This review summarizes the evolution of trauma resuscitation from a one-size-fits-all approach to one tailored to patient physiology. The most dramatic change is in the management of actively bleeding patients, with a balanced blood product–based resuscitation approach (avoiding crystalloids) and surgery focused on hemorrhage control, not definitive care. When hemostasis has been achieved, definitive resuscitation to restore organ perfusion is initiated. This approach is associated with decreased mortality, reduced duration of stay, improved coagulation profile, and reduced crystalloid/vasopressor use. This article focuses on the tools and methods used for trauma resuscitation in the acute phase of trauma care.
Key points
- •
Future research should inform clinicians on the role of permissive hypovolemia, for how long this should be maintained, and how/if this should be applied to patients with traumatic brain injury.
- •
Our understanding of trauma-induced coagulopathy (TIC) is evolving and may see targeted blood component therapy incorporated early in trauma shock resuscitation.
- •
The role of viscoelastic hemostatic assays in assessing TIC and directing blood component resuscitation requires further study.
- •
There is increasing understanding of endothelial damage as a driver of TIC, raising the possibility of targeting repair to improve hemostasis and reduce organ failure.
- •
More work is required to identify the most appropriate goals for posthemostasis resuscitation balancing the risks of fluid overload and underresuscitation.
Introduction
The 2 leading causes of death after trauma are blood loss and neurologic injury, which account for more than three-quarters of injury related mortality. Fifty percent of early deaths (<24 hours from injury) are due to hemorrhage, with hemorrhagic shock an important driver for postresuscitation organ failure and late mortality. There has been a considerable improvement in our understanding of trauma resuscitation over the past 30 years, particularly in hemorrhage control and trauma resuscitation, seeing outcomes steadily improve. However, blood loss remains a leading cause of preventable death in the initial 24 hours of hospital admission.
This review summarizes the evolution of trauma resuscitation, offering clinicians the knowledge base to enable the highest standards of clinical care. Trauma resuscitation has evolved from a one-size-fits-all approach to one tailored to patient physiology. The most dramatic change is in the management of patients who are actively bleeding, with a balanced blood product–based resuscitation approach (avoiding crystalloids) and surgery focused on hemorrhage control, not definitive care. The key components of this “damage control resuscitation (DCR)” approach are (1) hemorrhage control, (2) permissive hypotension/hypovolemia, and (3) the prevention and correction of trauma-induced coagulopathy (TIC). When hemostasis has been achieved, definitive resuscitation to restore organ perfusion is initiated. This DCR strategy prioritizes TIC, temporarily sacrificing perfusion for haemostasis. This approach is associated with a decrease in mortality, reduced duration of stay in intensive care and hospital, improved coagulation profile, and reduced crystalloid/vasopressor use. There are many areas of trauma resuscitation that remain controversial and subject to ongoing research, such as patients with concomitant traumatic brain injury (TBI), environments with limited access to blood products, and the optimal diagnostic and therapeutic approaches to treating coagulopathy. In this article, we focus on the tools and methods used for trauma resuscitation in the acute phase of trauma care ( Box 1 ).
Permissive hypotension
Early empiric use of red blood cells and clotting products
Limited fluids that may dilute coagulation proteins
Introduction
The 2 leading causes of death after trauma are blood loss and neurologic injury, which account for more than three-quarters of injury related mortality. Fifty percent of early deaths (<24 hours from injury) are due to hemorrhage, with hemorrhagic shock an important driver for postresuscitation organ failure and late mortality. There has been a considerable improvement in our understanding of trauma resuscitation over the past 30 years, particularly in hemorrhage control and trauma resuscitation, seeing outcomes steadily improve. However, blood loss remains a leading cause of preventable death in the initial 24 hours of hospital admission.
This review summarizes the evolution of trauma resuscitation, offering clinicians the knowledge base to enable the highest standards of clinical care. Trauma resuscitation has evolved from a one-size-fits-all approach to one tailored to patient physiology. The most dramatic change is in the management of patients who are actively bleeding, with a balanced blood product–based resuscitation approach (avoiding crystalloids) and surgery focused on hemorrhage control, not definitive care. The key components of this “damage control resuscitation (DCR)” approach are (1) hemorrhage control, (2) permissive hypotension/hypovolemia, and (3) the prevention and correction of trauma-induced coagulopathy (TIC). When hemostasis has been achieved, definitive resuscitation to restore organ perfusion is initiated. This DCR strategy prioritizes TIC, temporarily sacrificing perfusion for haemostasis. This approach is associated with a decrease in mortality, reduced duration of stay in intensive care and hospital, improved coagulation profile, and reduced crystalloid/vasopressor use. There are many areas of trauma resuscitation that remain controversial and subject to ongoing research, such as patients with concomitant traumatic brain injury (TBI), environments with limited access to blood products, and the optimal diagnostic and therapeutic approaches to treating coagulopathy. In this article, we focus on the tools and methods used for trauma resuscitation in the acute phase of trauma care ( Box 1 ).
Permissive hypotension
Early empiric use of red blood cells and clotting products
Limited fluids that may dilute coagulation proteins
Hemorrhage control
The most effective resuscitation fluid is our patients’ own blood; thus, preserving circulating volume and minimizing blood loss is a key component of trauma resuscitation. Strategies include minimal handing, direct pressure on wounds, early and accurate fracture splinting, and rapid surgical or radiologic hemorrhage control.
The management of bleeding patients has 2 aims—to arrest bleeding and to restore blood volume—and is recognized as the single most important step in the management of the severely injured patient. Hemorrhage can be compressed by applying direct pressure to the site or using tourniquets, but there are certain anatomic regions (groin, axilla, neck) that prove difficult for standard tourniquet use. Junctional tourniquets (for injuries in the inguinal and axillary regions) and hemostatic dressings have been developed to control proximal bleeding, but there are few data on their effectiveness, with studies mainly from combat-related case series. A number of different devices and interventions have been developed in an attempt to reduce the morbidity and mortality associated with noncompressible torso hemorrhage, with military campaigns driving forward innovation in this field. Devices such as tourniquets and splints are used alongside interventions, such as resuscitative endovascular balloon occlusion of the aorta to preserve circulating volume until definitive surgical treatment can be undertaken.
The recognition of shock and active bleeding
All patients need to be resuscitated from shock and have normal organ perfusion restored. However, in trauma patients who are actively bleeding, attempts to restore perfusion before achieving hemostasis are counterproductive. Patients who are shocked may have stopped bleeding, whereas patients who are bleeding may not yet be clinically shocked. Because it is the presence or absence of active bleeding that changes the resuscitation strategy, the recognition of ongoing hemorrhage is the key step in the management of trauma patients. Understanding the depth of shock guides ongoing resuscitation.
Recognizing the Actively Bleeding Patient
Homeostasis with peripheral vasoconstriction acts to preserve blood pressure as circulating volume is progressively lost, potentially leading to inadequate cardiac output for organ perfusion even though blood pressure remains within the normal range. Thus, in trauma patients, adequate cardiac output cannot be inferred from blood pressure, and only when blood loss is critical, or rapid, is there a relationship between the two. Patients with overt hypovolemic shock characterized by hypotension, tachycardia, and overt blood loss are readily identified, but trauma patients may be shocked despite normal blood pressure and pulse, termed cryptic shock; this is associated with increased mortality.
The Advanced Trauma Life Support manual and courses have popularized the role of physiologic parameters to estimate and grade the severity of blood loss. However, physiologic data from a large UK database suggests that this approach is not borne out in practice. Data from UK and US databases demonstrate that mortality increases progressively below a systolic blood pressure of 110 mm Hg. A 2011 databank analysis of 902,852 records identified that the optimal systolic blood pressure cutoff for hypotension as a predictor of mortality differed with age: namely, 85 mm Hg for those 18 to 35 years, 96 mm Hg for those 36 to 64 years, and 117 mm Hg for those over 65 years of age.
In the absence of obvious external exsanguination, the identification of patients who are actively bleeding can be very challenging. Only dynamic methods such as fluid responsiveness are of practical utility in this patient group. Classically, patients are categorized according to their response to a fluid bolus into responders, nonresponders, or the intermediate ‘transient responder’ group. Both transient responders and nonresponders are actively bleeding.
The use of an initial fluid bolus to determine responsiveness is itself now controversial. In a patient who has lost 40% of their circulating volume, a bolus of 500 or 1000 mL of crystalloid will lead to a 15% to 30% dilution and worsen existing coagulopathy. Giving 1 to 2 units of red blood cells and fresh frozen plasma as initial bolus will have a similar effect but will potentially use blood products for a large proportion of patients who are not bleeding.
Focus has shifted, therefore, to looking at whether bleeding can be predicted from a patient’s physiology and injury characteristics. Various scoring systems have been developed that aim to predict major hemorrhage or massive transfusion (variably defined). The Assessment of Blood Consumption score is widely used and is derived from penetrating disease, systolic blood pressure in the emergency department, and positive Focused Assessment with Sonography in Trauma ; the Trauma Associated Severe Hemorrhage score is more complex and is based on systolic blood pressure, hemoglobin, intraabdominal fluid on ultrasound/computed tomography scanning, Abbreviated Injury Scale, heart rate, and male gender. These and other tools report sensitivities in the region of 70% to 80% for the prediction of massive transfusion requirements, may induce delays in recognition, and clinician gestalt has been shown to perform marginally below this, with sensitivities of around 65%. Trauma Associated Severe Hemorrhage and Assessment of Blood Consumption scores are excellent at defining which patients will not require massive transfusion. The 2016 UK National Institute for Health and Care Excellence (NICE) guidelines for major trauma reviewed these scoring systems and concluded that there was insufficient evidence of clinical usefulness to support the use of any of these tools to predict massive transfusion. A practical approach to identifying these bleeding patients is therefore to use a combination of the severity of the patient’s physiology and injuries, and response to initial bolus volume resuscitation (ideally given as red blood cells [RBCs]).
Assessing Shock Severity
There are 2 key shock thresholds that determine outcome from hemorrhagic shock. The lower threshold is that coronary artery perfusion must be maintained to preserve cardiac function. The upper threshold is the endpoint of resuscitation from shock, as indicated by normalization of global perfusion parameters.
Coronary artery perfusion is blood pressure dependent and, therefore, this represents the lowest acceptable blood pressure threshold during a trauma resuscitation. Cerebral perfusion is essentially a proxy for maintaining the coronary circulation in this group of patients. Coronary perfusion occurs principally during diastole, and so maintenance of diastolic pressure (25–35 mm Hg) is the key value to observe and maintain. It is difficult to equate this to a specific systolic or mean arterial blood pressure, or a specific palpable pulse. With the narrowed pulse pressure of hemorrhagic shock, it is reasonable to target systolic blood pressures of 60 to 70 mm Hg. Resuscitative endovascular balloon occlusion of the aorta may increase diastolic pressure, so improving coronary artery perfusion for a given circulating volume. NICE guidance recommends maintenance of a palpable central pulse (carotid or femoral) at minimum throughout resuscitation.
At the other end of shock resuscitation is normalization of systemic parameters of organ perfusion, such as measurements of base deficit and lactate. These parameters give an estimation of the severity of shock state and tend to be used to the response to resuscitation (although again the goal before hemorrhage control is not necessarily to normalize these figures). Although there is disagreement as to which is the best acid–base parameter to use, generally in trauma patients without preexisting comorbidities, there is strong correlation between all the standard parameters of lactic acidosis (base deficit or lactate, arterial or venous), and these measures are strong predictors of outcome.
Because these parameters depend on blood draws, they do not provide a continuous assessment of the severity of shock and systemic perfusion. Many technologies have been investigated as a means to continuously monitor the depth of shock. These include near infrared spectrometry, sublingual videomicroscopy, and noninvasive cardiac output monitoring. All have their proponents, benefits, and drawbacks, and all require further study. The most important and routinely available continuous measure of the depth of shock is end-tidal capnography. Studies have shown good correlation between cardiac output and end-tidal capnography in models of hemorrhage and reperfusion. Hemodynamic instability is associated with low end-tidal capnography and low cardiac output in ventilated trauma patients. Decreased delivery of CO 2 and increase in alveolar dead space result in a high ventilation/perfusion ratio, which is reflected in decreased CO 2 output from the lungs in a shocked state. End-tidal capnography should be used in all trauma hemorrhage resuscitations where the patient is intubated and ventilated.
Permissive hypotension and the elimination of crystalloid infusions
Permissive hypotension is conceptually the most difficult part of the DCR paradigm to understand and to implement. DCR temporarily prioritizes hemostasis over perfusion, and permissive hypotension is the means to this end. Resuscitation during active bleeding aims to maintain blood pressure above, but possibly close to, the coronary perfusion threshold as described.
Restoration of a normal blood pressure is not a goal at any point during trauma resuscitation. Although many registry studies have shown that patients with low blood pressures (with increasing mortality below a systolic blood pressure of 110 mm Hg) have worse outcomes, this is principally a reflection of the nature of their injuries. It does not follow that normalization of blood pressure will improve outcomes. In bleeding animals and patients, increasing or attempting to increase blood pressure with fluid amounts greater than that required to maintain basal coronary–cerebral circulation is undesirable, because it increases bleeding from sites of hemorrhage and because the methods we have available to increase blood pressure are ultimately harmful in this group of patients. Giving fluid infusions (as crystalloid or colloid) causes dilutional coagulopathy, anemia, endothelial damage, and tissue edema, all of which are associated with poor outcomes. Even volume administered with RBCs or balanced blood product resuscitation will induce some degree of dilutional coagulopathy. High-volume fluid resuscitation may increase trauma-related bleeding, organ failure, and mortality.
The experimental evidence for permissive hypotension is compelling, but the majority of studies were conducted before balanced blood product–based resuscitation was available. There are no animal or human studies of uncontrolled hemorrhage that show high-volume resuscitation regimens lead to improved outcomes. A 2014 metaanalysis of liberal versus restricted fluid resuscitation for victims of trauma shock included prehospital and in-hospital studies, blunt and penetrating disease, and studies targeting specific volume of fluid and/or specific blood pressures. The authors included 4 randomized, controlled trials, reporting no overall mortality difference between liberal and restrictive fluid strategies (relative risk, 1.18; 95% confidence interval [CI], 0.98–1.42). However, when the Turner study was excluded because it involved a very high level of protocol violations then patients treated with liberal fluid resuscitation strategies had a higher mortality (relative risk, 1.25; 95% CI, 1.01–1.55; I , 0%). The proportion of patients with penetrating trauma was higher than is observed in most clinicians’ practice and patients with TBI were predominantly excluded. A recent pilot randomized, controlled trial has explored prehospital and emergency department resuscitation using crystalloid targeting either a systolic pressure of greater than 70 or 110 mm Hg. There was a decrease in mortality at 24 hours in the lower pressure group for blunt trauma with significant differences in the infused crystalloid volume (1 vs 2 L), but the numbers of deaths were small and a larger trial is warranted.
Clinical studies of permissive hypotension are difficult, and reflect the challenges of identifying and managing these patients in daily practice. All human trials are remarkably consistent in findings that, (1) in practice it is impossible to achieve a set blood pressure target in bleeding patients, and (2) attempts to reach and maintain these targets only leads to increased fluid resuscitation without any improvement in mortality outcomes. However, the 2011 US recommendations for military resuscitation and the UK 2016 NICE guideline on major trauma recommend fluid administration be titrated to a palpable carotid or femoral pulse or systolic pressures of 80 to 85 mm Hg. In practice, such targets are rarely achievable.
Vasopressors in Early Trauma Shock Resuscitation
The sympathetic response to trauma and blood loss shifts the blood from the unstressed to stressed circulation (mainly from the splanchnic circulation) enabling preservation of blood pressure in the face of significant blood losses. However, vasoplegia occurs rapidly in shock as a result of cytokine release, nitric oxide production; tissue, cellular, and mitochondrial damage; hypoxia; and reperfusion injury. This finding offers a potential theoretic framework to administer vasopressors during trauma resuscitation and has been hypothesized to reduce blood loss and provide early hemodynamic stability, thus buying time to definitive surgical haemostasis. However, inopressors have a wide range of potential adverse effects, including arrhythmia, increased cardiac work, increased afterload, reduced tissue perfusion, and increasing blood loss as a result of induced increases in blood pressure, with evidence for harm when applied in the initial phase; indeed, adrenergic stress is associated with numerous adverse effects in critical illness.
Animal work suggests a potential role for vasopressin, alone or in combination with noradrenaline, with some work suggesting improved tissue oxygenation combined with a DCR approach and early blood products. Norepinephrine alone has shown mixed results.
Given the common use of vasopressors in trauma care and their support in the European guidelines for hemorrhagic shock, there are few human data. Vasopressors are commonly used to improve cerebral perfusion. Arginine vasopressin release occurs early in major blood loss and assists in centralizing the remaining circulating volume to the brain, heart, and lungs. However, stores are depleted rapidly. Limited data, including 1 small randomized, controlled trial, suggest that vasopressin may improve outcomes if combined with rapid hemorrhage control and improve blood pressure while not worsening blood loss.
However, 3 large observational studies show an association between vasopressin (55% vs 41%; P = .02) and vasopressor (noradrenaline/norepinephrine, vasopressin, dopamine, phenylephrine) to be associated independently with higher mortality. A systematic review of vasopressor use in early trauma resuscitation including 6 studies identified an increase in mortality associated with vasopressor use in all five included observational studies (all with high risk of bias) and an underpowered randomized, controlled trial. It remains unclear if vasopressor use is causative of poor outcomes or a marker of severity of illness. The role for vasopressin is currently being evaluated in the VITRIS.at trial (Vasopressin in Traumatic Hemorrhagic Shock Study; NCT00379522 ; EudraCT number 2006-004252-20).
Currently, there is insufficient evidence to define the place of vasopressors in early trauma resuscitation. In our practice, vasopressors have no role in the initial resuscitation phase, because we find we can maintain blood pressures above coronary artery perfusion pressure with blood products and early surgical intervention, where required.
Resuscitation in Patients with Traumatic Brain Injury
The permissive hypotension approach is most conflicted in patients who are bleeding and have a major TBI. Data from patients with isolated TBI shows that those who experience episodes of hypotension have worse outcomes and guidelines advocate maintaining a systolic blood pressure of greater than 100/110 mm Hg to preserve cerebral perfusion. Bleeding trauma patients who have TBI do worse than those without TBI. However, neither of these facts directly support an approach that sets higher blood pressure targets in bleeding patients with TBI. Experimental evidence suggests that, in the setting of combined hemorrhage and brain injury, high-dose crystalloid resuscitation to target higher blood pressures is associated with increased cerebral edema, increased intracranial pressure, decreased cerebral perfusion pressure, and reduced cerebral oxygen delivery with the cerebral circulation protected in trauma shock. Human data in this area are almost completely absent, in part because this represents a small group of patients with generally very poor outcomes, and studies on permissive hypotension have excluded patients with TBI. The UK NICE major trauma guideline suggests that the clinician should focus resuscitation on the predominant injury complex, so a restrictive fluid administration is advocated where bleeding is the dominant pathology being treated.
High-volume crystalloid resuscitation is associated with endothelial damage, tissue edema, organ failure, dilutional coagulopathy, impaired clot formation, dilutional anemia, and increased bleeding, all of which are associated with poor outcomes. Over the past decade, as the DCR approach has become more prevalent, multiple retrospective studies of bleeding patients have shown a decrease in crystalloid use associated with decreased, organ failure, decreased abdominal compartment syndrome, and decreased mortality. The presence of acute lung injury and abdominal compartment syndrome in particular are strongly associated with worse TBI outcomes. The presence or absence of TBI is unknown for most patients in the prehospital and early in-hospital phase of care. The concomitant TBI population has not been examined specifically in retrospective studies, but to date there is no compelling evidence that patients with possible TBI should be treated according to a different management paradigm than those who do not. In trauma patients, where hemorrhagic shock seems to be the dominant pathophysiology, a DCR approach with permissive hypotension should be used. Where brain injury seems to be the dominant pathology, then blood pressure targets should be targeted toward the maintenance of cerebral perfusion pressure.
Conclusions: Permissive Hypotension
Several questions remain around the application of permissive hypotension in contemporary trauma care. All experimental and the majority of clinical studies have been conducted in the context of crystalloid-first resuscitation, and whether the plasma or balanced transfusion regimens reduce the requirement for permissive hypotension is yet to be determined. Methods for continuous monitoring and ensuring coronary and cerebral perfusion would allow a personalized approach and remove many of the unknowns from the permissive approach to blood pressure management.
Permissive hypotension has become a central component of the DCR approach. It must be understood as a passive process that is temporarily tolerated until hemorrhage control is achieved. Basal coronary–cerebral circulation is maintained by avoiding crystalloids and the judicious use of balanced blood product transfusions. At all times, hemorrhage control is the goal, not normalization of blood pressure.
Prevention and correction of trauma-induced coagulopathy
DCR aims to preserve hemostatic function, reduce blood loss, and directly address TIC, and is associated with improved outcomes. The mechanisms by which this is achieved and detailed pathophysiology of TIC are yet to be fully elucidated but are the focus of investigation for multiple international trauma research consortia (Targeted Action for the Cure of Trauma-Induced Coagulopathy [Euro TACTIC, http://www.tacticgroup.dk/ ], Trans-Agency Consortium for Trauma-Induced Coagulopathy [US TACTIC, http://www.tacticproject.org/ ] and Research Outcomes Consortium [ROC] [ https://roc.uwctc.org/tiki/tiki-index.php?page=roc-public-home ]).
Pathophysiology of Trauma-Induced Coagulopathy
TIC is a multifactorial failure of the coagulation system to sustain adequate hemostasis after major trauma hemorrhage. The 2 principle processes are an endogenous coagulopathy induced by the injury and shock state, and a dilutional coagulopathy caused by volume resuscitation. The endogenous coagulopathy (acute traumatic coagulopathy [ATC]) is present almost immediately in severely shocked and injured trauma patients and can be detected in the prehospital phase before large volume resuscitation. Resuscitation-induced coagulopathy augments this process and may dominate later in the clinical course. At physiologic extremes, hypothermia and acidemia will also exacerbate the coagulopathy. Around 25% of critically injured trauma patients are coagulopathic on arrival, increasing to close to 100% after receiving a massive transfusion. Once TIC is established, it is a poor prognostic indicator, with greater transfusion requirements, higher levels of organ dysfunction, and a 4-fold increase in mortality.
Although there seem to be different patterns of the endogenous ATC, it is characterized by the delayed formation of weak clots, increased clot breakdown, and with only minor prolongation of clotting times. Loss or consumption of procoagulant factors (such as factors VII or X) is not the prime driver of ATC. Rather, there is loss or breakdown of fibrinogen and a systemic hyperfibrinolysis owing to increased plasmin activity. ATC also seems to cause platelet dysfunction, with reduced aggregation in response to all standard activators, although platelet count is typically maintained after trauma. The changes seem to be driven by the combination of injury and shock, and may be mediated through endothelial dysfunction and glycocalyx degradation.
Resuscitation coagulopathy complicates ATC by adding in a progressive reduction in procoagulant factors. Crystalloid and colloid infusions will rapidly dilute circulating coagulation factors, especially when circulating blood volume is already low. RBC alone will also cause this dilutional effect. Even the recommended “balanced” transfusion of 1:1:1 units of red cell, plasma, and platelets only approaches the normal clotting factor concentrations of whole blood. These transfusion products also have reduced functionality owing to processing and storage. This reduction in circulating clotting factors is compounded by further endothelial injury caused by crystalloid transfusions, which likely further exacerbates TIC.
Patients, therefore, present with an established endogenous coagulopathy, which is exacerbated by resuscitation in the face of ongoing bleeding. DCR focuses on maintaining the hemostatic potential of blood, by minimizing any resuscitation-induced coagulopathy and correcting any existing coagulopathy.
Treatment of trauma-induced coagulopathy
Treatment of Endogenous Acute Traumatic Coagulopathy: Tranexamic Acid
At present, the only specific recommended treatment for ATC is the administration of the antifibrinolytic tranexamic acid (TXA). Military and civilian studies, including the large CRASH-2 trial (Clinical Randomisation of an Antifibrinolytic in Significant Haemorrhage), have shown that administration of TXA to bleeding trauma patients reduces overall mortality. Treatment with TXA is usually recommended empirically because there are questions about the sensitivity of diagnostic tests to identify hyperfibrinolysis in trauma patients.
There are multiple unanswered questions regarding the use of TXA in trauma. It is unknown which patient groups benefit from TXA. CRASH-2 was conducted in all patients suspected of active bleeding, and therefore included a large number of patients who were not bleeding. A subgroup analysis in CRASH-2 found the greatest survival benefit to be in those patients with shock, and was confirmed in a retrospective civilian study. In combat casualties, empiric use of TXA showed the greatest benefit observed in patients who subsequently required a massive transfusion. Given that ATC is driven by the presence of shock, it would seem biologically consistent to administer TXA to those patients who are in shock or who are bleeding and likely to develop shock.
Other questions include the optimal dose and timing of TXA, as well as its mechanism of effect. TXA seems to reduced bleeding-related deaths without affecting transfusion requirements. It also may worsen outcomes if given more than 3 hours after an injury, which may reflect a dynamic change in coagulation profiles of trauma patients. Further mechanistic studies and clinical trials of TXA are ongoing.
Prevention of Resuscitation Coagulopathy
The development of a resuscitation coagulopathy is prevented or reduced by using a balanced transfusion regimen that approaches that of whole blood. In practice, this means providing an equal ratio of RBCs, plasma, and platelet transfusions (note that some of these products may be pooled in some countries).
There have been many retrospective studies showing the potential benefit of this approach over RBC transfusions alone, catchup strategies, or the use of plasma ratios. The PROPPR randomized, controlled trial (Pragmatic, Randomized Optimal Platelet and Plasma Ratios) examined the effectiveness and safety of a 1:1:1 transfusion ratio (fresh frozen plasma:platelets:RBCs) compared with a 1:1:2 ratio in patients with trauma who were predicted to receive a massive transfusion. Overall, there was no significant difference between groups in either 24-hour or 28-day mortality, but deaths from exsanguination were reduced in the 1:1:1 group, with greater numbers achieving anatomic hemostasis and a consistent trend toward improved overall survival. Although there are many further questions raised by these results, this is likely to remain the best quality evidence in this area. Most recent guidelines suggest that a 1:1:1 strategy is started immediately and used throughout DCR.
Major Hemorrhage Protocols
Massive hemorrhage protocols (MHPs) are central to DCR and designed to specifically target ATC. Protocolized transfusion avoids dilutional coagulopathy through empiric provision of RBCs with simultaneous, high-dose clotting products (plasma, cryoprecipitate or fibrinogen concentrate, and platelets). To enable rapid delivery of a 1:1:1 strategy, MHPs are activated solely based on physiology and suspected active hemorrhage rather a formal diagnosis of coagulopathy by laboratory or point-of-care assays. Volume resuscitation with RBCs and clotting products from the outset avoids the need for crystalloids and colloids, with blood banks providing a continuous supply of “transfusion packs” until hemorrhage control is achieved (through abbreviated surgery or interventional radiology).
Preventing dilutional coagulopathy and avoiding crystalloids from the start has implications for the prehospital care of these patients. Following experience gained in recent military conflicts with prehospital use of RBC and fresh frozen plasma, a number of civilian services are beginning to use blood products at the scene of the injury. However, data supporting the prehospital use of RBC and fresh frozen plasma are all retrospective and the results are conflicted. Several randomized, controlled trials are underway evaluating the use of blood products as first-line resuscitation in the prehospital phase of care. The REPHILL trial (Resuscitation with Pre-HospItaL bLood products; https://www.clinicaltrialsregister.eu/ctr-search/trial/2015-001401-13/GB ) compares RBC and lyophilized plasma (LyoPlas N-w) against standard of care (crystalloid) and the COMBAT trial (Control of Major Bleeding After Trauma Study; https://clinicaltrials.gov/ct2/show/NCT01838863 ) explores the role of initial resuscitation using plasma as compared with normal saline.
Correction of Trauma-Induced Coagulopathy
Despite early administration of TXA and volume resuscitation with balanced transfusion, patients will still become coagulopathic as they continue to bleed. Different patterns of coagulopathy will develop depending on the nature of the injuries, severity and duration of shock, and the nature and timing of fluids and blood products patients have received. Although most coagulation therapy is empiric, it is important to monitor coagulation status throughout bleeding to ensure that hemostatic competence is maintained. Point-of-care coagulation monitoring may allow for a more precision approach to the management of TIC during DCR.
Laboratory and Point-of-Care Diagnostics
Traditional monitoring of coagulopathy during hemorrhage has relied on standard laboratory tests of coagulation such as prothrombin time, partial thromboplastin time, platelet count, and fibrinogen levels. These are of limited value in trauma hemorrhage owing to the time delays in laboratory processing and the lack of sensitivity of prothrombin time, partial thromboplastin time, and platelet count for the underlying pathophysiology of TIC. Of the laboratory tests, the fibrinogen level is the most valuable. Fibrinogen is affected by both ATC and resuscitation, and levels can rapidly become dangerously low. Although some MHPs routinely supplement fibrinogen with cryoprecipitate or fibrinogen concentrate, the doses used are rarely sufficient to maintain levels or replace losses. Fibrinogen levels should be taken regularly during DCR and sample processing prioritized by the laboratory.
Point-of-care prothrombin time assays have been appraised in trauma but have been found to have limited accuracy. The viscoelastic hemostatic assays (VHAs), namely, thromboelastography (Haemoscope Corp, Niles, IL) and rotational thromboelastometry (Pentapharm GmbH, Munich, Germany) provide more specific information related to the clot strength and lysis aspects of TIC, but have yet to be fully evaluated in trauma patients. VHAs are capable of rapidly diagnosing ATC and, importantly, are able to rapidly assess the need for fibrinogen supplementation. The lack of data-driven, trauma-specific algorithms has hampered inclusion of VHAs into standard practice and guidelines ; however, their use is supported by European guidelines for major hemorrhage management. Many European trauma centers and an increasing number of high-volume centers in the UK and the United States are using rotational thromboelastometry/thromboelastography to diagnose and initiate coagulation therapy. An international randomized controlled trial (ITACTIC [Implementing Treatment Algorithms for the Correction of Trauma Induced Coagulopathy], NCT02593877 ) is currently ongoing to compare empiric MHPs with MHP plus VHAs in trauma resuscitation.
Fibrinogen Replacement
During major hemorrhage, fibrinogen is depleted and not restored to normal levels despite MHPs providing balanced 1:1:1 transfusions therapy. Because fibrin is a critical component of clot, maintaining adequate fibrinogen levels is a central goal of coagulation management. Fibrinogen levels should be maintained at greater than 2 g/L during DCR. There are 2 main sources of fibrinogen replacement: cryoprecipitate and fibrinogen concentrate. Two observational civilian cohort trauma studies have reported a decrease in mortality in patients receiving higher fibrinogen content during major transfusion therapy, data that are mirrored by military cohort studies. If fibrinogen replacement is incorporated into MHPs it is usually relatively late in the transfusion sequence and at a relatively low dose. A small pilot study of early high-dose cryoprecipitate supplementation (CRYOSTAT [Early Cryoprecipitate in Major Trauma Haemorrhage]) showed it was possible to rapidly restore fibrinogen levels with a trend toward improved survival. The phase III CRYOSTAT-2 trial of 1544 patients is due to commence enrolling in mid-2017. Fibrinogen concentrate may offer an alternative to cryoprecipitate, but it is expensive and evidence to support its efficacy is lacking. Feasibility studies have shown that fibrinogen concentrate can be administered rapidly to bleeding trauma patients in both in-hospital and prehospital environments with improvements in functional fibrinogen (rotational thromboelastometry) and plasma fibrinogen levels with further studies (eg, FEISTY and EFIT-1, NCT02745041 ) ongoing. Fibrinogen loss is central to the pathophysiology of TIC, fibrinogen levels must be assayed regularly during DCR, and hypofibrinogenemia must be corrected rapidly.
TIC is a multifactorial, described by varying degrees of dysfibrinogenemia, hyperfibrinolysis, endothelial dysfunction, and impaired platelet activity. Early endogenous processes that drive the aberrant response in the coagulation system depend on the magnitude of trauma, and the severity of hemorrhagic shock. DCR and the use of MHPs are associated with improved outcomes, although the mechanisms of how it corrects TIC have yet to be characterized fully. Optimizing TIC treatment to improve outcomes requires further research to understand the dynamic changes in the equilibrium between procoagulant and anticoagulant factors, fibrinogen use, downstream effectors (eg, fibrinolysis), and the individual response to transfusion therapy.
Temperature and Electrolytes
Calcium is a vital cofactor for procoagulant clotting proteases and levels decrease rapidly during massive bleeding, in part owing to chelation after transfusion of citrate-containing blood products during DCR. Low ionized calcium is associated with increased mortality and greater transfusion requirements. Levels should be checked regularly during resuscitation and replaced to maintain levels within the normal range.
Numerous studies report an independent association between hypothermia and death, with effects significant at a body temperature of less than 35°C. The early use of warming blankets and aggressive rewarming on arrival at the hospital may reduce morbidity and mortality. A 2016 European multicenter retrospective study focusing on 564 severely injured patients reported that mild hypothermia did not alter transfusion rates, but a German study involving 15,895 patients found that hypothermia increased the relative risk for transfusion by 2, and increased the duration of stay as well as mortality. Thus, maintaining normocalcemia and normothermia in early resuscitation are key aspects of trauma resuscitations.
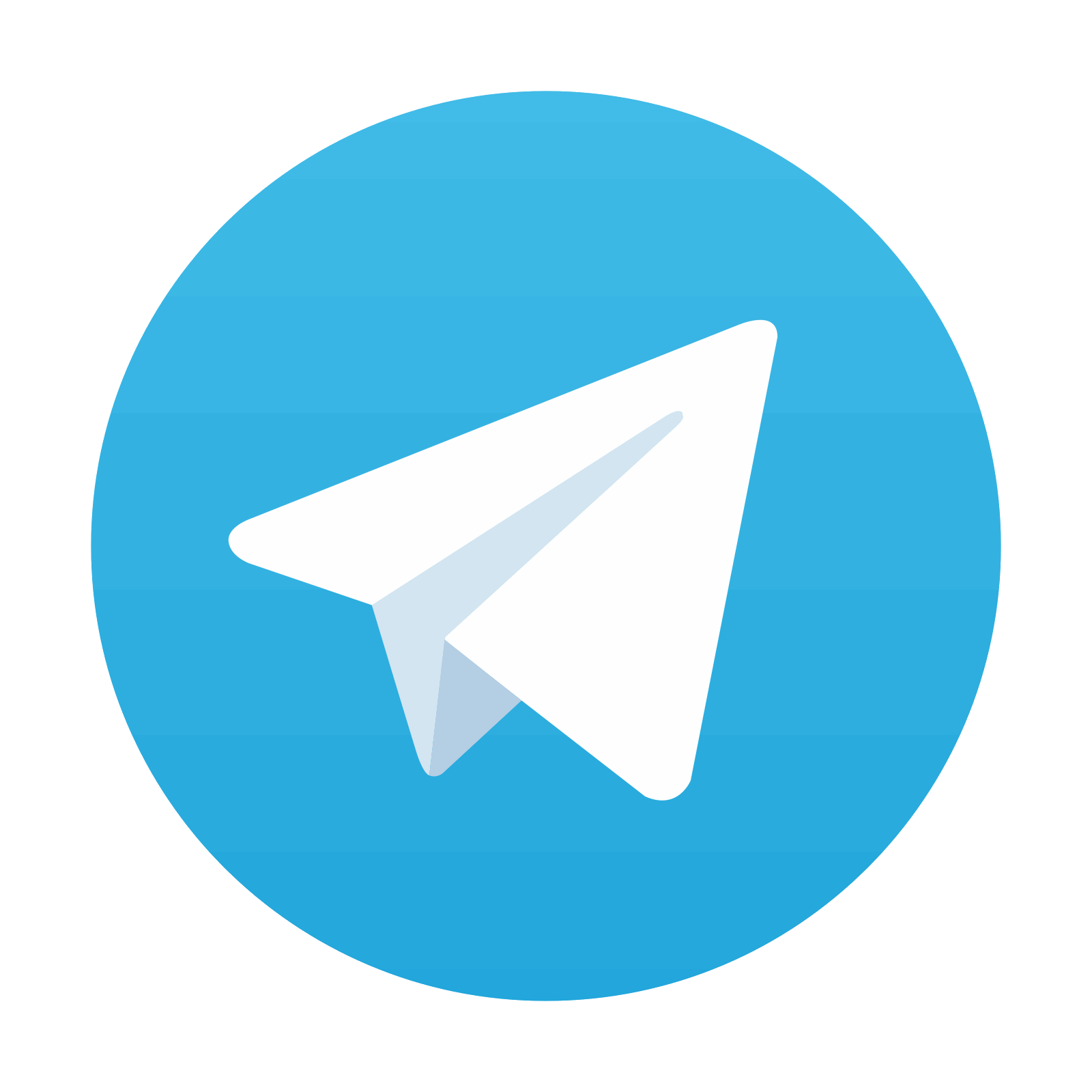
Stay updated, free articles. Join our Telegram channel

Full access? Get Clinical Tree
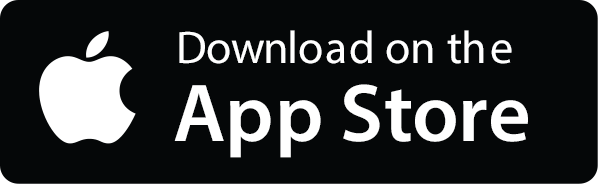
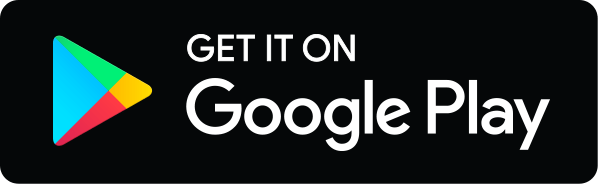