KEY POINTS
Extreme obesity presents unique cardiorespiratory challenges in the intensive care unit and frustrates the delivery of routine care.
Extreme obesity leads to a variety of cardiovascular diseases through diverse mechanisms. Therefore, a high index of suspicion for their presence is warranted in the critically ill patient.
Many patients with extreme obesity suffer from varying degrees of pulmonary hypertension. Potential etiologies include the obesity hypoventilation syndrome, the “overlap syndrome” of coexisting obstructive sleep apnea and chronic obstructive pulmonary disease, chronic venous thromboembolic disease, and left heart failure. Diastolic dysfunction is common and may contribute to this pathology.
Although simple obesity has relatively minor effects on pulmonary function, extreme obesity may be associated with reductions in forced vital capacity, forced expiration volume in 1 second, and total lung capacity. Arterial hypoxemia may be present, particularly in the supine position.
Unrecognized sleep-disordered breathing in the critically ill patient may contribute to cardiopulmonary failure. It may also confer increased sensitivity to sedatives and narcotics.
An increased risk of venous thromboembolism in obesity merits an aggressive approach to prophylaxis.
Intraabdominal pressure is elevated in obesity, placing the patient at increased risk for the abdominal compartment syndrome.
Extreme obesity is associated with a significant increase in the percentage of oxygen consumption attributable to the work of breathing. This decreased respiratory reserve results in a predisposition to the development of respiratory failure even after trivial insults.
Atelectasis is common in the extremely obese postoperative patient and, along with sleep-disordered breathing, may lead to respiratory failure. The early use of noninvasive ventilation in the high-risk postoperative patient may prevent the development of respiratory failure.
Intubation of the extremely obese patient may be technically challenging because of poor visibility of the glottis and decreased oxygen stores in alveoli from a reduced functional residual capacity.
Extremely obese patients should be ventilated in the upright or semi-upright position to improve respiratory system compliance and reduce the work of breathing. Positive end-expiratory pressure between 8 and 15 cm H2O may be necessary to prevent atelectasis.
Because the compliance of the respiratory system is reduced in extreme obesity, a high plateau pressure does not necessarily indicate alveolar overdistention. When using low tidal volume ventilation in the management of the acute respiratory distress syndrome, a plateau pressure of 35 to 40 cm H2O may be acceptable in some patients.
Ultrasound guidance may be useful in establishing vascular access in the extremely obese patient.
Numerous unpredictable alterations in pharmacokinetics have been described in obesity. Reference to published guidelines for individual drugs and close monitoring of clinically available serum drug levels are recommended.
Nutritional support in the form of carefully balanced hypocaloric enteral regimens is recommended.
Interestingly, while obesity is associated with increased all-cause mortality, the preponderance of evidence suggests that ICU outcomes are not worse in the obese critically ill patient.
An ever-increasing percentage of the inhabitants of developed countries is obese or overweight. This trend includes men and women and spans all age groups, including children. Obesity is associated with diabetes mellitus, cardiovascular disease, hypertension, and cancer and confers a reduced life expectancy, particularly in younger and extremely obese individuals. Extreme obesity is frequently associated with life-threatening cardiopulmonary disease and presents substantial obstacles to the delivery of routine care. We present a summary of the challenges involved in caring for the extremely obese critically ill patient and highlight physiological principles important to the care of such patients.
THE OBESITY EPIDEMIC
Due to the impracticality of determining body fat composition in the clinical setting, obesity is typically defined as excessive weight relative to body surface area as determined by calculation of the body mass index (BMI), calculated as weight in kilograms divided by height in square meters. The correlation between BMI and obesity in most middle-age adults is very good, although using BMI as a proxy for body fat is misleading in individuals engaged in weight training who have increased muscle mass, and when significant edema is present. The World Health Organization definition of overweight is a BMI greater than 25 kg/m2 but less than 30 kg/m2, while obesity is defined as a BMI greater than 30 g/m2. Obesity has been further subclassified into mild (class I) obesity with BMI 30 to 34.9 kg/m2, moderate (class II) obesity with BMI 35 to 39.9 kg/m2, and extreme/severe/morbid (class III) obesity with BMI 40.0 kg/m2 or greater. A relatively new category of “super obesity” has been proposed for BMI greater than 50 kg/m2.
The prevalence of obesity in the United States has increased dramatically in both men and women over the past 50 years (Fig. 130-1). In 2007-2008 data from the National Health and Nutrition Examination Survey indicated that approximately 34.2% of all US adults were overweight, 33.8% were obese, and 5.7% were extremely obese.1 There are racial and ethnic differences in the prevalence of overweight and obesity, with the highest rates occurring in non-Hispanic black women and Mexican American women. Of greatest concern is the high prevalence of obesity in children and adolescents.2
The obesity epidemic is the result of an interaction between genetics and what has been called the “obesigenic” environment. Technologic advances have decreased the cost of food production, thereby making food more affordable, and decreased the energy expended by the typical worker.3 Leisure time is increasingly dominated by sedentary activities such as watching television or using the computer. Television viewing is associated with increased food intake and a decrease in metabolic rate even when compared with other sedentary activities such as reading or sewing.4 The risks of obesity and type II diabetes mellitus have been positively correlated with the amount of television watched,5 and children randomized to an intervention discouraging television viewing had significant reductions in relative BMI compared with controls.6 Relatively recent data suggest that long-term societal reductions in sleep quantity may play a role in the obesity epidemic. A number of studies performed in young adults indicate that short-term sleep curtailment is associated with decreased insulin sensitivity and glucose tolerance, elevated sympathovagal balance, increased levels of the hunger-promoting hormone ghrelin, decreased levels of the satiety hormone leptin, and increased hunger.7 In addition, studies performed in mice suggest that exposure to low levels of light at night—for instance, in the form of indoor lighting and exposure to television and personal electronic devices—may promote obesity by disrupting the timing of food intake.8 Other possible explanations for the obesity epidemic include an increased percentage of meals eaten outside the home, the serving of larger portions at commercial establishments, and the widespread consumption of diets low in vegetables and fibers and high in refined sugars. Individual susceptibility to these influences is poorly understood but likely includes a genetic component.
Unfortunately, the obesity epidemic is not confined to highly developed countries. The World Health Organization estimates that 1 billion people worldwide are overweight or obese. Worse, while there is some evidence that increases in obesity prevalence may be plateauing in the United States, most indications are that the worldwide epidemic has not yet peaked. Obesity is increasing in prevalence in developing countries coincident with decreased physical activity and the replacement of traditional fruits and whole grains in the diet with calorie-dense, processed foods.
PHYSIOLOGIC EFFECTS OF EXTREME OBESITY
Obesity is associated with a reduced life expectancy, particularly in the extremely obese.9 Obesity is associated with cardiovascular disease, diabetes, sleep apnea, lung disease, liver and gallbladder disease, chronic kidney disease, and cancer. This section summarizes some of these physiologic derangements, with an emphasis on those germane to the care of the extremely obese critically ill patient (Table 130-1).
Critical Care Considerations in the Extremely Obese Patient
|
Increases in BMI above 25 kg/m2 are associated with progressive increases in the risk of death from ischemic heart disease, stroke, and other vascular diseases, the latter category including heart failure and hypertensive disease.9 Obesity promotes cardiovascular disease through a variety of mechanisms.10 First, obesity increases the risk of coronary atherosclerosis by inducing several risk factors in parallel. For example, obesity is associated with hypertension, insulin resistance, dyslipidemia, and coagulation abnormalities, which separately and collectively promote the development of cardiovascular disease. Impaired fibrinolysis and systemic inflammation are suggested by the frequent elevation of plasminogen activator inhibitor I, fibrinogen, and interleukin-6 and C-reactive protein levels. The metabolic syndrome in particular represents a cluster of risk factors for atherosclerosis.11
There are other, more direct mechanisms through which obesity causes heart disease.10 Obesity may impair cardiac function through chronic pressure and volume overload. Cardiac output is increased in obesity as a result of increased extracellular volume and increased blood flow to most tissue beds. This increased cardiac output is associated with increased preload and cardiac dilation, with the subsequent development of eccentric left ventricular hypertrophy. This chronic volume-overloaded state, when combined with increased left ventricular afterload from concurrent hypertension, may result in marked left ventricular hypertrophy. Over time, left ventricular hypertrophy leads to impaired ventricular filling and diastolic heart failure. Systolic heart failure may result from ischemic heart disease, microvascular disease from diabetes mellitus, longstanding hypertension, or, in severe, longstanding obesity, a decrease in mid-wall fiber shortening and ejection fraction.12
Pulmonary hypertension with right ventricular hypertrophy and dilation may accompany obesity. Frequently, the pathogenesis is multifactorial: for instance, due to the combination of diastolic heart failure and untreated sleep disordered breathing. Importantly, uncomplicated obstructive sleep apnea alone results in only mild pulmonary hypertension. The clinical implication of this is that the clinician who encounters a patient with moderate to severe pulmonary hypertension is obligated to search for causes in addition to obstructive sleep apnea. Occult diastolic dysfunction and chronic thromboembolic disease should be considered. The so-called “overlap syndrome” describes the relatively common presentation of the patient who has both obstructive sleep apnea and chronic obstructive pulmonary disease, a condition that may lead to severe pulmonary hypertension.13 Cor pulmonale may also develop in patients with the obesity hypoventilation syndrome (OHS).14 This poorly understood disorder is associated with daytime hypercapnia and hypoxemia, with the latter arising from alveolar hypoventilation and poor ventilation of the basal lung due to airway closure and atelectasis. Hypercapnia and hypoxemia elicit pulmonary vasoconstriction. Eventually, this may lead to vascular remodeling with resulting irreversible pulmonary hypertension and cor pulmonale. Individuals with the OHS usually, but not always, have coexisting obstructive sleep apnea.
Interestingly, patients with cardiovascular disease who are overweight and obese tend to have better outcomes than patients with who are not.15 There are many potential explanations for this so-called “obesity paradox,” including the possibility that patients who develop cardiovascular disease through obesity-related mechanisms may develop more mild forms of disease.
What are the clinical implications of this susceptibility? First, the intensivist caring for the extremely obese patient should have a high index of suspicion for the presence of ischemic heart disease from coronary artery or microvascular disease. Second, abnormal cardiac function, diastolic or systolic, may be present, even if other risk factors for heart disease are absent. Particular sensitivity to changes in intravascular volume may result. Third, pulmonary hypertension and right ventricular failure should be suspected when obstructive lung disease and/or daytime hypoxemia or hypercapnia are present. The diagnosis of these conditions is complicated by poor sensitivity of physical examination and transthoracic echocardiography in extremely obese individuals. In selected patients, transesophageal echocardiography or invasive hemodynamic monitoring may be necessary.
Mechanics of the Respiratory System: The effect of obesity on pulmonary function varies considerably between individuals, with most patients exhibiting relatively normal pulmonary function but some patients experiencing more significant effects.16-18 In general, abnormalities accrue as the BMI increases, and with increases in thoracic and abdominal (eg, central) adiposity. The most predictable effect of obesity is a decrease in the functional residual capacity (FRC) caused by the mass load of adipose tissue around the rib cage and abdomen and in the visceral cavity.18 In extremely obese patients, the FRC may little exceed the residual volume, predisposing to small airway closure and atelectasis, particularly in the dependent areas of the lung. In addition, the overall compliance of the respiratory system is reduced, mildly so in simple obesity and to as low as 45% of normal in patients with OHS. This has historically been thought to be due to a combination of reduced lung compliance and reduced chest wall compliance. Conceivably, the former may arise from some combination of dependent airway closure and increased surface tension related to breathing at low lung volumes, and from increased thoracic blood volume. Studies of chest wall compliance have yielded conflicting results. However, it is likely the case that, while the configuration of the pressure-volume curve of the chest wall is relatively normal, chest and abdominal adiposity impose an inspiratory threshold load to breathe. In other words, while the initiation of the breath requires more effort, once this threshold is surpassed chest wall compliance is normal.19
Airway function may be abnormal in some patients with obesity.20,21 There is some evidence that airway resistance is increased beyond that expected from a reduction in airway caliber due to reduced lung volume. In addition, the effects of bronchoconstriction on expiratory flow limitation and airway closure may be more pronounced in obesity as a consequence of smaller lung volumes and airway caliber. Whether obesity is itself associated with increased airway hyperresponsiveness is controversial.
Pulmonary Function: The most frequent abnormality in pulmonary function in obesity is a decrease in the expiratory reserve volume attributable to cephalad displacement of the diaphragm by adipose tissue. In extremely obese individuals and in those with the OHS, total lung capacity and vital capacity may be reduced. In such patients, the residual volume actually may be increased relative to total lung capacity because of small airway closure and gas trapping. This is supported by the finding of larger total lung capacity by body box plethysmography than by helium dilution. Similarly, spirometry is typically normal in simple obesity, whereas severely obese individuals or those with the OHS may exhibit reductions in the forced expiratory volume in 1 second and in the forced vital capacity, although the ratio of these two variables is preserved or even increased.
Why do some individuals exhibit diminished pulmonary function and/or the OHS, whereas comparably overweight individuals may be little affected? Some, but not all, data suggest that the distribution of body fat may be an important determinant of pulmonary function; simply put, adipose tissue that is more centrally located is more likely to negatively influence pulmonary function. There may also be differences in respiratory muscle strength between patients with simple obesity and those with the OHS, with the latter exhibiting relatively decreased inspiratory muscle strength. Weakness may be considered absolute, a result of mechanical disadvantage from diaphragm malposition, and relative, when the increased work of breathing from extreme obesity is considered (see below).
Gas Exchange: Extreme obesity causes closure of small peripheral airways in the dependent regions of the lung, resulting in mismatching of ventilation and perfusion. The result may be a widened alveolar to arterial oxygen gradient and mild to moderate hypoxemia that worsens in the supine position. Severe hypoxemia may be present in individuals with the OHS because of the additional contribution of hypoventilation. The diffusing capacity for carbon monoxide is typically normal or even elevated when indexed to alveolar volume.
Control of Breathing: Ventilatory drive is increased in simple obesity as assessed by the mouth occlusion pressure and diaphragm electrical activity in response to carbon dioxide inhalation. In contrast, mouth occlusion pressure response to carbon dioxide is reduced in OHS compared to normal individuals and to patients with simple obesity. Diaphragm electrical activity in response to carbon dioxide is inappropriately low in OHS. In obese patients with OSA, hypercapnea is associated with the severity of OSA, with increasing BMI, and with the degree of restrictive chest wall mechanics.22
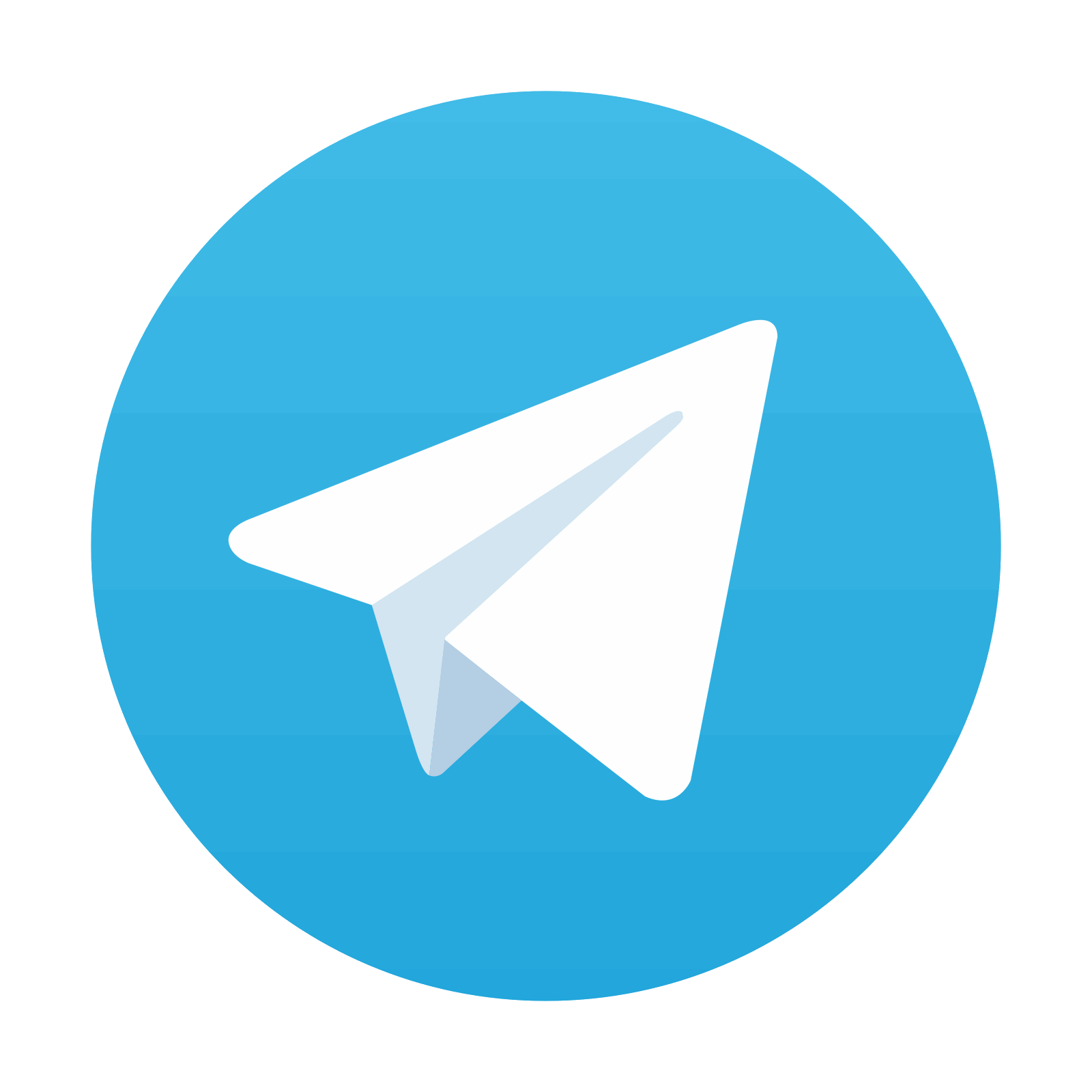
Stay updated, free articles. Join our Telegram channel

Full access? Get Clinical Tree
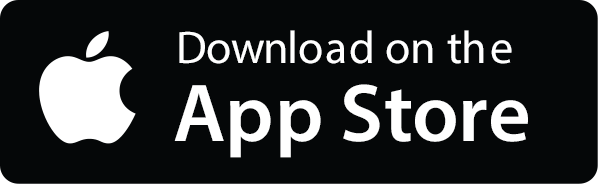
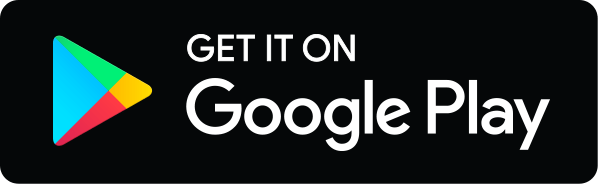