Significance of Perioperative Organ Injury
Although anesthesia-related complications have decreased in the last two decades, perioperative mortality has not. The leading precursor to death after surgery is acute organ injury progressing to single-organ or multiorgan failure. The failure of two or more vital organs has been termed “multiorgan dysfunction syndrome” (MODS). The common trigger for development of organ dysfunction or acute organ injury is the systemic inflammatory response. The pathophysiology of MODS involves an indiscriminate activation of the inflammation response by hostile stimuli, including sepsis, hypovolemia, trauma, or other circumstances involving significant tissue damage. Several organ systems are more at risk for perioperative organ injury. The acute stress response related to surgery has been shown to create a hypercoagulable state and to impair neuroprotective mechanisms, forming a potential for cerebral ischemia or stroke. Postoperative pulmonary and renal complications are similarly seen as an exacerbation of inflammatory pathways unless related to direct surgical trauma.
Recent advances in the understanding of the pathogenesis of organ dysfunction in sepsis indicate that coagulation abnormalities contribute strongly to its evolution. With a less organ-based but more histological approach, the systemic endothelial injury appears to be central for the development of organ failure. Shock-induced sympathoadrenal activation with excessive catecholamine levels induces systemic injury to endothelium and microcirculation of all organs. The natural anticoagulation protection of the endothelium is provided primarily by the heparinoid-rich glycocalyx, the tissue factor pathway inhibitor (TFPI), and the protein C/thrombomodulin system. In the case of tissue ischemia or inflammatory stress, the degradation/shedding of glycocalyx combined with coagulation activation and profibrinolytic factors from the injured endothelium may result in profound coagulation imbalance. Major surgery has been shown to induce a patient-specific inflammatory acute stress response contributing to the imbalance of the coagulation system, and the inflammatory environment may contribute to hypo- and hypercoagulability.
The coagulation factor activation, and indeed the entire coagulation cascade, extend beyond the clotting function. Relevant to perioperative stress, the coagulation proteins are connected to numerous proinflammatory signaling pathways. The overall state of coagulation in the circulation depends on the balance between pro- and anticoagulant mechanisms. When the balance is disturbed in favor of a procoagulant state, the proinflammatory signals of the coagulation proteins may be more dominant. Alternatively, disruption of coagulation ability may lead to bleeding, which has major clinical importance in the operative setting. Impaired coagulation ability and the consequences of bleeding in the operative setting are well recognized.
Several mechanisms contribute to the organ injury related to the activation of coagulation system, including extravascular fibrin deposition, small-vessel obstruction by thrombosis, and amplification of cellular inflammatory pathways. The procoagulant state has been shown to be especially important in the pathogenesis of sepsis-associated MODS. Similar mechanisms are probably pertinent to organ dysfunction in the perioperative period, when multiple proteins intermingle to alter the balance between procoagulant and anticoagulant. Attributing organ dysfunction solely to a specific type of surgical procedure is difficult, because the true incidence of perioperative organ dysfunction is unknown, and perioperative organ dysfunction severity appears to vary between surgical sites and procedures. Perioperative complications include postoperative sepsis and shock, which may produce coagulation abnormalities and organ dysfunction that are difficult to disassociate from the surgical event. The following paragraphs cover the basic coagulation and fibrinolytic functions and discuss alterations of these functions in the perioperative period.
Current Understanding of Coagulation and the Fibrinolytic Process
The traditional understanding of the coagulation cascade is that the coagulation is initiated by a sequential activation of several serine proteases, leading to a polymerization of the fibrin clot. The extrinsic pathway of the coagulation cascade is initiated by cell surface expression of tissue factor (TF), which binds to activated factor VII (FVIIa) to form a complex. The intrinsic pathway is initiated by contact activation. The TF–FVIIa complex converts factor X to factor Xa, which converts prothrombin to thrombin. Thrombin cleaves fibrinogen to fibrin. The cross-linking of fibrin generates the mechanical durable fibrin clot.
Focusing on a more in vivo understanding of coagulation, a cell-based model of the coagulation cascade was introduced by Hoffman and colleagues in 2003. The cell-based coagulation model operates on the notion that the “intrinsic” and “extrinsic” pathways are operating in parallel on different cell surfaces. The model describes four overlapping stages of coagulation: initiation, amplification, propagation, and stabilization. In the initiation phase, TF is expressed, and the extravascular TF binds to FVII, leading to FVII activation and forming the activated TF–FVIIa complex, which leads to the thrombin generation as described before. In the amplification phase, the small amount of thrombin produced by the TF–FVIIa complex allows platelet adhesion and activation. Thrombin is a very potent in vivo platelet activator. The activated platelets bind von Willebrand factor, cleave FVIII and activate it to FVIIIa. The propagation phase begins when FVa and FVIIIa are bound on the platelet surface. Large numbers of platelets are engaged to the site of injury, and surface FXa binds to FVa to produce a burst of thrombin generation for clot formation. During the stabilization phase, the formation of fibrin monomer and sufficient thrombin amount activate FXIII, which cross-links the fibrin into a stable fibrin clot ( Fig. 3.1 ).

The endothelium plays a pivotal role in the activation of the extrinsic coagulation pathway because injury to the endothelial glycocalyx exposes TF. Under normal conditions, natural anticoagulant systems protect the endothelium to limit fibrin generation and deposition to the specific injury site. The negatively charged glycocalyx is rich in heparinoids and interacts with antithrombin. Other natural anticoagulants include TFPI, proteins C and S, and antithrombin III (ATIII).
Fibrinolytic proteins function to regulate fibrin accumulation. Tissue and urokinase plasminogen activators initiate the plasminogen conversion to plasmin, which directly degrades fibrin. The fibrinolytic system is controlled by plasminogen activator inhibitors (PAIs). Increased PAI expression has been reported in several pathological situations, impairing fibrinolysis and therefore creating a hypercoagulable environment. To control the vascular injury in a normal host, surgical trauma generally promotes a more procoagulant state.
How Does Inflammation Interfere With/Change the Coagulation Process?
In addition to endothelial TF exposure secondary to surgical trauma, injured cells release endogenous molecules to communicate the cell injury to other pathways. TF activates immunologically active cells (monocytes and neutrophils). The TF activation leads to excess fibrin deposition. Natural anticoagulation mechanisms (including TFPI, antithrombin, protein C) are reduced or dysfunctional secondary to inflammation or tissue hypoperfusion. Protease-jactivated receptors (PARs) establish the link between coagulation and inflammation on the molecular level. Of the four PAR subtypes identified, PAR1 is particularly relevant in inflammatory pathophysiology. The cytoprotective effect of PAR1 is stimulated by activated protein C (APC) or a low amount of thrombin. When activated by high-dose thrombin, PAR1 has a more disruptive effect on the endothelial barrier.
The observation that a patient in septic shock had low APC levels and that microvascular thrombosis was partially responsible for impaired tissue oxygenation became the rationale for exploring APC in septic shock to improve patient outcome. Clinical trials failed to demonstrate a treatment benefit of APC or protein C zymogen but was associated with increased bleeding.
Perioperative Monitoring of Coagulation
Due to the complexity of the coagulation abnormalities in the perioperative period, conventional coagulation assessment with prothrombin time (PT), activated partial thromboplastin time (aPTT), and fibrinogen levels may not be sufficient or timely enough. The long turnaround times may render laboratory test results irrelevant before the information is available. In the operating room or in the intensive care unit, activated clotting time is a perioperatively available point-of-care test. The most concerning limitation of coagulation monitoring is its artificial nature, assessing isolated components of the coagulation system under artificial laboratory conditions. Recent publications are favoring perioperative coagulation monitoring using viscoelastic point-of-care testing such as thromboelastography (TEG) or rotational thromboelastometry (ROTEM). Although the technology is still FF and artificial, it more closely reflects the in vivo coagulation status. There are different variants of this technique currently available: TEG (Haemonetics Corp., Braintree, MA), ROTEM (Tem International GmbH, Munich, Germany), and Sonoclot (Sienco, Inc, Arvada, CO). The information obtained using this technology has been shown to be helpful for clinical decision-making during high-blood loss surgical procedures (trauma, cardiac surgery, and liver surgery) and for coagulation assessment during pregnancy.
Information obtained from TEG and ROTEM indicates (1) time to initiation of clot formation (R), (2) speed or rate of clot propagation (a), and (3) clot strength (amplitude A, or shear elastic modulus G) ( Fig. 3.2 ). The test takes into account both platelet function and fibrin–platelet interaction. Modifications of the method use inhibitors of platelet–fibrin interaction and inhibitors of platelet aggregation, and they can distinguish coagulation factors from platelet contributions to the clot formation. Viscoelasticity-based coagulation assessment and treatment have been shown to be helpful in multiple clinical situations with complex coagulopathies (trauma, liver transplantation, sepsis, and cardiopulmonary bypass). A test to assess not only platelet quantity but also quality is the platelet function analyzer (PFA-100; Dade International Inc., Miami, FL), which measures platelet aggregation or plug time. D-dimer assays are widely available to detect fibrin degradation products, and the presence of these products indicates activation of fibrinolysis, but the D-dimer is not necessarily a marker of increased fibrin formation.

Alteration of Coagulation Status During Perioperative Period
Surgical procedures and surgical stress commonly unbalance this elaborate arrangement, therefore causing hypo- or hypercoagulability. In addition to direct surgical injury to vascular structures and blood loss, fluid therapy-induced dilution of coagulation components, hypothermia, or tissue acidosis may promote hypocoagulability and bleeding. Surgical stress and tissue trauma release of TF may activate the coagulation process. The stress response also results in a downregulation of the anticoagulant response. The result of decreased anticoagulant activity, in combination with immobility and other patient factors (malignancy, drugs, and comorbidities), may be hypercoagulability.
During the perioperative period, the function of the coagulation system is altered by activation of the procoagulant mechanisms and depression of the anticoagulant and fibrinolytic mechanisms. The primary outcomes and events measured for perioperative activation of coagulation are vascular thrombotic complications, including stroke, myocardial infarction, and venous thromboembolism. Specific pathways and mechanisms have been implicated in surgery-associated hypercoagulability. Extrinsic coagulation is activated by vascular injuries that expose or upregulate TF on the endothelial surfaces and by platelet thrombi at sites of injury that also generate fibrin clot formation. Other surgical responses include increased levels of coagulation factors, decreased levels and activity of endogenous anticoagulants such as APC and ATIII, and inhibition of fibrinolytic function.
Several variables related to the surgical procedures and concurrent interventions affect the coagulation function in the perioperative period. Although several specific variables have been studied with respect to activation of the coagulation response, identifying the role of individual factors in the complexity of the whole surgical setting has been difficult. Variables that have been implicated include the pain or stress response, the mode of anesthesia, the type and quantity of intravenous fluid administration, body temperature, and the site of surgery.
Intraoperative Activation of Coagulation
Stress Response or Pain
Hypercoagulability has been reported as part of a complex stress or pain response. Enhanced coagulability developed over 15 minutes after insertion of a peripheral intravenous line in patients in the operative theater before undergoing elective cesarean section. This observation may be related to the stress response. This has also been inferred from other studies addressing effects of pain on coagulation parameters (see Pain Control, later).
Site of Surgery
Surgery itself puts the patient at risk for thrombotic complications resulting from immobilization, although some procedures seem to have especially high rates of clot formation. With the true incidence of venous thromboembolism unknown, it is estimated that 25% of in-hospital surgical patients are affected by venous thromboembolic events. In addition to patient-related factors (age, sex, obesity, and malignancy), the highest incidence is seen in orthopedic, abdominal, neurosurgical, and gynecological procedures. Although an increased likelihood of thrombotic complications is certainly influenced by the degree of immobilization required by the procedure, it may also reflect differential activation of coagulation related to operative location. Coagulation abnormalities have been demonstrated after a number of different types of surgical procedures. In certain surgical settings (cardiovascular, trauma, and obstetric surgical settings), the primary cause of bleeding may be more anatomical than pathophysiological. Acquired coagulopathy may occur secondary to blood loss, hemodilution, and loss or consumption of coagulation factors. After major abdominal surgery, such as the Whipple procedure or pancreatic, gastric, or rectal resections, multiple signs of activation of both coagulation and fibrinolysis can be demonstrated by the end of the procedure, including decreased ATIII and fibrinogen. In addition, D-dimer and platelet aggregation are elevated several hours after surgery and continue to be elevated on the first postoperative day. Although the available literature has not compared coagulation parameters between different procedures, certain sites of surgery may be more apt to activate coagulation. For example, the brain is highly enriched in TF, and the release of TF during parenchymal manipulation may explain an apparent increased susceptibility to thrombosis in neurosurgical patients undergoing craniotomy. In the era before routine deep venous thrombosis (DVT) prophylaxis, such patients had a 29%–42% incidence of thrombotic complications. Vascular procedures are also likely to activate coagulation because of intravascular stimulation of TF exposure.
Minimally invasive surgical techniques may have less impact on the coagulation system. Comparisons of open versus laparoscopic procedures have shown that open surgery was associated with higher levels of thrombin–antithrombin complexes and prothrombin fragments postoperatively. Other studies have found more prothrombotic coagulation changes in patients after laparoscopic procedures. Although several observational studies have documented changes in coagulation profile related to laparoscopic versus open technique, it is currently unclear if these laboratory changes have clinical significance. The influence of surgical technique on coagulation may be related to surgical procedure, surgical duration (venostasis), and patient factors (e.g., comorbidities, malignancy). Anesthesia-related factors (neuraxial technique for postoperative analgesia, fluid management, and anesthesia agents) may contribute as well.
Mode of Anesthesia
On the basis of several studies, epidural anesthesia reduces the incidence of thrombotic events and is associated with beneficial effects on postoperative outcome compared with general anesthesia. Patients receiving epidural or spinal (neuraxial) anesthesia show diminished elaboration of procoagulants compared with those undergoing general anesthesia. Other investigations have shown that after epidural anesthesia, patients appear to maintain adequate fibrinolysis, as evidenced by lower release of PAI-126 and enhanced release of plasminogen activators. In addition, it is proposed that certain local anesthetic agents, such as lidocaine, may have anti-inflammatory actions, which could potentially attenuate recruitment of procoagulant mechanisms. In a meta-analysis of studies of complications comparing general anesthesia alone with neuraxial anesthesia (epidural or spinal anesthesia), it was reported that in addition to having an overall benefit, concomitant neuraxial blockade reduced the odds of thrombotic complications, including DVT and pulmonary embolism, by 44% and 55%, respectively. This meta-analysis is confounded by the inclusion of studies of multiple surgical procedures and sites, which limits the conclusions. Moreover, in some of the studies reviewed, the use of general anesthesia was combined with neuraxial blockade for postoperative pain control. Thus, the effect of pain on coagulation was a confounding variable in the analysis.
Regional anesthesia and adequate pain control are core principles of all enhanced recovery after surgery (ERAS) guidelines, providing supportive evidence of the potential impact of these techniques of controlling the inflammatory response and therefore improving outcomes.
Intravenous Fluid Management
The amount and quality of intraoperative fluid therapy may have an impact on the coagulation system during the perioperative period. Obviously, an excessive amount of crystalloid or colloid intravenous fluid can have a dilutional effect and cause an acquired coagulopathy.
Large-volume crystalloid administration and dilutional coagulopathy in trauma is associated with poor outcome. Because intravenous solutions do not contain coagulation factors, the dilutional coagulopathy is nonspecific to the solution used. However, specific anticoagulant effects are specific to the agent used. Intravenous fluid replacement is undertaken with crystalloid, such as 0.9% sodium chloride, lactated Ringer, or synthetic colloids. Colloid plasma substitutes are thought to lead to hemostatic derangement independent of effects on hemodilution. A meta-analysis of studies of colloids and hemostatic function concluded that these fluids are most likely to result in impaired coagulation when given in large volumes. Colloids used in the perioperative period are hydroxyethyl starch (HES), dextran, gelatins, and albumin. The different HES solutions are slowly degradable HES (heta-, hexa-, and pentastarch) or rapidly degradable HES (tetrastarch). The specific anticoagulant effect differs between colloids (dextran > hetastarch > tetrastarch, gelatins > albumin). The anticoagulant activity is caused by transient decrease in FVIII and von Willebrand factor levels, impaired thrombin–fibrinogen interaction, impaired thrombus generation, impaired FXIII–fibrin polymer interaction, and enhanced fibrinolytic activity. Platelet reactivity is reduced because of diminished glycoprotein IIb-IIIa availability and decreased platelet aggregability/adhesion.
Viscoelastic coagulation assessment has been shown to be helpful if non–blood component therapy is used for major fluid loss replacement in the perioperative period to assess the severity of the acquired coagulopathy. Advanced coagulation assessment is needed to use physiological transfusion triggers and allow more restrictive blood component therapy practice.
Body Temperature
Hypothermia is encountered during surgical procedures, particularly following shock and massive resuscitation. Decreased body temperature may alter the coagulation and clotting function responses outlined earlier. Starting at a core temperature of less than 36°C (mild-moderate hypothermia < 36–33°C), coagulation ability is impaired by decreased platelet aggregation and adhesion and decreased TF activity. Hypothermia contributes to the trauma coagulopathy described earlier. Surgical procedures with a wide range of temperature fluctuations (e.g., hyperthermic intraperitoneal chemotherapy, cardiopulmonary bypass) are prone to display complex coagulation abnormalities. Various studies documented temperature-related abnormal coagulation tests (PT, aPTT) but also objective reduction in coagulation factor activity (ATIII, fibrinogen, FXIII, and thrombocytopenia). Perioperative monitoring with TEG is encouraged if complex perioperative coagulation abnormalities are expected or suspected.
Trauma Coagulopathy
Recent reviews have described the current understanding of the complex coagulation disorder occurring after massive trauma when hypoperfusion, shock, and vascular damage are present. In addition to significant blood loss and hemodilution, the tissue injury triggers an endogenous coagulation response that is amplified by hypothermia and acidemia. Although the increased catecholamine levels initiate endothelial changes, as described earlier, the endogenous response entails increased APC, fibrinogen depletion via fibrinolysis, and platelet dysfunction. APC and fibrinolysis have a critical role in the pathophysiology of trauma-induced coagulopathy. Protein C is a serine protease with anticoagulant and inflammation-modulating functions. Initiated by tissue injury and hypoperfusion, elevated endothelial and circulating thrombomodulin bind thrombin to form a thrombomodulin–thrombin complex, which subsequently activates protein C. APC inhibits clot formation by deactivating FV and FVIII and depleting PAIs. The reduced activity of thrombin-activated fibrinolysis inhibitors further exacerbates the process. The result is unopposed fibrinolysis.
The dilutional and consumptive characteristics of the trauma-induced coagulopathy are not easily identified using conventional in vitro testing. Early recognition and a hemostatic resuscitation approach (damage control resuscitation) are critical to improving patient outcomes.
Perioperatively, point-of-care monitoring based on viscoelastic properties (TEG or ROTEM) has been used in management of trauma patients and has been shown to improve blood component therapy use. Point-of-care TEG may be useful to guide the use of antifibrinolytic therapy in trauma patients.
Postoperative Activation of Coagulation
Several important variables in the postoperative course have an impact on coagulation function and the possible risk of organ dysfunction. Increased coagulation parameters have been found to continue into the postoperative period. Complications (such as sepsis and hypotension) and requirements for further fluid resuscitation appear to be major factors that perpetuate coagulation abnormalities during this time.
Pain Control
Pain is one very important variable that is determined to contribute to coagulation abnormalities. As mentioned previously (see Mode of Anesthesia section earlier), it has been suggested that local anesthetic agents may have biochemical effects that alter coagulation. Improved pain control may have a beneficial effect on coagulation state by reducing perioperative stress, but available studies do not allow these effects to be delineated clearly. One study compared postoperative pain control using epidural anesthesia with narcotic analgesia in patients undergoing major surgery for peripheral vascular disease under general anesthesia. Patients in the epidural analgesia group had lower activation of coagulation, as evidenced by both thromboelastography and number of vascular events, including venous thromboembolism, myocardial infarction, and thrombosis of the vascular graft. However, another group showed that in a large number of patients undergoing abdominal aortic surgery, there was no difference between the number of major organ failures in patients treated intensively with neuraxial pain control and those treated with narcotic analgesia.
Interesting research developments are the influences of opioids and propofol on patient outcomes after oncologic procedures. On the basis of current understanding of how surgical stress and anesthesia may interfere with immune response, it may not be unreasonable to stipulate a connection between the immune system and the coagulation system, with an important impact on outcomes.
Consequences of Dysregulated Coagulation
When coagulation balance is disrupted to favor procoagulant mechanisms, organ dysfunction is elicited by the deposition of fibrin in the vessel lumen and tissue parenchyma as well as by proinflammatory actions of coagulation proteins. The association between activation of coagulation and organ dysfunction is established in sepsis and other systemic inflammatory states. The following paragraphs briefly review these mechanisms and point out their potential relevance in perioperative organ dysfunction, which remains largely unexplored.
Proinflammatory Actions of Coagulation Proteases
Several key proteins in the extrinsic coagulation system are capable of generating intracellular signals. TF is an integral membrane glycoprotein with structural features of a class 2 cytokine receptor. TF is expressed on monocytes or macrophages and on endothelial cell surfaces, and after binding by circulating FVIIa, its interaction with other cell receptors or direct intracellular action leads to increased oxidant production, protein tyrosine phosphorylation, and calcium oscillations in human cells. Signal transduction pathways activated by the FVIIa/TF complex include p44/42 (extracellular signal-regulated kinase) and p38 mitogen-activated protein kinase. These signaling pathways activate inflammatory transcription factors and expression of proteins, such as interleukin-6 and vascular endothelial growth factor, that further amplify inflammation and may promote capillary leak.
FXa and thrombin are serine protease components of the coagulation cascade downstream from TF-based initiation that have independent inflammatory signaling functions. As previously discussed, FXa attaches to a cell surface–based receptor, effector cell protease receptor-1 (EPR-1), and thrombin binds to PAR1. Multiple proinflammatory roles of EPR-1 and PAR1 have been demonstrated, including nuclear factor-κB activation. Proliferative and proinflammatory responses to thrombin have been demonstrated in endothelial cells and macrophages, respectively.
Summary and Recommendations
Anesthesiologists have a crucial role in improving the outcomes of surgical patients. Improving understanding of the pathophysiology of perioperative organ injury should facilitate the development of preventive or therapeutic strategies. The pathophysiology of coagulation abnormalities leading to perioperative organ dysfunction is an area of ongoing investigation. This understanding has been well accepted in organ injury related to sepsis and trauma, and it is quite likely relevant to perioperative conditions when coagulation abnormalities are present. In addition to the effects of surgical trauma, many other variables are known to affect the evolution of coagulation abnormalities, including pain, fluid replacement, and anesthetic regimen. Of special interest as possible therapeutic targets are procoagulant aspects of hemodilution and anticoagulant effects of colloids and anti-inflammatory and anticoagulant effects of propofol, opioids, non–opioid-based analgesics, and local anesthetic agents. These factors correlate with changes in coagulation status, but much work remains to be done to connect them causally to less obvious effects such as organ dysfunction. Furthermore, true incidences of organ dysfunction remain to be defined, as do special aspects related to the surgical procedure, surgical technique, and perioperative care. Care paradigm changes such as ERAS guidelines may influence patient outcomes, partially due to changes in coagulation activation. Finally, much of the available data were obtained prior to or without regard to standard practices of postoperative thromboembolism prophylaxis. A modern approach to coagulation abnormalities in the postoperative setting needs to be developed to evaluate their effects on outcome, especially involving postoperative infection and organ failure. Recommendations for thromboembolism prophylaxis should be followed rigorously and organ function closely monitored in all postoperative patients at high risk for complications of hypercoagulability.
References
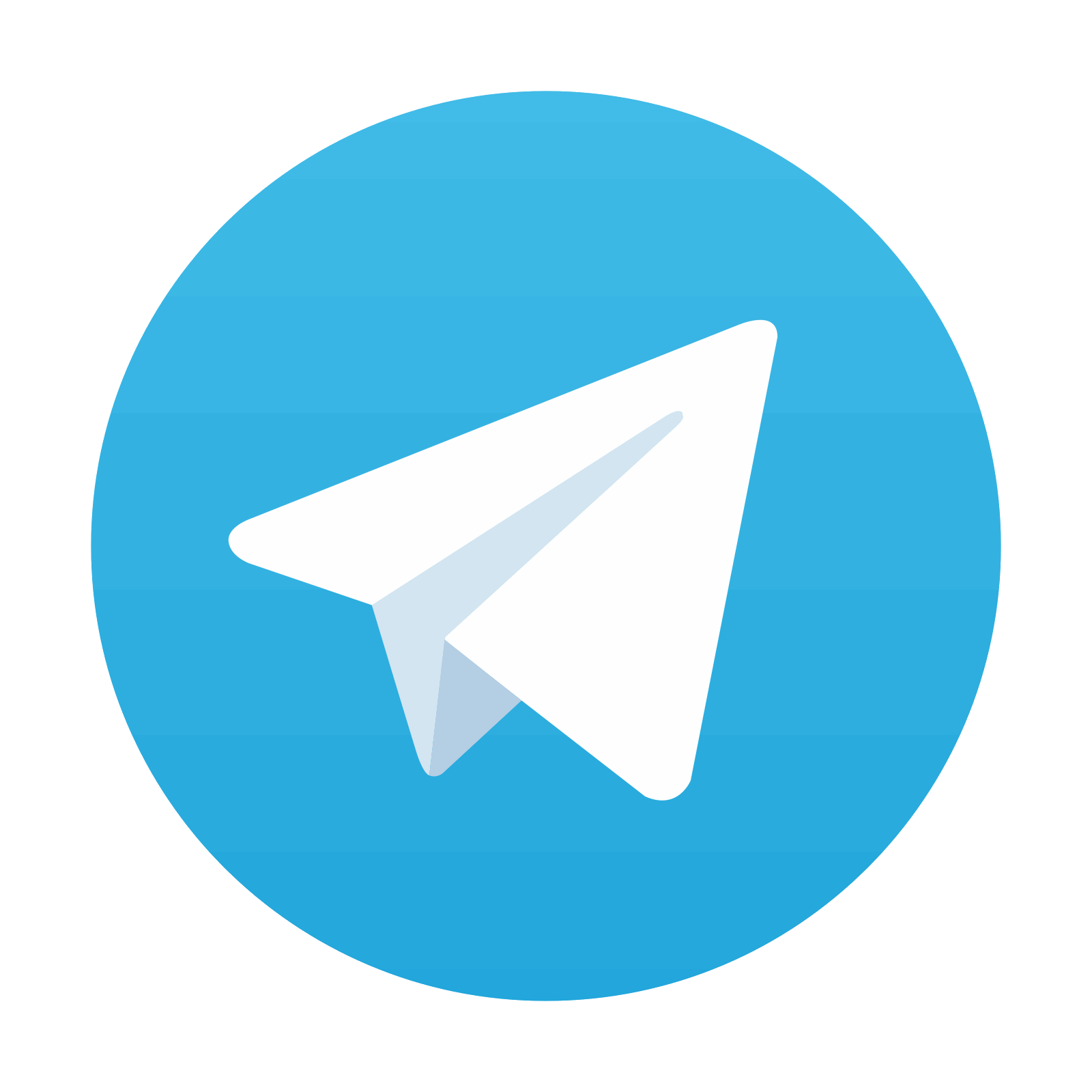
Stay updated, free articles. Join our Telegram channel

Full access? Get Clinical Tree
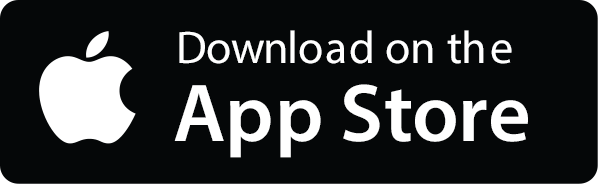
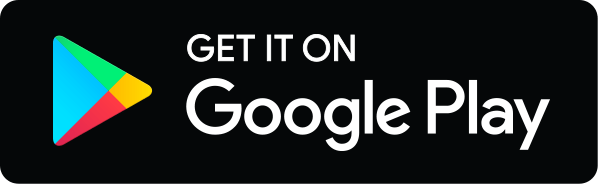
