first trimester, the level rises subsequently to a value at term that is 5% to 10% above the nonpregnant level. These changes result in a progressive decrease in the albumin-to-globulin ratio from approximately 1.5 during pregnancy to 1.1 at term gestation. Maternal colloid osmotic pressure decreases in parallel with the decline in serum albumin concentration from nonpregnant values of 25 to 26 mmHg to approximately 22 mmHg at term. During pregnancy, the glomerular filtration rate (GFR) increases 50% as compared to pregravid levels (28).
TABLE 77.3 Blood Gas Analysis in Late Pregnancya (22) | |||
![]() |

FIGURE 77.2 Cardiovascular changes of pregnancy (24). HR, heart rate (bpm); B/Ps, systolic blood pressure (mmHg); B/Pm, mean blood pressure (mmHg); B/Pd, diastolic blood pressure (mmHg); CI, cardiac index (L/min/m2); SV, stroke volume (mL); EDV, end-diastolic volume (mL).
Aortocaval Compression
Angiographic studies show that the aorta and inferior vena cava can be significantly compressed by the gravid uterus when the woman is in the supine position. In fact, Kerr et al. (29) observed a complete obstruction of the inferior vena cava at the level of the bifurcation in 80% of women in late pregnancy. Partial obstruction of the aorta at the level of the lumbar lordosis (L3–L5) has also been demonstrated in patients between the 27th week of pregnancy and term gestation (30,31).

FIGURE 77.3 Changes of blood count during pregnancy (27). RCM, red cell mass in mL/10; Bvol, blood volume in mL/kg; Hb, hemoglobin in g/100 mL; Hct, hematocrit in %; BL, prepregnant data; early, 8th week of pregnancy; mid, 14th week of pregnancy; late, 20th week of pregnancy.
The pregnant woman at term, when placed in the lateral decubitus position, exhibits a right ventricular filling pressure (central venous pressure [CVP]) similar to that of a nonpregnant woman (32). This observation suggests that venous return in this position is maintained by the collateral circulation despite partial caval obstruction (29). In the plain supine position, however, right atrial pressure falls substantially, demonstrating that collateral circulation cannot compensate for complete, or nearly complete, vena cava obstruction (30). The fall in the cardiac filling pressure that follows this position change, evident by 20 to 28 weeks of gestation, results in a decreased stroke volume and cardiac output of approximately 25%, and a 20% reduction of uterine blood flow (33); these are reliably improved by a tilt to the left of at least 25 degrees (34).
Despite the reduction of cardiac output and stroke volume, a position change from lateral to supine can be associated with elevation of blood pressure, resulting from an increase of systemic vascular resistance due to compression of the aorta by the gravid uterus and enhanced sympathetic nervous system outflow (35). In approximately 5% of women, however, a substantial drop in blood pressure occurs (“supine hypotensive syndrome”), which is associated with bradycardia (usually following a transient tachycardia) and maternal symptoms of low systemic perfusion, such as of pallor and sweating, possibly followed by cardiocirculatory collapse. This occasional, but profound, drop of venous return may be exacerbated by neuraxial block, the preferred method of providing anesthesia in pregnant women (36). In conclusion, and based on the observations above, the intensivist should always consider in his or her emergency treatment plan the proper positioning of the pregnant patient and its influence on hemodynamics.
DIAGNOSIS AND TREATMENT
Thrombosis and Thromboembolism in Pregnancy
Venous thromboembolism (VTE), which includes deep venous thrombosis (DVT) and pulmonary embolism (PE), occurs in approximately 1 in 1,000 pregnancies (37). Women are five times more likely to develop VTE during pregnancy than during a nonpregnant state (38). Fatal PE, a possible sequela of VTE, remains a leading cause of maternal mortality in the Western world (39). The rate of PE in pregnancy is five times greater than that for nonpregnant women of the same age, and is about 1 in 100 deliveries; the risks are even higher in the puerperium.
Risk Factors and Predisposition to Venous Thrombosis
Compared to nonpregnant women, pregnant women have a 10-fold higher risk of a thrombotic episode. Risk factors for VTE other than pregnancy are noted in Table 77.4 (40,41).
Pregnancy is associated with an increased clotting potential, decreased anticoagulant properties, and decreased fibrinolysis. Pregnancy is accompanied by a two- to threefold increased concentration of fibrinogen and a 20% to 1,000% increase in factors VII, VIII, IX, X, and XII, all of which peak at term (42). Levels of von Willebrand factor (vWF) increase up to 400% at term (42). Free protein S levels decline significantly (up to 55%) during pregnancy due to increased circulating levels of its carrier molecular, complement 4 binding protein (42). As a consequence, pregnancy is associated with an increase in resistance to activated protein C (42,43). Levels of plasminogen activation inhibitor-1 (PAI-1) increase three- to fourfold during pregnancy, while plasma PAI-2 values, negligible before pregnancy, reach concentrations of 160 mg/L at delivery (35).
TABLE 77.4 Risk Factors for Venous Thromboembolism (VTE) During Pregnancy |
![]() |
Pregnancy is also associated with venous stasis in the lower extremities, due to compression of the inferior vena cava and pelvic veins by the enlarging uterus and hormone-mediated increases—in circulating levels of estrogen and local production of prostacyclin and nitric oxide—in deep vein capacitance secondary. Important hereditary risk factors that can increase DVT risk are antithrombin III deficiency, protein S and C deficiency, a G1691A mutation of the factor V gene (44), and a G20210A mutation of the factor II gene (45).
Diagnosis of Venous Thromboembolism during Pregnancy
Bates and Ginsberg have recently addressed the diagnosis of VTE during pregnancy in detail (49). In pregnant women presenting with lower extremity edema, back pain, and/or chest pain, the prevalence of VTE is less than in the general population because of the high frequency of these complaints related to pregnancy. D-dimer assays, which can be used to exclude VTE in healthy nonpregnant individuals, usually become positive late in pregnancy, which decreases the utility of this assay (47). Radiologic studies used to diagnose VTE in the nonpregnant woman have not been validated in pregnancy, and potential risks to the fetus, particularly in terms of ionizing radiation exposure, need to be considered (48). Compression ultrasonography (CUS) of the proximal veins has been recommended as the initial test for suspected DVT during pregnancy (46). When results are equivocal or an iliac vein thrombosis is suspected, magnetic resonance venography (MRV) can be used. MRV does not carry the radiation risk of contrast venography, and is becoming increasingly available in the United States.
The approach to the diagnosis of PE is similar in the pregnant and nonpregnant individual. Ventilation/perfusion (V/Q) scanning confers relatively low radiation exposure to the fetus, a risk less than that of missing a diagnosis of PE in the mother. However, when a V/Q study is indeterminate in a pregnant woman without demonstrated lower extremity thrombosis, it is usually followed by angiography. A brachial approach carries less radiation exposure to the fetus than spiral computed tomography (CT).
Prevention of Thrombosis during Pregnancy
The optimal anticoagulation regimen has not been established. Low–molecular-weight heparins (LMWHs) have become the anticoagulant of choice because, like unfractionated heparin (UFH), they do not cross the placenta, have better bioavailability, and carry less risk of osteoporosis and heparin-induced thrombocytopenia than UFH (49,50). A recent review of published data on the use of LMWHs in pregnancy supports their use as safe alternatives to UFH as anticoagulants during pregnancy (51). A more recent practice trend, especially in the United States, has been to switch patients to the longer-acting, subcutaneous UFH a few weeks before delivery to allow the use of activated partial thromboplastin time (aPTT) as a diagnostic test to assess anticoagulation pre- and postlabor (52).
Another means of providing VTE prophylaxis is with elastic compression stockings, which may be used for the entire pregnancy. Elastic stockings are appropriate for in-hospital patients at increased risk of VTE, and may be combined with the use of LMWH. Vena cava filter placement represents a potentially important, but poorly evaluated, therapeutic modality in the prevention of pulmonary emboli. Randomized trials to establish the appropriate role of vena cava filters in the treatment of venous thromboembolic disease are lacking (53).
Thrombolytic Therapy for Pulmonary Embolism
The indications for thrombolytic therapy for PE remain controversial. The incidence of intracranial hemorrhage may be as high as 2% to 3% with systemic thrombolytic therapy (54), although rates were lower in a recent trial (55). Fatality rates in patients with PE presenting in cardiogenic shock may be as high as 30% (54); thrombolytic therapy should be considered in this circumstance, although evidence for this subgroup is limited (56). Approximately 10% of symptomatic pulmonary emboli are rapidly fatal (57,58). The International Cooperative Pulmonary Embolism Registry, established to ascertain PE mortality, reported that 2% of patients were first diagnosed with PE at autopsy (59). Of patients diagnosed with PE before death, 5% to 10% have shock at presentation, which is associated with a mortality of 25% to 50% (59–61). Echocardiographic evidence of right ventricular dysfunction at presentation also has been suggested as an indication for thrombolytic therapy (55); however, a recent randomized trial failed to demonstrate a survival benefit with thrombolysis in patients with this finding (55), and mortality rates with conventional therapy are conflicting (54). At the time of this writing, routine thrombolysis cannot be justified in all patients.
Hemorrhage
Peripartum hemorrhage (PPH) remains a significant cause of maternal and fetal morbidity and mortality. In the United States and other industrialized nations, massive obstetric hemorrhage has generally ranked among the top three causes of maternal death despite modern improvements in obstetric practice and transfusion services.
PPH includes a wide range of pathophysiologic events. Antepartum bleeding occurs in nearly 4% of pregnant women (62). The causes of serious antepartum bleeding are abnormal implantation (placenta previa, placenta accreta), placental abruption, or uterine rupture. The latter is often caused by a dehiscence of a pre-existing uterine scar. The main reason for postpartum bleeding is uterine atony when myometrial contraction is inadequate. It is not surprising that uterine bleeding may be fatal when considering the massive amount of blood flow perfusing the uterus at term (up to 600 mL/min).
TABLE 77.5 Management of Severe Postpartum Hemorrhage |
![]() |
Patients with hemodynamic instability or massive hemorrhage require prompt resuscitative measures, including the administration of supplemental oxygen, placement of at least two large bore* intravenous lines, intravenous hydration with isotonic crystalloid, and blood typing and cross-matching for the replacement of packed red blood cells (Table 77.5). A delay in the correction of hypovolemia, diagnosis and treatment of impaired coagulation, or surgical control of bleeding are the avoidable factors in most maternal mortality cases caused by hemorrhage. If a transfusion must be given before full cross-matching is finished, type-specific uncross-matched blood can be used (63); alternatively, if there is no cross-matched blood, type O, Rh negative blood may be used in an emergency. Several recent studies suggest that fibrinogen is an important predictor of severe PPH (64,65). Standard coagulation screening tests such as the prothrombin time, partial thromboplastin time, and fibrinogen concentration have been supplemented or replaced by global coagulation tests such as thromboelastography (TEG) or rotational thrombelastography (ROTEM) (66).
There is virtually unanimous agreement from professional societies on the need of multidisciplinary hemorrhage protocols for management of both trauma and PPH (67–69). Such protocols ensure rapid availability of prepared blood products and a concomitant reduction in the time to transfusion and resuscitation. If the placenta has not been delivered when hemorrhage begins, it should be removed, if necessary by manual exploration of the uterine cavity. Placenta accreta is diagnosed if the placental cleavage plane is indistinct. In this situation, the patient should be prepared by the intensivist or the anesthesiologist for probable urgent hysterectomy. Firm bimanual compression of the uterus (with one hand in the posterior vaginal fornix and the other on the abdomen) can limit hemorrhage until help can be obtained. Hemorrhage after placental delivery should prompt vigorous fundal massage while the patient is rapidly given 10 to 30 units of oxytocin in 1 L of intravenous crystalloid. Uterotonic agents such as oxytocin are routinely used in the management of uterine atony (70). This synthetic nonpeptide is a first-line therapeutic agent because of the paucity of side effects and the absence of contraindications. If the fundus does not become firm, uterine atony is the presumed (and most common) diagnosis. Although fundal massage continues, the patient may be then given 0.2 mg of methylergonovine (Methergine) intramuscularly, with this dose to be repeated at 2- to 4-hour intervals if necessary. Methylergonovine, an ergot alkaloid, is used as a second-line uterotonic agent in the setting of massive obstetric hemorrhage due to uterine atony. It may cause undesirable adverse effects such as cramping, headache, and dizziness. Coexisting severe hypertension is an absolute contraindication to its use.
Injectable prostaglandins may also be used when oxytocin fails. Both prostaglandin E and prostaglandin F2 stimulate myometrial contractions, and have been used intramuscularly or intravenously for refractory hemorrhage due to uterine atony. In particular, carboprost (Hemabate), 15-methyl prostaglandin F2-α, may be administered intramuscularly or intramyometrially in a dosage of 250 μg every 15 to 90 minutes, up to a maximum dosage of 2 mg. Sixty-eight percent of patients respond to a single carboprost injection; 86% respond to a second dose (71). Because oxygen desaturation has been reported with the use of carboprost (72), patients should be monitored by pulse oximetry.
The use of a hydrostatic balloon has been advocated as an alternative to uterine packing for controlling hemorrhage due to uterine atony (73). The inflated Rusch balloon can conform to the contour of the uterine cavity and provides an effective tamponade. Life-threatening hemorrhage can also be treated via arterial embolization by interventional radiology (74). Finally, in cases of continuing hemorrhage, a variety of surgical techniques can be used to avoid a hysterectomy, such as bilateral uterine artery ligation or internal iliac artery ligation (75).
Amniotic Fluid Embolism
Although the entry of amniotic fluid into the maternal circulation was already recognized as early as 1926 (76), Morgan published the first major review on the topic in 1979 (77). He reviewed 272 cases reported in the English language literature to that date. Although the true incidence of this disease entity is not known, most authors estimate it to be between 1 in 8,000 and 1 in 80,000 pregnancies.
Clinical Presentation
The classic presentation of amniotic fluid embolism is described as a sudden, profound, and unexpected cardiovascular collapse followed, in many cases, by irreversible shock and death (78). The only known predisposing factor to this life-threatening complication of pregnancy appears to be multiparity, seen with 88% of cases (77). In a smaller percentage of cases (51%), the presenting symptom is respiratory related. Hypotension is present in 27% of surviving cases, with coagulopathy comprising 12% and seizures 10%. Fetal bradycardia (17%) and hypotension (13%) are the next most common presenting features (Table 77.6).
TABLE 77.6 Clinical Presentation of Amniotic Fluid Embolism |
![]() |
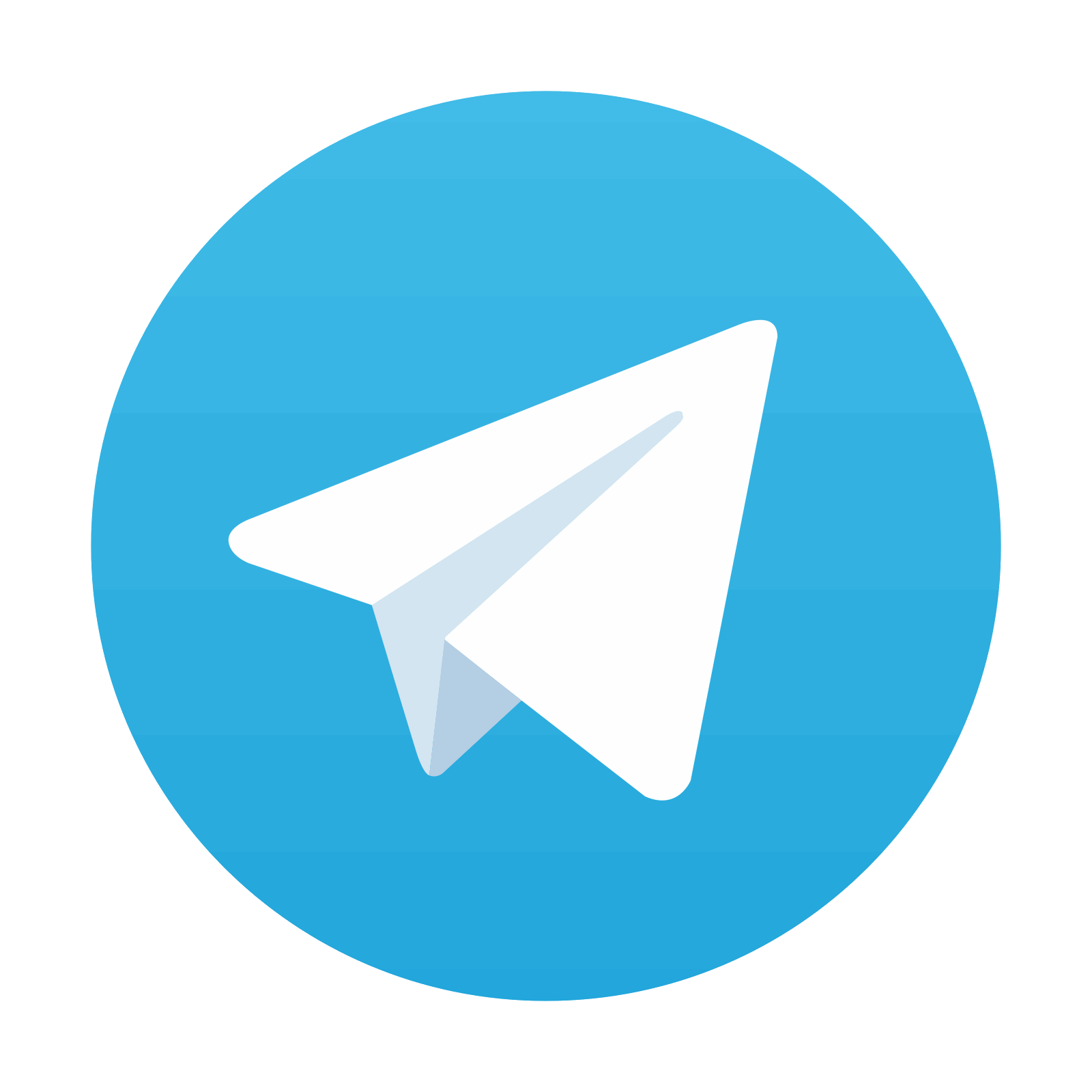
Stay updated, free articles. Join our Telegram channel

Full access? Get Clinical Tree
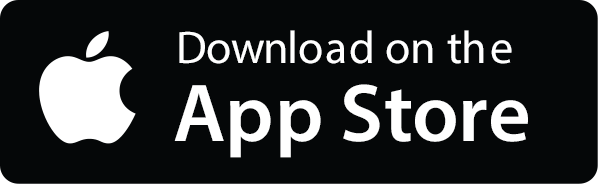
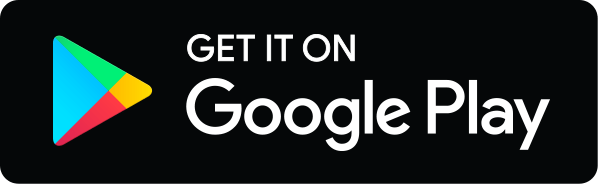