TOPICS
1. The patient with impaired systolic and diastolic ventricular function
3. The diabetic patient and perioperative glucose control
4. The vascular disease patient in need of cardiac surgery
5. The renal failure patient and cardiac surgery
The elective patient for cardiac anesthesia and surgery free of other disease processes is increasingly a rara avis. Prior to advances in percutaneous interventions, the routine cardiac surgery patient was an otherwise healthy middle-aged man in need of a one to two vessel coronary artery bypass—How times have changed. Today’s cardiac surgery patient is likely to be quite elderly with multiple medical problems presenting for combined revascularization and valvular replacement surgery. Moreover, many patients will have had over the course of their lives other cardiac procedures including previous operations and/or percutaneous interventions. Further complicating matters, many of these patients suffer from systolic and diastolic dysfunction, some with remarkably low ejection fractions to less than 20%. Consequently, the “healthy” patient for heart surgery is an oxymoron.
THE PATIENT WITH IMPAIRED SYSTOLIC AND DIASTOLIC VENTRICULAR FUNCTION
Systolic Dysfunction
In past decades, patients presented for cardiac surgery in need of one to two vessel bypass grafts. Usually, their chief complaint was angina and they had no or minimal myocardial damage. Patients with ejection fractions (EFs) of greater than 60% were the rule and not the exception.
Such patients tended to tolerate the peri-induction period well and were readily separated from cardiopulmonary bypass (CPB) with little need for pharmacological or mechanical support. Ventricular function tended to be preserved periopertively assuming acceptable myocardial preservation and surgical techniques.
Today’s cardiac surgical patient is far more challenging. Patients are older with varying degrees of systolic and diastolic dysfunction frequently presenting in congestive heart failure.
Congestive heart failure affects more than 5 million Americans with coronary artery disease as a primary etiology.1 Cardiac surgical outcomes are worse in patients with previous episodes of congestive heart failure, chronic obstructive pulmonary disease, increased age, and peripheral vascular disease (Figure 5–1).
However, preserved right ventricular function in the setting of a severely compromised left ventricle (EF < 25%) may improve perioperative outcomes2(Figure 5–2). Impaired LV diastolic function produces an increase in left ventricular end-diastolic pressure (LVEDP) that is transmitted to the pulmonary circulation. A patient whose right ventricle functions well, tolerates any perioperative worsening in LV diastolic dysfunction and has a potentially better surgical outcome. Consequently, if the right heart is working the patient with severe LV dysfunction will tolerate better surgical revascularization than a patient with biventricular failure.
What is certain is that long-term survival in the heart failure (HF) population is low compared with patients with preserved function3 (Figure 5–3). Operative mortality (< 30 d postoperative) in patients with EF < 30% undergoing surgical revascularization has been reported as high as 4.6% compared with 1.9% in patients with preserved ventricular function.3 However, low EF patients treated with surgical revascularization have a better long-term survival than those treated medically.3 Thus, although immediate short-term morbidity and mortality is high compared to patients with normal ventricular function, surgical revascularization may offer improved survival over the long-term when compared to low EF patients treated medically. Consequently, an ever-increasing number of patients with compromised systolic left ventricular function may become candidates for surgical revascularization.
Heart failure (HF) in cardiac surgical patients may be secondary to ischemia, valvular heart disease, infectious agents, or a myriad of cardiomyopathies.4 Measurements of brain natriuretic peptide (BNP) are routinely used to identify the heart failure patient. A BNP concentration of > 500 pg/mL is indicative of heart failure. BNP produces arterial and venous vasodilatation as well as diuresis. Recombinant BNP (nesiritide) is employed to manage acute episodes of congestive heart failure. Unlike inotropes, it does not affect heart rate or myocardial contractility but unloads the failing heart.
In response to ventricular failure the body attempts to compensate for failing LV systolic function through the sympathetic and the renin-angiotensin-aldosterone systems. Consequently, the HF patient experiences salt retention, volume expansion, sympathetic stimulation, and vasoconstriction.4 The heart dilates to maintain stroke volume (SV) in spite of decreased contractility. Augmented sympathetic tone preserves blood pressure. However, these compensatory mechanisms ultimately fail and contribute to many of the symptoms associated with HF including fluid overload, peripheral and pulmonary edema, tachycardia, and decreased organ perfusion. Ventricular remodeling of the failing heart is the result of multiple factors which produce a dilated, dysfunctional LV. The benefit in the use of angiotensin converting enzyme inhibitors (ACE-I) in HF patients is secondary to their vasodilatory properties and inhibition of ventricular remodeling. However, ACE-I can exacerbate hypotension upon anesthetic induction. Angiotensin receptor blockers (ARBs) are also employed as an alternative to ACE inhibitors with similar potential to lead to peri-induction hypotension. Aldosterone inhibitors when used may be associated with hyperkalemia.
Figure 5–1. In a retrospective analysis of 525 patients with ejection fractions less than 25% long-term outcomes were identified in these Kaplan-Meier survival curves. Increasing age (A), presence of peripheral vascular disease (B), emergent nature of surgery (C), presence of chronic obstructive pulmonary disease (D), and previous episodes of pulmonary congestion (E) all predict a poorer long-term outcome following coronary artery bypass surgery. (With permission from: DeRose J, Tompoulis I, Balaram S, et al. Preoperative prediction of long-term survival after coronary artery bypass grafting in patients with low left ventricular ejection fraction. J Thorac Cardiovasc Surg. 2005;129:314-321.)
Figure 5–2. Survival of patients with poor left ventricular systolic function (EF < 25%) is influenced by right ventricular function as estimated by TEE measures of right ventricular fractional area contraction (RVFAC). Patients with normal right function (RVFAC 35%) had superior short- and long-term survival following coronary artery bypass surgery. (From: Maslow AD, Regan M, Panzica P, et al. Precardiopulmonary bypass right ventricular function is associated with poor outcome after coronary artery bypass grafting in patients with severe left ventricular systolic dysfunction. Anesth Analg. 2002;95:1507-1518, with permission.)
The patient with LV systolic dysfunction and low EF can present to anesthesiologists either with acute or with chronic heart failure. The acute heart failure patient may appear with a greatly reduced EF following a recent myocardial infarction. Other patients will present for surgical revascularization with long-standing ischemic cardiomyopathy. Chronic HF patients usually have undergone numerous PCIs or perhaps previous cardiac surgery interventions. Preoperative evaluation should focus on the review of catheterization and echocardiography reports. Knowledge of baseline left and right heart function is paramount. Pulmonary arterial pressures will often give clues as to the extent of LV dysfunction and provide insight into the pressure load against which the right heart must work to deliver the SV to the LV. Often the patient with a low EF and/or with active myocardial ischemia will have had placed an intra-aortic balloon pump (IABP)5 to support them until the time of surgical intervention.
Figure 5–3. 7841 patients were studied between 1996 and 2001 who underwent coronary artery bypass surgery in Canada. Patients were divided into three groups: lo EF (EF < 30%), med EF (EF between 30% and 50%), and Nl EF (EF > 50%). Patients with low ejection fractions had significantly reduced long-term survival following bypass surgery compared with those with preserved function. (From: Appoo J, Norris C, Merali S, et al. Long-term outcome of isolated coronary artery bypass surgery in patients with severe left ventricular dysfunction. Circulation. 2004;110(suppl II):II-13-II-17, with permission.)
The IABP is placed through the femoral artery into the descending thoracic aorta just below the take off of the left subclavian artery (Figure 5–4, Video 5–1). The IABP provides counter-pulsation to assist the heart in its pumping function. The IABP requires a working heart capable of producing a cardiac output to be effective—it is not to be confused with other mechanical supports for ventricular function that more or less assume the pumping duty of the ventricle. The IABP works opposite to the beating of the heart. When the heart contracts during systole the IABP deflates assisting in drawing blood from the LV into the aorta. When the heart relaxes during diastole and the aortic valve is closed, the IABP inflates increasing diastolic blood pressure and improving coronary perfusion pressure (CPP). Timing of IABP inflation and deflation is accomplished by a triggering mechanism, which can be synchronized to the heart’s cycle using either the ECG, or through the arterial pressure wave. The patient with low EF and myocardial ischemia benefits from IABP placement because the increased diastolic pressure can improve coronary perfusion improving myocardial oxygen supply. Additionally, by reducing afterload in systole, LV wall tension is decreased, reducing myocardial oxygen demand. IABP placement is contraindicated in patients with moderate to severe aortic insufficiency (inflation during diastole would increase regurgitant flow across the aortic valve), aortic aneurysms, and in patients with severe peripheral vascular disease. In most cardiac surgery teams the perfusionist manages the IABP. The IABP can be adjusted to provide various levels of assistance to the heart. It can be triggered with every heartbeat or with every 1:2 or 1:4 beats. Likewise, the degree to which the balloon is inflated can be adjusted on the control panel. The IABP produces an easily recognizable arterial pressure waveform with increased diastolic pressure. Correct timing of balloon inflation and deflation is necessary to maximize the benefit of IABP placement to the patient. IABPs are not risk free and can produce vascular injury, thrombosis, and leg ischemia.
Figure 5–4. An intra-aortic balloon pump (IABP) is positioned distal to the subclavian artery in the descending thoracic aorta. The balloon inflates during diastole. The timing of IABP inflation is adjusted to maximally augment the diastolic pressure. The insets show a normal arterial pressure wave form with the IABP deflated during 1:1 ballooning. During diastole the balloon inflates producing the characteristic “M”-shaped arterial pressure waveform seen here.
Patients with low EF are also frequently treated with implantable cardioverter-defibrillators (ICDs) and cardiac resynchronization therapy (CRT) to both improve ventricular performance and mitigate the effects of any life-threatening dysrhythmias. The ICD is placed in the therapy—off mode during most surgical procedures including cardiac surgery so as not to trigger aberrantly during surgery. External defibrillation pads are placed when the ICD defibrillation function is turned off. These patients require continuous monitoring throughout the perioperative period until ICD function is restored and the device reprogrammed.
There are no anesthetic agents, which offer specific benefits in the induction and maintenance of patients with systolic left heart failure. Various combinations of anesthetic drugs can be used effectively with attention focused on how best to minimize hemodynamic instability in patients with limited cardiac reserve. Whatever agents employed, all can/will impact the three determinants of stroke volume (SV): the volume delivered to the LV to eject (preload), the contractility of the LV, and the resistance against which the LV must work to deliver the SV into the systemic circulation (afterload). The patient with preserved cardiac function has the reserve to respond to a decrease in loading conditions that routinely accompany anesthesia induction. In the patient with coronary artery disease (CAD) and preserved ventricular function the main peri-induction task of the anesthesiologist is to avoid myocardial ischemia. Tachycardia and reduced CPP in the CAD patient can lead to myocardial ischemia as heralded by ST-segment changes and segmental wall motion abnormalities on TEE. However, if the anesthesiologist is able to maintain coronary perfusion and keep the balance between myocardial oxygen supply and demand in balance, the induction and maintenance of anesthesia can be achieved with very little excitement. In contrast, the systolic HF patient has limited ability to increase myocardial contractility in response to changed loading conditions. The loss of sympathetic tone, institution of positive pressure ventilation, and vasodilatation can lead to hemodynamic collapse. In a patient with chronic compensated heart failure, the enlarged heart contracts and ejects enough of a SV to generate a blood pressure and a cardiac output to meet the patient’s metabolic needs.
Recall,
BP = CO × SVR, where CO = SV × HR
However, when the loading conditions of the heart are reduced, and compensatory mechanisms are impaired the blood pressure collapses with anesthetic induction. This reduction in blood pressure then decreases CPP leading to myocardial ischemia further worsening cardiac dysfunction. Anesthetic induction in the HF patient can lead to hemodynamic collapse resulting in ventricular fibrillation and the need for the immediate institution of cardiopulmonary bypass.
Therefore, induction of anesthesia in the HF patient can be stressful for patient and anesthesiologist alike. Plans should be made in advance for the institution of emergency CPB. Both the surgeon and the perfusionist should be closely at hand during anesthesia induction and the bypass circuit should be primed. The placement of an IABP prior to anesthetic induction can be useful in the patient with severe failure and/or myocardial ischemia.
Any anesthetic agent can be employed as long as its impact upon the loading conditions of the heart is managed. Large venous catheters should be in place in order to assist in volume delivery. Since IABPs carry their own risks including limb ischemia and embolization, they are not always placed in patients with borderline ventricular function. In such patients a femoral arterial catheter can be placed before induction for systemic blood pressure monitoring. Should the patient deteriorate, that line can be rewired to permit IABP placement. Additionally, femoral arterial line monitoring provides a more central invasive blood pressure measurement than that obtained by a radial arterial catheter.
Following induction, management can be greatly aided by placement of the TEE probe. TEE can be employed to determine both the cause and etiology of hypotension following induction. The patient with systolic dysfunction presents with a dilated and poorly contractile left ventricle. With anesthesia induction the poorly contracting LV cannot increase stroke volume. Systemic blood pressure decreases along with systemic vascular resistance. Hemodynamic collapse can readily follow. Of course, peri-induction hypotension can also occur in the patient with well-preserved left ventricular function in the setting of hypovolemia and/or vasodilatation. With minimal TEE training, the anesthesiologist can quickly determine the contractile and/or the volume status of the heart in the mid-transgastric view.
Should the patient become hemodynamically unstable efforts are made to restore the vascular tone and improve contractility. Vasopressin 1 to 2 U bolus or as an infusion (up to 6 U/h) can be employed to restore vascular tone especially in those patients treated with an ACE-I. Inotropes can be used to improve myocardial contractility. TEE is used to guide volume loading of the heart and determine ventricular function as described in Chapter 2.
Diastolic Dysfunction
TEE is also used to discern the presence of diastolic dysfunction. Recall that many patients can present with symptoms of heart failure with a relatively well-preserved EF. Such patients have diastolic dysfunction. Failure of the heart to relax leads to an increase in left ventricular end-diastolic pressure (LVEDP) that is transmitted to the pulmonary vasculature leading to symptoms of congestion and heart failure. TEE can detect the presence of LV diastolic dysfunction through the use of PW Doppler. Recall that PW Doppler measures the velocity of flow at a specific point determined by the position of the sample gate. Placing the PW Doppler sample gate at the tips of the mitral valve during left ventricular filling will result in a characteristic transmitral diastolic flow pattern (Figure 5–5). For patients in sinus rhythm there are two peaks in the velocity of blood flow entering the LV during diastole. One peak occurs during early filling after the opening of the mitral valve (MV) (E wave) and the other peak occurs during atrial contraction (A wave) (Figure 5–6).
In subjects with normal diastolic function the ratio between E and A velocities varies from 0.8 to 2. There are various grades of severity of diastolic dysfunction. In early stages of diastolic dysfunction, the primary abnormality is impaired relaxation. When LV relaxation is delayed, the initial pressure gradient across the MV at the beginning of diastole is lower. The reduced transmitral pressure gradient results in a decline in the early filling with a greater proportion of filling seen during atrial contraction resulting in a decreased E velocity, increased A velocity, and a lower E/A ratio (Figure 5–7). This filling pattern represents grade I diastolic dysfunction or impaired relaxation. The impaired relaxation filling pattern can also be seen in elderly due to less vigorous relaxation of the LV. Thus, loss of the atrial contraction during periods of atrial fibrillation in older patients can significantly reduce the SV and lead to hemodynamic collapse. In healthy, younger patients, the atrial contraction does not play as essential a role in diastolic filling. Consequently, loss of the “P” wave on the ECG and atrial contraction is better tolerated hemodynamically.
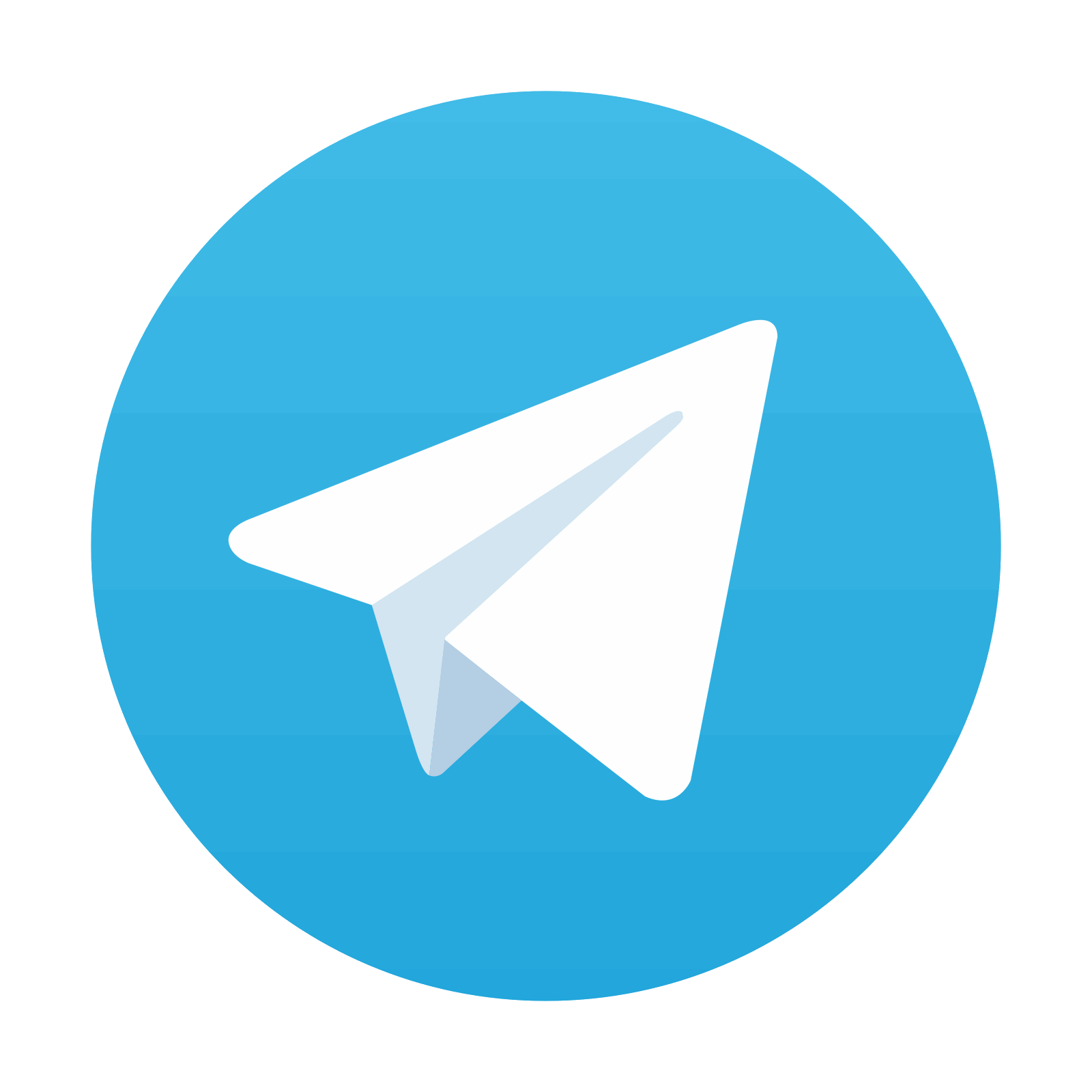
Stay updated, free articles. Join our Telegram channel

Full access? Get Clinical Tree
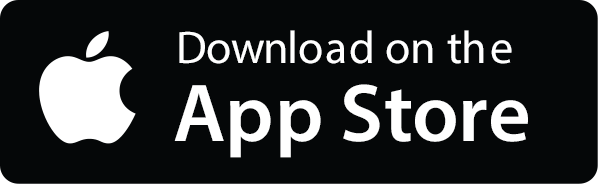
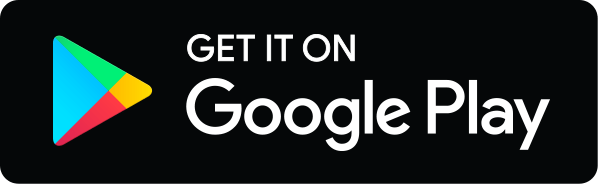