The Management of Acute Radiation Casualties
Lawrence C. Mohr Jr
Introduction
It has been stated by the nation’s political and military leadership that it is not a matter of “if” but “when” another terrorist attack will occur within the continental United States. Such future attacks could include the use of a radiological dispersion device, commonly called a “dirty bomb,” an attack on a nuclear power plant or the detonation of a nuclear weapon. Indeed, a nuclear attack by a group of rogue terrorists is the single greatest risk to our homeland security. The objective of this chapter is to become familiar with the medical consequences of a radiological or nuclear attack and the management of casualties that could result from such an attack. It is to help you to think about the “unthinkable.”
Radiological Weapons of Terrorism
Radiological dispersion devices, or “dirty bombs,” consist of radioactive materials that are placed around a high explosive charge. The radioactive material is released and dispersed by detonation of the high explosive charge. Dirty bombs are easy to make and raw materials are readily available throughout the world. It is important to note that dirty bombs are not nuclear weapons and are not weapons of mass destruction. Their adverse health effects depend on the type and amount of explosive used, the type and amount of radioactive material used, and atmospheric conditions at the time of detonation. Most injuries from a dirty bomb will come from the blast effects of the conventional explosion [1,2]. Acute radiation health effects are very unlikely. Delayed health effects, such as the development of cancer, are also unlikely. The risk of developing cancer following a dirty bomb attack is related to the radiation exposure dose and to the amount of internal radiation that results from the inhalation, ingestion, and absorption of radioactive material through the skin or open wounds. Long-term psychological trauma is likely to occur among some members of a population who have been exposed to radioactive material from a dirty bomb [2,3].
A nuclear explosion results from nuclear fission or from thermonuclear fusion, in which a tremendous amount of energy is suddenly released in the form of heat, blast, and radiation. Human injury is caused by exposure to a combination of these three forms of energy following a nuclear detonation. The radiation exposure from a nuclear explosion can be very intense and lead to a life-threatening acute radiation syndrome, radiation burns, thermal burns, and blast injuries. Such radiation exposure can also result in the development of various types of cancer and leukemia over a period of many years if an individual survives the short-term initial effects of a nuclear explosion [4,5,6].
The life-threatening acute radiation syndrome may develop in some radiation-exposed individuals following a nuclear explosion. The acute radiation syndrome consists of a continuum of complex and unique medical sub-syndromes that involve the hematopoietic, gastrointestinal, and central nervous systems in a dose-related fashion. Patients who develop any of the acute radiation sub-syndromes require prompt assessment and critical care management in order to minimize loss of life. It is essential that any physician who may be called upon to treat patients following a nuclear explosion be familiar with the diagnosis and management of these unique sub-syndromes [7]. The acute radiation syndrome and its associated sub-syndromes are discussed in detail later in the chapter.
Basic Radiation Physics
In order to understand the medical aspects of radiation exposure, it is important to review some basic principles of radiation physics. Radiation is defined, simply, as energy that is transmitted through space. The transmitted energy may be in the form of high-speed particles or electromagnetic waves. There are two general types of radiation: ionizing radiation and nonionizing radiation. Ionizing radiation has enough energy, so that when it interacts with an atom, it can remove tightly bound electrons from their orbits and cause the atom to become charged. Nonionizing radiation, on the other hand, does not have enough energy to remove electrons from their orbits. Ionizing radiation is more harmful to humans than nonionizing radiation and is the type of radiation that would be expected to be released in a radiological or nuclear attack.
Ionizing radiation can take four forms: alpha, beta, gamma, and neutron radiation. Alpha radiation consists of the emission of a helium nucleus from a parent nucleus, such as 235Uranium; it is a particle that has an atomic mass of four and a charge of plus one. Beta radiation is the emission of a small negatively charged particle from a parent nucleus, such as 40Potassium. Beta particles have a mass that is almost undetectable and a charge of minus one, similar to that of an electron. Gamma radiation is the emission of high-energy electromagnetic waves from a parent nucleus, such as 60Cobalt. Gamma rays have no mass, no charge, and frequently accompany the emission of alpha or beta particles. Neutrons are very high-energy particles that are emitted from parent nuclei, such as 235Uranium and 239Plutonium during a nuclear chain reaction. Nuclear chain reactions can be controlled, such as the kind found in a nuclear reactor, or they can be uncontrolled, such as the type that causes a nuclear explosion. Neutrons are very damaging to human cells and tissues [8,9].
Each specific type of ionizing radiation has a different penetrating distance with respect to inert material and human tissues. Alpha particles will not penetrate paper or human skin. Indeed, you can safely hold an alpha-emitter, such as 240Plutonium, in your hand as long as you do not have any breaks in the skin. However, if alpha particles are ingested, inhaled, or internalized through a break in the skin, they can do
a tremendous amount of internal damage to human cells and tissues [8,9].
a tremendous amount of internal damage to human cells and tissues [8,9].
Beta radiation will penetrate paper, thin layers of skin, and the conjunctiva of the eye, but will not penetrate thin layers of plastic or aluminum foil. Beta particles travel relatively short distances and will be stopped by most clothing. As with alpha particles, beta radiation is more damaging to human tissues if inhaled or ingested [8,9].
Gamma rays can travel significant distances and are a highly penetrating type of ionizing radiation. They readily penetrate skin and clothing. Gamma radiation can cause considerable damage to human cells and tissues after penetration. Several inches of lead or several feet of concrete are required to stop gamma rays [8,9].
Neutrons are high-energy nuclear particles that have no charge. Neutron radiation easily penetrates skin and clothing and can cause significant damage to internal tissues and organs. Neutrons primarily cause biological damage by colliding with other particles. They transfer the most energy when they collide with particles that are about the same size, especially protons. These high-energy, subatomic collisions result in the dislodgement of both protons and tightly bound electrons from atoms that are bombarded by neutrons, with ionization of atoms in surrounding cells and tissues. Neutron radiation is extremely harmful to humans. It is not stopped by plastic, glass, or lead; it can only be stopped by several feet of concrete [8,9].
Ionizing radiation causes damage to human cells and tissues through two biological mechanisms: (i) direct high-energy damage to DNA molecules and (ii) the generation of free radicals, which secondarily damage DNA molecules by superoxide radicals generated from ionized water. The fate of irradiated human cells is dependent on the dose of radiation exposure. Low-dose exposures are characterized by DNA and cellular repair. Moderate-dose exposures are characterized by permanently damaged DNA and significantly altered cells, which may be eliminated by apoptosis, or reproduce abnormally and eventually lead to the development of cancer. High-dose radiation exposures typically result in cell death, which causes several serious, acute radiation syndromes that can result in death of the organism [10,11].
Human radiation exposure can be categorized as either external or internal. External exposure, which involves exposure to the skin, may be either whole body or partial body depending on the surface area exposed to radiation. Internal exposure may occur from the inhalation, ingestion, or transdermal penetration of radioactive material. Combined radiation injuries may also occur in cases where radiation exposure and trauma occur concurrently. In combined radiation injuries, radioactive material is introduced through open wounds [8].
Radiation Doses
There are two units of radiation dose that physicians must be familiar with: the Rad and the Gray. It is not essential for physicians to understand the physics that underlie the determination of these doses, but it is important for them to know that these are the units which are used to express the amount of radiation that is absorbed by human tissues. The Rad is the traditional unit of radiation absorbed dose. One Rad is defined as 100 ergs per g. The Gray (abbreviated Gy) is the newer Standard International unit of radiation exposure. One Gy is equal to 100 Rads, which is defined as 1 J per kg. One hundred centi-Gray (100 cGy) are equal to 1 Gray [12].
Table 215.1 Lymphocyte Count Between 24 and 48 Hours After Radiation Exposure, Estimated Dose Range (Gy), and Estimated Lethality (%) | |||||||||||||||||||||
---|---|---|---|---|---|---|---|---|---|---|---|---|---|---|---|---|---|---|---|---|---|
|
Radiation doses can be measured by several techniques. A radiac meter is an instrument that directly measures radiation dose using a Geiger–Müller tube or similar device. There are many different types of radiac meters, each of which may be more sensitive to specific types of radiation, such as alpha, gamma, or neutrons, than to other types of radiation. It is important, therefore, to know both the capabilities and limitations of any radiac meter that one uses to determine radiation doses. Most radiac meters in use today are highly portable and will accurately measure alpha, beta, gamma, and neutron radiation. The measurement of the lymphocyte count between 24 and 48 hours after exposure can provide a useful biological estimate of radiation dose, especially in the clinical setting [13]. The dose range in Gy and the estimated lethality associated with each dose range is illustrated in Table 215.1.
Chromosomal aberrations and translocations in lymphocytes can provide a useful estimate of both the type of radiation that one has been exposed to as well as the radiation dose [14]. This method requires considerable expertise in fluorescent in situ hybridization techniques as well as expertise in the interpretation of the chromosomal abnormalities. As a result, the analysis of chromosomal aberrations is primarily used as a research tool at the present time.
Overview of Radiation Casualties
Radiation casualties consist of two general types: an acute radiation syndrome and delayed illnesses that may occur many years after radiation exposure. Acute radiation syndrome is a life-threatening condition consisting of a continuum of dose-related sub-syndromes that occur shortly after a high-dose radiation exposure, such as may occur following the detonation of a nuclear weapon. Delayed illnesses include leukemia, lymphoma, and various solid tumors, which may occur later in life following radiation exposure doses that are lower than those needed to produce acute radiation illness. In general, the higher the radiation dose, the more severe the acute effects of radiation exposure, the greater the probability of delayed illnesses, and the higher the mortality rate [4,7,15].
In considering the human dose-response to radiation exposure, a measurement known as the LD50/60 is commonly used. The LD50/60 is the radiation dose that causes a 50% mortality rate in an exposed population within 60 days following exposure. For whole-body radiation exposure, the LD50/60, with no treatment, is 3 to 4 Gy. Therefore, 50% of a population that receives a radiation dose of 3 to 4 Gy will die within 60 days unless they receive treatment. With appropriate treatment and
supportive care following radiation exposure, the LD50/60 is 4 to 5 Gy [7,12].
supportive care following radiation exposure, the LD50/60 is 4 to 5 Gy [7,12].
Acute Radiation Syndrome and Sub-Syndromes
Acute radiation syndrome is a continuum of dose-related organ system sub-syndromes that develop after an acute radiation exposure of greater than 1 Gy. There are three main sub-syndromes that occur: the hematopoietic sub-syndrome, the gastrointestinal sub-syndrome, and the central nervous system sub-syndrome. Each of these sub-syndromes occurs in a dose-related fashion. The hematopoietic sub-syndrome occurs with radiation exposures greater than 1 Gy. The gastrointestinal sub-syndrome occurs in addition to the hematopoietic sub-syndrome at radiation exposures greater than 6 Gy. The central nervous system sub-syndrome occurs in addition to the hematopoietic and gastrointestinal sub-syndromes at radiation exposures greater than or equal to 20 Gy.
All acute radiation sub-syndromes begin with a prodromal phase that lasts for 2 to 6 days. This phase is characterized by nausea, vomiting diarrhea, and fatigue. The higher the radiation dose, the more rapid the onset, and the more severe the symptoms of the prodromal phase. After 2 to 6 days of the prodromal phase, the patient enters a latent phase, in which he or she appears to recover and is totally asymptomatic. The latent phase lasts for several days to 1 month, with the time period inversely proportional to the radiation exposure dose, that is, the higher the dose, the shorter the latent period. After the asymptomatic latent period, the patient enters the manifest illness phase. This phase of acute radiation illness lasts from several days to several weeks and is characterized by the manifestation of the hematopoietic, gastrointestinal, and central nervous system sub-syndromes, according to the exposure dose that the patient received [16].
Some individuals may develop a radiation-associated multiple organ dysfunction syndrome in association with the organ-specific clinical syndromes mentioned in the previous paragraph. Multiple organ system dysfunction typically occurs in the manifest illness phase, but may also occur early after a sublethal radiation exposure. Patients with hematopoietic, gastrointestinal, central nervous system, and multiple organ dysfunction syndromes will require management in an intensive care unit [17,18].
The Hematopoietic Sub-Syndrome
The hematopoietic sub-syndrome typically occurs with a radiation dose of greater than 1 Gy. It is characterized by bone marrow suppression resulting from the radiation-induced destruction of hematopoietic stems cells within the bone marrow. Hematopoietic stem cell destruction results in pancytopenia, which is characterized by a progressive decrease in lymphocytes, neutrophils, and platelets in the peripheral blood. Both the magnitude and the time course of the pancytopenia are related to the radiation dose. In general, the higher the radiation dose, the more profound and the quicker the pancytopenia occurs [7,16].
Lymphocytic stem cells are exquisitely sensitive to radiation and circulating lymphocytes decrease rapidly following radiation exposure and remain low for a long period of time. Erythrocytic stem cells, on the other hand, seem to be more resistant to radiation than lymphocytic, neutrophilic, and thrombocytic stem cells. Therefore, the red blood cell count and hemoglobin concentration typically do not decrease to the same extent as lymphocytes, neutrophils, and platelets following radiation exposure. Hematological effects that occur in the manifest illness phase of the hematopoietic sub-syndrome following radiation exposures of 1 Gy and 3 Gy are depicted in Figure 215.1.
As seen in Figure 215.1, the hematological effects of acute radiation exposure are dependent on the radiation dose. A radiation exposure of 3 Gy or more results in significant hematological effects than a radiation exposure of 1 Gy. Lymphocytes will decrease very rapidly following a radiation exposure of 3 Gy, and they will stay low for a relatively long period of time. Typically, it takes about 90 days before lymphocytes begin to recover from a 3 Gy radiation exposure. Neutrophils, after an initial period of intravascular demargination, will also begin to decline fairly rapidly following a 3 Gy exposure. Neutrophils do not fall as rapidly as lymphocytes, but between 3 and 5 days following exposure, such patients will be significantly neutropenic. Platelets also decrease steadily following a 3 Gy exposure and patients will become significantly thrombocytopenic at 2 to 3 weeks. Both platelets and neutrophils will reach a nadir, with values close to zero, at about 30 days following a 3 Gy exposure. Platelets and neutrophils then recover gradually during the next 30 days. Lymphocytes remain low for a long period of time, however, and typically do not begin to recover for at least 90 days following a 3 Gy exposure. Thus, there is a period of about a month following a 3 Gy exposure when patients will be significantly lymphopenic, neutropenic, and thrombocytopenic. Such patients are susceptible to developing serious infections and serious bleeding problems during that time [7].
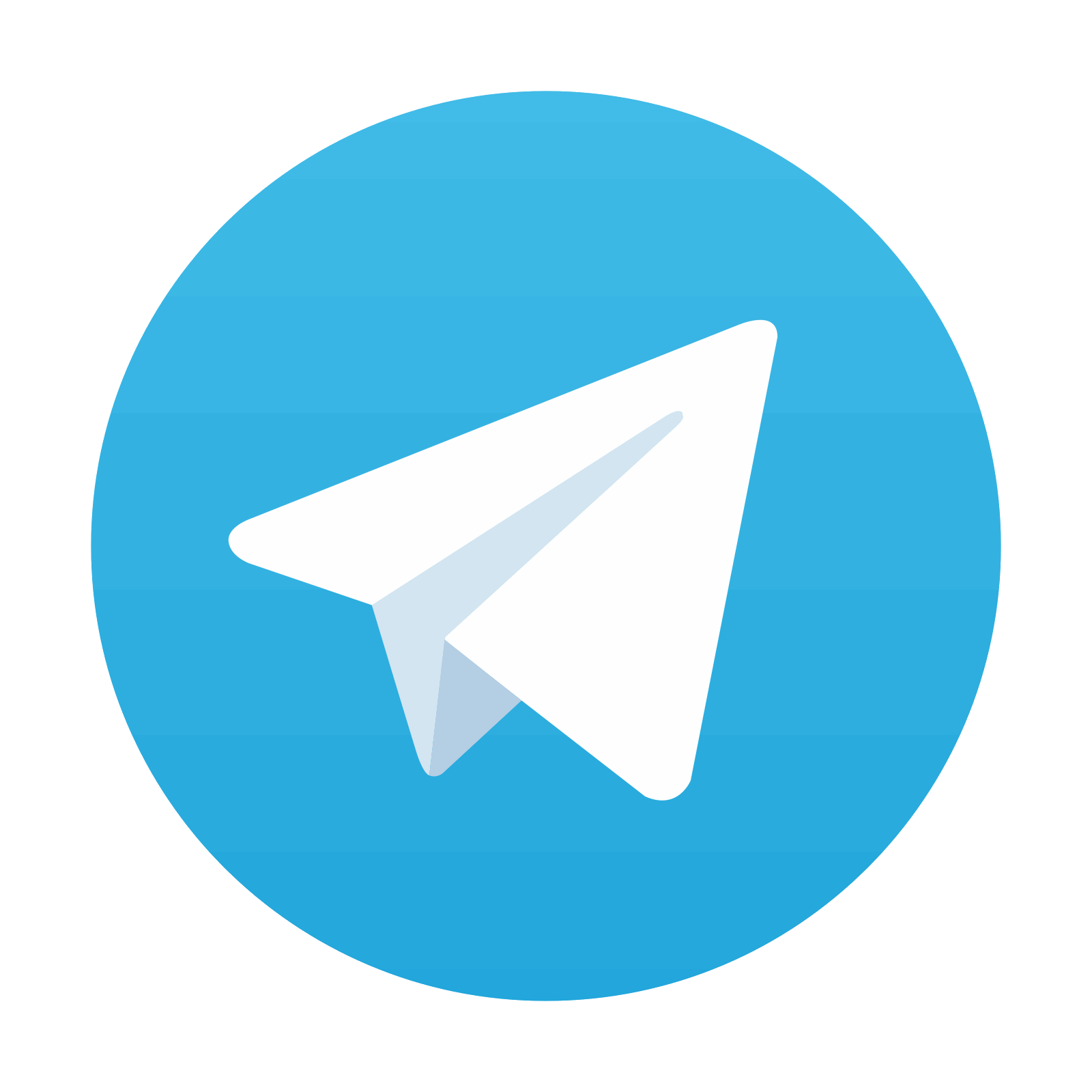
Stay updated, free articles. Join our Telegram channel

Full access? Get Clinical Tree
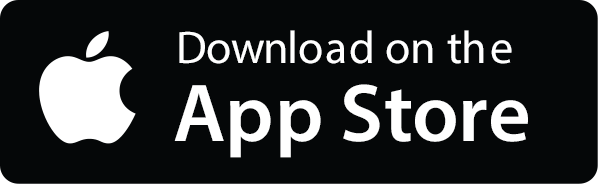
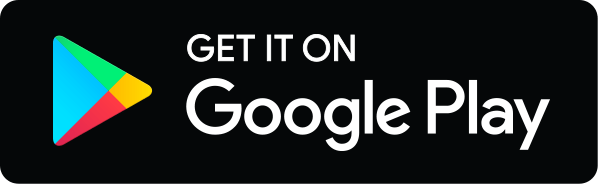