
Epinephrine
Epinephrine is the prototype sympathomimetic. It is a circulating hormone synthesized, stored, and released from the adrenal medulla. Its natural functions upon release into the circulation include regulation of myocardial contractility, heart rate, vascular and bronchial smooth muscle tone, glandular secretions, and metabolic processes such as glycogenolysis and lipolysis. It is a potent activator of α-adrenergic receptors and also activates β1 and β2 receptors. Oral administration is not effective as epinephrine is rapidly metabolized in the gastrointestinal mucosa and liver. Therefore, epinephrine is administered subcutaneously, intravenously, or intramuscularly. Absorption after subcutaneous injection is slow because of local epinephrine–induced vasoconstriction. Epinephrine is poorly lipid soluble, preventing its ready entrance into the central nervous system (CNS) and accounting for the lack of cerebral effects.
Clinical Uses
Clinical uses of epinephrine include treatment of life-threatening allergic reactions/anaphylaxis, treatment of severe asthma and bronchospasm, administration during cardiopulmonary resuscitation as a vital therapeutic drug,1 administration during periods of hemodynamic instability to promote myocardial contractility and increase vascular resistance, and continuous infusion for continuous support of myocardial contractility and vascular resistance. Epinephrine is used to promote inotropy during weaning from cardiopulmonary bypass. It is also used as a vasoconstrictor to increase oxygen delivery and increase cardiac output in sepsis.2,3 Lastly, epinephrine is added to local anesthetic solutions to decrease systemic absorption prolonging the duration of action of the anesthetic for regional and local anesthesia. Epinephrine is used in local and field blocks to promote a bloodless surgical field.
Cardiovascular Effects
The cardiovascular effects of epinephrine result from stimulation of α- and β-adrenergic receptors (see Table 18-1).4 Stimulation of α1 receptors leads to arteriolar vasoconstriction and pulmonary artery vasoconstriction. α1 Receptors are predominantly located in cutaneous, splanchnic, and renal vascular beds. Stimulation of α2 receptors also leads to vasoconstriction. β2 Receptor stimulation leads to vasodilation, predominantly in the skeletal muscles. The relative balance of α1 and β2 receptors in the vasculature of an organ determines epinephrine’s overall effect on blood flow to the organ. The net effect of these changes in peripheral vascular tone is preferential distribution of cardiac output to skeletal muscles and increased systemic vascular resistance. Renal blood flow is substantially decreased by epinephrine, even in the absence of changes in systemic blood pressure. Epinephrine is estimated to be 2 to 10 times more potent than norepinephrine as a renal vasoconstrictor. In general, β2 receptors are more sensitive to lower epinephrine doses while effects on α1 receptors predominate at higher doses. At high doses, the predominant α activity and resultant vasoconstriction leads to increased afterload, which may impede increases in cardiac output. Initial tachycardia may be followed by heart rate decreases due to baroreceptor reflexes. Venous return is also enhanced by venoconstriction from the high density of α receptors in the venous vasculature system. Blood pressure is increased by an increase in cardiac index as well as an increase in systemic vascular resistance.
Epinephrine stimulates β1 receptors causing an increase in heart rate, myocardial contractility, and cardiac output. There may be a mild decrease in diastolic blood pressure, reflecting vasodilation in skeletal muscle vasculature due to stimulation of β2 receptors. The net effect of these systemic blood pressure changes is an increase in pulse pressure and a mild change in mean arterial pressure.
Epinephrine increases heart rate by accelerating the rate of spontaneous phase 4 depolarization, which also increases the likelihood of cardiac dysrhythmias. Epinephrine increases conduction velocity and decreases the refractory period in the atrioventricular node, bundle of His, Purkinje fibers, and ventricular muscle. It also may increase automaticity of latent pacemakers. Tachycardia, premature ventricular contraction, ventricular tachycardia, and ventricular fibrillation all may occur.
Increased cardiac output reflects epinephrine-induced increases in heart rate, myocardial contractility, and venous return. Repeated doses of epinephrine produce similar cardiovascular effects in contrast to the tachyphylaxis that accompanies administration of synthetic noncatecholamines that cause the release of norepinephrine, such as ephedrine. Myocardial oxygen consumption is increased with enhanced left ventricular preload, increased contractility, increased afterload, and tachycardia. Diastolic function is improved by increasing the rate of myocardial relaxation, and early left ventricular filling is enhanced.
Epinephrine stimulates renal β receptors, resulting in increased secretion of renin. In usual therapeutic doses, epinephrine has no significant vasoconstrictive effect on cerebral arterioles. Coronary blood flow is enhanced by epinephrine,5 even at doses that do not alter systemic blood pressure.
Chronic increases in the plasma concentrations of epinephrine, as in patients with pheochromocytoma, result in a decrease of plasma volume because of a loss of protein-free fluid into the extracellular space. Arterial wall damage and local areas of myocardial necrosis may also accompany chronic circulating excesses of epinephrine. Conventional doses of epinephrine, however, do not produce these effects.
The hemodynamic effects of epinephrine are attenuated and can be blocked by prior administration of α- or β-adrenergic receptor antagonists.4 Supratherapeutic doses of epinephrine may lead to acute heart failure, pulmonary edema, arrhythmias, hypertension, and myocardial ischemia.
Airway Smooth Muscle
Smooth muscles of the bronchi are relaxed by epinephrine-induced activation of β2 receptors. By increasing intracellular concentrations of cyclic adenosine monophosphate (cAMP), β2 stimulation decreases release of vasoactive mediators associated with symptoms of bronchial asthma. The bronchodilating effects of epinephrine are not seen in the presence of β-adrenergic blockade. In the presence of β-adrenergic blockade, epinephrine instead induces bronchoconstriction from stimulation of bronchial α receptors.
Metabolic Effects
Epinephrine has the most significant effect on metabolism of all the catecholamines.6 β1 Receptor stimulation due to epinephrine increases liver glycogenolysis and adipose tissue lipolysis, whereas α1 receptor stimulation inhibits release of insulin. Liver glycogenolysis results from epinephrine-induced activation of hepatic phosphorylase enzyme. Lipolysis is due to epinephrine-induced activation of triglyceride lipase, which accelerates the breakdown of triglycerides to form free fatty acids and glycerol. Infusions of epinephrine usually increase plasma concentrations of glucose, cholesterol, phospholipids, and low-density lipoproteins. Release of endogenous epinephrine and the resulting glycogenolysis and inhibition of insulin secretion is the most likely explanation for perioperative hyperglycemia. In addition, epinephrine can inhibit peripheral glucose uptake, which is also due in part to inhibition of insulin secretion. Increased plasma concentrations of lactate presumably reflect epinephrine-induced glycogenolysis in skeletal muscles. Some studies demonstrate that epinephrine-induced hyperlactemia is primarily a transient phenomenon associated with inhibition of pyruvate dehydrogenase and has no relationship with cellular hypoxia and tissue perfusion or associated metabolic acidosis.7
Electrolytes
Selective β2-adrenergic agonist effects of epinephrine are speculated to reflect activation of the sodium–potassium pump in skeletal muscles, leading to a transfer of potassium ions into cells (Fig. 18-2).8 The observation that serum potassium measurements in blood samples obtained immediately before induction of anesthesia are lower than measurements 1 to 3 days preoperatively is presumed to reflect stress-induced release of epinephrine (Fig. 18-3).9 The ability of a nonselective β1 and β2 antagonist (propranolol) but not a cardioselective β1 antagonist (atenolol) to prevent “preoperative hypokalemia” is consistent for a β2-adrenergic agonist effect as the explanation for potassium transfer (Fig. 18-4).9 In making therapeutic decisions based on a preinduction serum potassium measurement, especially in patients without a reason to experience hypokalemia, one should consider the possible role of preoperative anxiety and the release of epinephrine.



Epinephrine-induced hypokalemia may contribute to cardiac dysrhythmias, which occasionally accompany stimulation of the sympathetic nervous system. Conversely, epinephrine may stimulate the release of potassium from the liver, tending to offset the decrease in extracellular concentration of this ion produced by entrance into skeletal muscles.
Ocular Effects
Epinephrine causes contraction of the radial muscles of the iris, producing mydriasis. Contraction of the orbital muscles produces an appearance of exophthalmos. Adrenergic receptors responsible for these ocular effects are likely α receptors as norepinephrine is less potent than epinephrine and isoproterenol has practically no ocular effects.
Gastrointestinal and Genitourinary Effects
Epinephrine, norepinephrine, and isoproterenol produce relaxation of gastrointestinal smooth muscle. Activation of β-adrenergic receptors relaxes the detrusor muscle of the bladder, whereas activation of α-adrenergic receptors contracts the trigone and sphincter muscles.
Hepatosplanchnic vasoconstriction occurs as well as impaired renal blood flow as cardiac output is diverted to the dilated skeletal muscle vasculature.10 This results in decreased hepatosplanchnic oxygen exchange and lactate clearance. The impairment of splanchnic circulation that occurs is greater than that associated with norepinephrine or dopamine.11,12 It may be dependent on the severity of the shock state and the dose of epinephrine used.
Coagulation
Coagulation is accelerated by epinephrine. A hypercoagulable state present during the intraoperative and postoperative period may reflect stress-associated release of epinephrine. Epinephrine increases the total leukocyte count but causes eosinopenia. It is a potent inducer of platelet aggregation and increases factor V activity.
Norepinephrine
Norepinephrine is the endogenous neurotransmitter synthesized and stored in postganglionic sympathetic nerve endings and released with sympathetic nerve stimulation. It is the immediate precursor of epinephrine. Norepinephrine stimulates β1– and α1-adrenergic receptors. It is approximately equal in potency to epinephrine for stimulation of β1 receptors but, unlike epinephrine, has minimal effect at β2 receptors (see Table 18-1). Through its action on β1 receptors, norepinephrine increases heart rate, conduction, and contractility. Norepinephrine is a potent α1 agonist producing intense arterial and venous vasoconstriction in all vascular beds except for the coronary arteries.13 Norepinephrine causes greater increase in systemic vascular resistance and diastolic blood pressure, mean arterial pressure, and systolic blood pressure than epinephrine. Venous vasoconstriction decreases venous capacitance, thereby increasing venous return to augment stroke volume and cardiac output. Heart rate changes may be minimal as baroreceptor reflexes triggered by arterial vasoconstriction are counteracted by β1-mediated increases in heart rate. This is in contrast to epinephrine, which has a more significant chronotropic effect. Norepinephrine increases mean arterial pressure primarily by vasoconstriction and to lesser degree by increasing stroke volume and cardiac output. At higher doses, vasoconstriction predominates to an even greater extent. Norepinephrine and epinephrine increase total peripheral vascular resistance more than dobutamine (described in the following texts). Unlike epinephrine, norepinephrine has minimal metabolic effects. Hyperglycemia is unlikely to occur as a result of norepinephrine administration.
Intravenous administration of norepinephrine results in intense vasoconstriction in skeletal muscle, liver, kidneys, and skin vascular beds. Peripheral vasoconstriction may decrease tissue blood flow to the extent that metabolic acidosis occurs. Chronic infusion of norepinephrine or increased circulating concentrations of this catecholamine, as may be associated with pheochromocytoma, may cause precapillary vasoconstriction and loss of protein-free fluid into the extracellular space. Like epinephrine, norepinephrine dilates coronary arteries.13
A continuous infusion of norepinephrine, 2 to 16 µg per minute, may be used to treat refractory hypotension. Placement of norepinephrine in a 5% glucose solution provides sufficient acidity to prevent oxidation of the catecholamine. Extravasation during infusion can produce severe local vasoconstriction and possible necrosis; hence, administration should be via central venous access. Norepinephrine administration should be accompanied by invasive hemodynamic monitoring. The primary method of elimination is by reuptake into the adrenergic nerve endings where it is stored for subsequent release with only small amounts being metabolized.
Clinical Uses
The primary use of norepinephrine is as a potent vasoconstrictor to increase total peripheral vascular resistance and mean arterial pressure. It is a first-line agent in the treatment of refractory hypotension during severe sepsis.2,3,14 Norepinephrine-induced vasoconstriction and redistribution of flow may increase splanchnic blood flow and urine output in severely hypotensive septic patients. Norepinephrine is also used for patients with low systemic vascular resistance after cardiopulmonary bypass. In patients with coronary artery disease, norepinephrine can be used to maintain perfusion pressure, although it should be balanced with the resultant increased afterload associated with high doses.
Side Effects
Norepinephrine should be used cautiously in patients with right ventricular failure. Norepinephrine increases venous return to the heart and also raises pulmonary artery pressures via stimulation of pulmonary vascular α1-adrenergic receptors. Both of these may be poorly tolerated by patients with right heart failure.
The use of norepinephrine as an inotropic agent is limited by its action as a potent vasoconstrictor. The increased peripheral resistance and afterload may decrease cardiac output and increase the work of the left ventricle. Its use is also limited by the potential for tachycardia, although its arrhythmogenic potential is less than that of epinephrine.
One of the major concerns with norepinephrine use is organ ischemia. Excessive vasoconstriction and decreased perfusion of renal, splanchnic, and peripheral vascular beds may lead to end-organ hypoperfusion and ischemia. Renal arteriolar vasoconstriction may lead to oliguria and renal failure. However, when large doses of norepinephrine accompanied by adequate fluid volume resuscitation are used with caution to treat profound hypotension, perfusion pressure and renal blood flow may actually increase.
Dopamine
Dopamine is an endogenous catecholamine that is the immediate precursor of norepinephrine. Dopamine regulates cardiac, vascular, and endocrine function and is an important neurotransmitter in the central and peripheral nervous systems. The pharmacology of dopamine is complex as this catecholamine differentially stimulates a variety of dopaminergic as well as adrenergic receptors. It is a relatively nonspecific agonist at both dopamine1 (D1) and dopamine2 (D2) receptors and the α- and β-adrenergic receptors. D1 receptors are located postsynaptically. When activated D1 receptors elicit vasodilation in renal, mesenteric, coronary, and cerebral vascular beds and inhibition of sodium–potassium adenosine triphosphatase (Na+–K+ ATPase).15 Activation of these receptors is mediated by adenylate cyclase stimulation. D2 receptors are principally presynaptic and inhibit adenylate cyclase activity and release of norepinephrine in autonomic nervous system ganglia and adrenergic nerves (in renal and mesenteric vessels) leading to vasodilation.16,17 D2 receptors are also present in the pituitary gland, emetic center of the medulla, and kidney. Nausea and vomiting produced by dopamine probably reflect stimulation of D2 receptors. Dopamine receptors may also be associated with the neural mechanism for “reward” that is associated with cocaine and alcohol dependence.
Traditionally, the pharmacokinetics of dopamine has been attributed to dose-dependent effects on varying receptors.16 At low intravenous (IV) infusion rates (0.5 to 3 µg/kg/minute), dopamine primarily stimulates D1 and D2 receptors leading to vasodilation, decreased arterial blood pressure, and increased renal and splanchnic vascular blood flow. Diuresis and natriuresis also occur. The decrease in diastolic blood pressure might lead to a reflex increase in heart rate. At higher infusion rates (3 to 10 µg/kg/minute), dopamine primarily stimulates β1-adrenergic receptors in the heart as well as α receptors in the peripheral vasculature. It also induces norepinephrine release from vascular sympathetic neurons. The activation of β receptors leads to increased cardiac output by increasing chronotropy and contractility along with vasodilation and afterload reduction. As the infusion rate is increased even further (>10 µg/kg/minute), dopamine predominantly stimulates α1 receptors, acting similarly to a pure α agonist. The predominant stimulation of vascular smooth muscle α1 receptors at these higher doses lead to arterial and venous vasoconstriction, increased systemic vascular resistance, and increased blood pressure attenuating further increases in cardiac output. Reflex bradycardia may also occur at this point.
This aforementioned dose-dependent model of dopamine’s effects is too simplistic, even in healthy individuals. There are a wide range of clinical responses depending on individual variability in pharmacokinetics as well as other variables. For example, despite identical IV infusion rates, there may be a 10- to 75-fold variability in plasma dopamine concentrations produced even in healthy individuals with normal drug metabolism.18 The etiology of the wide pharmacokinetic variability and variation in individual responses is likely multifactorial, reflecting differences in drug distribution, elimination, and endogenous levels, among other factors. Such differences may be even more profound in critically ill patients. Hence, the effects of dopamine cannot be predicted based on the dose,19 and the drug must be titrated to effect.
Dopamine increases cardiac output by stimulation of β1 receptors, increasing stroke volume. This increase in cardiac output is usually accompanied by only modest increases in heart rate, systemic blood pressure, and systemic vascular resistance. A portion of the effect of dopamine is also due to stimulation of endogenous norepinephrine release, which may predispose to the development of cardiac dysrhythmias. Nevertheless, dopamine is less dysrhythmogenic than epinephrine. The release of norepinephrine caused by dopamine may be an unreliable mechanism for increasing cardiac output when catecholamine stores are depleted, as occurs with patients in chronic cardiac failure. Dopamine increases myocardial oxygen consumption.
Dopamine causes both relaxation and contraction of vascular smooth muscle with the predominant effect varying by vascular bed, predominant receptor type, and dose administered. Dopamine’s effect on pulmonary vascular resistance has not been well studied in humans, though there are some reports it may decrease pulmonary vascular resistance in patients with chronic obstructive pulmonary disease.
Rapid metabolism of dopamine with an elimination half-life of 1 to 2 minutes mandates its use as a continuous infusion (1 to 20 µg/kg/minute) to maintain therapeutic plasma concentrations. Dopamine should be dissolved in 5% glucose in water for IV administration to avoid the inactivation that may occur in alkaline solutions. Extravasation of dopamine, like norepinephrine, produces intense local vasoconstriction, which may be treated with local infiltration of phentolamine.20 Dopamine is not effective orally and does not cross the blood–brain barrier in sufficient amounts to cause CNS effects. The immediate precursor of dopamine, L-dopa, is absorbed from the gastrointestinal tract and readily crosses the blood–brain barrier. Dopamine is partially protein bound. Approximately 25% is converted to norepinephrine. Dopamine undergoes metabolism in the liver with conjugation to sulfates and glucuronides, pulmonary endothelium by catechol-O-methyltransferase (COMT) and excretion by the kidneys.
Clinical Uses
Dopamine is used clinically to increase cardiac output in patients with decreased contractility, low systemic blood pressure, and low urine output as may be present after cardiopulmonary bypass or with chronic heart failure.21 It is unique among the catecholamines in being able to simultaneously increase myocardial contractility, renal blood flow, glomerular filtration rate, excretion of sodium, and urine output.22 Dopamine exerts its positive chronotropic, dromotropic, inotropic, and lusitropic (myocardial relaxant) effects via β1-adrenergic receptors. Activation of arterial and venous α1 receptors increases systemic vascular resistance, preload, and left ventricular afterload.23 However, as dopamine increases pulmonary vascular resistance as well, it may not be the preferred inotropic agents in patients with pulmonary hypertension or right ventricular dysfunction.
The divergent pharmacologic effects of dopamine and dobutamine make their use in combination potentially useful. For example, infusions of dopamine and dobutamine in combination have been noted to produce a greater improvement in cardiac output, at lower doses, than can be achieved by either drug alone. Both are inotropic but each drug dilates different vascular beds such that the summation of afterload reduction by both drugs may produce a greater improvement in cardiac output than could be achieved by either drug alone. Dopamine may distribute the cardiac output to the renal and mesenteric vascular beds, whereas dobutamine may provide additional afterload reduction by dilating skin and skeletal muscle vascular beds. The objective of combination therapy is to increase coronary perfusion and cardiac output while decreasing afterload, similar to an intraaortic balloon pump.
Renal-Dose Dopamine
The term renal-dose dopamine or low-dose dopamine refers to the continuous infusion of small doses (1 to 3 µg/kg/minute) of dopamine to patients to promote renal blood flow. In healthy individuals, low-dose dopamine increases renal blood flow and induces natriuresis and diuresis. Theoretically, dopamine’s renal vasodilating effects may be useful in patients with impaired renal function or in patients at risk of decreased renal perfusion as may occur with decreased cardiac output. Small doses of dopamine increase renal blood flow predominantly by D1 receptors in the renal vasculature and possibly by D2 receptors via inhibition of norepinephrine release. Larger doses predominantly increase renal blood flow by β-adrenergic–mediated increases in cardiac output. Higher doses of dopamine presumably stimulate α receptors to increase perfusion pressure. In addition, dopamine triggers natriuresis and diuresis through direct effect on tubular cell function. Dopamine binds to D1 and D2 receptors in the proximal tubule, thick ascending loop of Henle, and cortical collecting ducts inhibiting Na+–K+ ATPase activity, increasing Na+ excretion, inducing natriuresis and diuresis.24 The activation of D2 in inner medullary collecting ducts stimulates prostaglandin E2 (PGE2) production. This antagonizes the effects of antidiuretic hormone and results in increased free water clearance. PGE2 enhances blood flow in the inner medulla. Inhibition of aldosterone also increases sodium excretion and diuresis. Hence, dopamine has direct and indirect effects on the renal vasculature in addition to functioning as a diuretic.
The term renal-dose or low-dose dopamine is misleading as dopamine has many effects at sites other than the kidneys, even at low doses. Dopamine’s effects based on dose alone are unpredictable. Low-dose dopamine also implies an unproven beneficial effect on renal function.25–27 In patients receiving dopamine before a “renal insult,” there is a clear diuretic effect but none for improved creatinine clearance or decreased need for hemodialysis.26 Despite drug-induced diuresis, there is no evidence that urine output in the presence of low cardiac output and/or hypovolemia protects renal function. The use of dopamine after the renal insult has occurred has not been shown to improve glomerular filtration rate. There is evidence that the beneficial effect of low-dose dopamine on renal blood flow and glomerular filtration rate observed in healthy individuals is due to drug-induced increases in cardiac output, and this benefit is lost in early renal failure.27–29 No randomized controlled studies have demonstrated a decrease in the incidence of acute renal failure when dopamine is administered to patients considered to be at risk for developing acute renal failure in multiple patient populations (major vascular surgery,30 cardiopulmonary bypass, intensive care,31 heart failure, sepsis,32 transplantation, patients exposed to nephrotoxic drugs33) confirming the results of two large retrospective studies25,26 and two meta-analyses,32,33 finding that dopamine does not prevent or reverse acute renal failure or improve outcome. Aside from one study demonstrating dopamine selectively increases renal blood flow in heart failure patients via dilation of both large conductance and small resistance renal blood vessels,34 there exists no proven improvement in renal perfusion, creatinine clearance, or glomerular filtration rate and no alteration in the course of renal failure or the need for renal replacement therapy.
Dopamine leads to an increase in cortical and inner medullary blood flow, shunting flow from the outer medulla. It increases solute delivery to the distal tubular cells increasing medullary oxygen consumption. The outer medulla is highly metabolically active and highly susceptible to ischemic injury and acute renal failure. Dopamine can induce renal failure in both normo- and hypovolemic patients.
Low-dose dopamine is associated with multiple complications affecting the cardiovascular, pulmonary, gastrointestinal, endocrine, and immune systems. A multicenter observational study demonstrated that dopamine is an independent risk factor for death for patients with shock due to any cause.35 In the absence of data confirming the efficacy of dopamine in preventing acute renal failure, renal-dose dopamine cannot be recommended.25,36 In fact, not only does low-dose dopamine not confer any renal protective mechanism, it may in fact be detrimental.27,37
Cardiovascular Effects
Dopamine is associated more than dobutamine or epinephrine with dose-related sinus tachycardia and the potential to cause ventricular arrhythmias23,38 and may predispose to myocardial ischemia by precipitating tachycardia, increasing contractility, increasing afterload, and precipitating coronary artery vasospasm. Dopamine increases peripheral vascular resistance and pulmonary artery pressures. Unlike dobutamine, dopamine does not inhibit hypoxic pulmonary vasoconstriction. Nevertheless, dopamine is not recommended for use in right heart failure.
Gastrointestinal Effects
Gastrointestinal mucosal ischemia and subsequent translocation of bacteria and bacterial toxins play an important role in the development of multiple organ dysfunction syndrome. Dopamine’s effect on splanchnic blood flow and gastric intramucosal pH is controversial and inconsistent. There is no evidence that low-dose dopamine has beneficial effects on splanchnic function or reduces the progression to multiorgan failure in sepsis. Dopamine may increase flow to the muscular layer of the gut with decreased flow to the mucosal layer with detrimental effects and possibly gut ischemia. In septic patients, dopamine but not norepinephrine, as administered to maintain an acceptable mean arterial pressure, resulted in an uncompensated increase in splanchnic oxygen requirements. Although dopamine infusion in septic patients led to increased hepatosplanchnic perfusion, hepatosplanchnic oxygen uptake was reduced suggesting an impairment of hepatosplanchnic metabolism.40 Most of these studies have looked at the effects of low-dose dopamine rather than doses used to treat hypotension.
D2 receptors are also located in the enteric nervous system. Dopamine agonists interfere with gastrointestinal motility. Low-dose dopamine has been demonstrated to slow gastric motility in mechanically ventilated intensive care patients.41
Endocrine and Immunologic Effects
Dopamine disrupts metabolic and immunologic functions through its effects on hormones and lymphocyte function. The anterior pituitary plays a crucial role in metabolic and immunologic homeostasis. The initial stress response stimulates pituitary hormone release, whereas the chronic phase is associated with suppression of the hypothalamic-pituitary axis. In the acute phase of an illness, dopamine induces the pattern of hypopituitarism seen in prolonged critical illness and chronic stress. When dopamine is used in the chronic phase of illness, it further suppresses the circulating concentrations of pituitary hormones.42
Dopamine depresses the immune status by reducing serum prolactin levels. Prolactin is an immunoregulatory hormone affecting T and B lymphocytes. Dopamine inhibits lymphocyte proliferation, immunoglobulin synthesis, and cytokine production and promotes lymphocyte apoptosis. Dopamine also decreases the secretion of growth hormone, which has anabolic, lipolytic, and immune-stimulating properties. Growth hormone deficiency can contribute to impaired anabolism and a negative nitrogen balance. Dopamine’s inhibition of thyrotropin-releasing hormone leads to “euthyroid sick syndrome.” It also decreases dehydroepiandrosterone sulfate and may affect luteinizing hormone release. Dopamine’s overall effect is to suppress the secretion and function of anterior pituitary hormones, aggravating catabolism and cellular immune function and inducing central hypothyroidism.42
Respiratory Effects
The infusion of low-dose dopamine in healthy subjects as well as heart failure patients interferes with the ventilatory response to arterial hypoxemia and hypercapnia, reflecting the role of dopamine as an inhibitory neurotransmitter at the carotid bodies.43 The result is depression of ventilation in patients who are being treated with dopamine to increase myocardial contractility.44 Dopamine also decreases arterial oxygen saturation by impairing regional ventilation/perfusion matching in the lungs.45 Dose-dependent reductions in arterial PO2 with increasing rates of dopamine in critically ill patients after major surgery have been demonstrated.46 Arterial blood gases have been observed to deteriorate during infusion of dopamine.
One study demonstrated low-dose dopamine did not influence ventilation specifically in patients with chronic obstructive pulmonary disease either breathing spontaneously or being weaned from mechanical ventilation.47 Dopamine may even decrease pulmonary vascular resistance in patients with chronic obstructive pulmonary disease. Other potential beneficial effects are improved respiratory muscle contraction, increased lung edema clearance, and inhibition of bronchoconstriction.47,48 However, such reports are anecdotal or not tested in clinical studies.
Intraocular Pressure
Continuous infusions of dopamine to critically ill patients are associated with increases in intraocular pressure.49 This may create a risk in patients with preexisting glaucoma especially if they are sedated and mechanically ventilated.
Synthetic Catecholamines
The two clinically useful synthetic catecholamines are isoproterenol and dobutamine (see Table 18-1 and Fig. 18-1).
Isoproterenol
Isoproterenol is the most potent activator of all the sympathomimetics with β1 and β2 receptor activity. It is two to three times more potent than epinephrine and at least 100 times more active than norepinephrine. In clinical doses, isoproterenol is devoid of α agonist effects and does not cause the vasoconstriction associated with the naturally occurring catecholamines.
The cardiovascular effects of isoproterenol reflect activation of β1 receptors in the heart and β2 receptors in skeletal muscle and to a lesser extent renal and splanchnic vascular beds. In an adult, continuous infusion of isoproterenol, 1 to 5 µg per minute, greatly increases heart rate, myocardial contractility, and cardiac automaticity, whereas vasodilation in skeletal muscles decreases systemic vascular resistance. Although cardiac output may increase thereby increasing systolic blood pressure, the mean arterial pressure may decrease due to decreases in systemic vascular resistance and associated decreases in diastolic blood pressure. Increases in cardiac output may be attenuated by impaired left ventricular filling due to tachycardia as well as decreased preload from venous vasodilation. There is preferential vasodilation in nonessential areas such as skeletal muscle. Decreased diastolic blood pressure, increased heart rate, and cardiac dysrhythmias may lead to a decrease in coronary blood flow at the same time that myocardial oxygen requirements are increased by tachycardia and increased myocardial contractility.50 Compensatory baroreceptor-mediated reflex slowing of the heart rate does not occur during infusion of isoproterenol because mean arterial pressure is not increased. This combination of events may be deleterious in patients with coronary artery disease.
Compared to dobutamine, for a comparable increase in cardiac output, isoproterenol is associated with larger decreases in total peripheral vascular resistance and blood pressure. It is also associated with more tachycardia both from direct β effects as well as a reflex increase in heart rate with decreased vascular tone.
Metabolism of isoproterenol in the liver by COMT is rapid, necessitating a continuous infusion to maintain therapeutic plasma concentrations. Uptake of isoproterenol into postganglionic sympathetic nerve endings is minimal.
Clinical Uses
A continuous infusion of isoproterenol, 1 to 5 µg per minute, is effective in increasing the heart rate in adults in the presence of heart block. Isoproterenol is used to provide sustained increases in heart rate before insertion of a temporary or permanent cardiac pacemaker in the treatment of bradydysrhythmias. Isoproterenol’s ability to decrease pulmonary vascular resistance may be useful in the management of pulmonary hypertension and right ventricular dysfunction.51 The use of isoproterenol as an inotropic drug has decreased with the availability of inotropic agents such as dobutamine and phosphodiesterase inhibitors. Likewise, the use of isoproterenol as a bronchodilator has been supplanted by the availability of specific β2 agonists.
Adverse Effects
Vasodilation and decreased blood pressure may limit the use of isoproterenol. In addition, it can lead to tachyarrhythmias. The combination of decreased diastolic blood pressure and increased heart rate and dysrhythmias may lead to myocardial ischemia.
Dobutamine
Dobutamine is a synthetic catecholamine derived from isoproterenol consisting of a 50:50 racemic mixture of two stereoisomers.52 The (−) enantiomer is a potent α1-adrenergic agonist as well as a weak β1– and β2-adrenergic agonist. The (+) enantiomer is a competitive antagonist at the α1 receptor site as well as a potent β1– and β2-adrenergic agonist.53 Dobutamine has potent β1-adrenergic effects with weaker β2-adrenergic activity. Its effect on α receptors increases at higher doses. Dobutamine’s cardiovascular effects are a result of the combination of activity of its two stereoisomers.53–56
Dobutamine acts primarily as a positive inotropic agent. Dobutamine leads to an increase in intracellular cAMP, increasing calcium release from the sarcoplasmic reticulum to increase contractility. Cardiac output is increased primarily by an increase in stroke volume. Contractility is increased from its action on myocardial β1 and α1 receptors and, to a lesser extent, by decreased afterload from its effect on vascular smooth muscle β2 receptors. Dobutamine has weak effects on vascular tone causing peripheral vasodilation. At higher doses, the (−) isomer stimulates α1 receptors, limiting further vasodilation. Blood pressure usually is not significantly affected as α1-mediated vasoconstriction by the (−) enantiomer is countered by α1 antagonism by the (+) enantiomer and by its β2 activity, although the latter may be unmasked by β blockade therapy.53–56 In addition, there may be minimal effects on the mean arterial pressure as the increased cardiac output offsets the decrease in peripheral vascular resistance. Because dobutamine does not possess clinically important vasoconstrictor activity, it may be ineffective in patients who require increased systemic vascular resistance rather than augmentation of cardiac output to increase systemic blood pressure.
Dobutamine affects heart rate through its action on β1-adrenergic receptors. Dobutamine stimulates sinoatrial node automaticity as well as atrioventricular nodal and ventricular conduction.38 The chronotropic effects of dobutamine per unit gain in cardiac output are less than that of dopamine and isoproterenol but may be greater than that of epinephrine.52,57,58 The increase in calcium that facilitates increased contractility also facilitates increased arrhythmias. At low doses, increases in heart rate may be minimal. However, high doses of dobutamine (>10 µg/kg/minute IV) may predispose the patient to tachycardia and cardiac dysrhythmias.
Dobutamine increases myocardial oxygen consumption by increasing tachycardia and myocardial contractility. Conversely, dobutamine-induced increases in cardiac output may indirectly lead to a decrease in heart rate in heart failure patients as sympathetic nervous system tone is reduced. Similarly, systemic vascular resistance may decrease by reflex withdrawal of sympathetic tone in addition to dobutamine’s β2 agonist effect. Myocardial oxygen consumption may also be reduced with improved contractility, decreased left ventricular end diastolic pressure (LVEDP), and decreased wall tension.
Dobutamine causes modest reductions in pulmonary arterial pressure and vascular resistance through its effects on β2 receptors. In patients with increased pulmonary artery pressure after mitral valve replacement, an infusion of dobutamine (up to 10 µg/kg/minute) increases cardiac output and decreases systemic and pulmonary vascular resistance.59 Dobutamine inhibits hypoxic pulmonary vasoconstriction. Pulmonary vasodilation may worsen ventilation/perfusion mismatching and may be associated with increased intrapulmonary shunt flow.59
Unlike dopamine, dobutamine does not act indirectly by stimulating the release of endogenous norepinephrine.52 Dobutamine does not activate dopaminergic receptors to increase renal blood flow. Renal blood flow, however, may improve as a result of drug-induced increases in cardiac output.60 Dobutamine but not dopamine is a coronary artery vasodilator. Redistribution of cardiac output in the presence of dobutamine may contribute to increased cutaneous heat loss manifesting as an additional decrease in body temperature.61
Rapid metabolism of dobutamine (half-life of 2 minutes) necessitates its administration as a continuous infusion of 2 to 10 µg/kg/minute to maintain therapeutic plasma concentrations. Tachyphylaxis may occur as it acts on β-adrenergic receptors. Like dopamine, dobutamine should be dissolved in 5% glucose in water for infusion to avoid inactivation of the catecholamine that may occur in an alkaline solution. Dobutamine undergoes biotransformation in the liver to inactive glucuronide conjugates and 3-0-methyldobutamine, most of which is excreted in the urine.62
Clinical Uses
Dobutamine produces potent β-adrenergic agonist effects at doses less than 5 µg/kg/minute IV increasing myocardial contractility (β1 and α1 receptors) and causing a modest degree of peripheral vasodilation (β2 receptors). The levorotatory isomer of dobutamine stimulates α1 receptors at higher doses (>5 µg/kg/minute) limiting further vasodilation. Individual variations exist with dose-dependent effects. Dobutamine is used to improve cardiac output in patients with congestive heart failure.60,63 Dobutamine is also useful for weaning from cardiopulmonary bypass.58 It may be of use in patients with pulmonary hypertension, although its effect on the pulmonary vasculature is less than that of the phosphodiesterase inhibitors. Combinations of drugs may be useful to increase the spectrum of activity and improve the distribution of cardiac output. For example, vasodilators may be combined with dobutamine or dopamine to decrease afterload, optimizing cardiac output in the presence of increased systemic vascular resistance. Most studies demonstrate minimal hemodynamic difference between dopamine and dobutamine use.21 Dobutamine does not have significant venoconstrictor activity compared to dopamine, so increase in ventricular filling pressures may be seen at low doses.
When used in the context of ischemia as opposed to heart failure, the reduction in filling pressures and oxygen requirements from the increased contractility is not offset by the increased tachycardia. Myocardial oxygen consumption is increased by the increasing tachycardia and contractility, whereas coronary blood flow is decreased by vasodilation.62 These properties make dobutamine useful for pharmacologic stress testing to detect potential areas of myocardial ischemia.
Adverse Effects
The use of dobutamine may be limited by the occurrence of tachyarrhythmias, although it is less likely than dopamine or isoproterenol. Sinus tachycardia occurs most commonly, although ventricular arrhythmias may also occur.38 Tachyarrhythmias occur more frequently at higher dosages or in patients with underlying arrhythmias or heart failure. Also, prolonged continuous infusion of dobutamine associated with eosinophilic myocarditis and peripheral eosinophilia have been reported.
Synthetic Noncatecholamines
The commonly used noncatecholamine sympathomimetic drugs are ephedrine and phenylephrine (Fig. 18-5). These will be familiar to every reader.

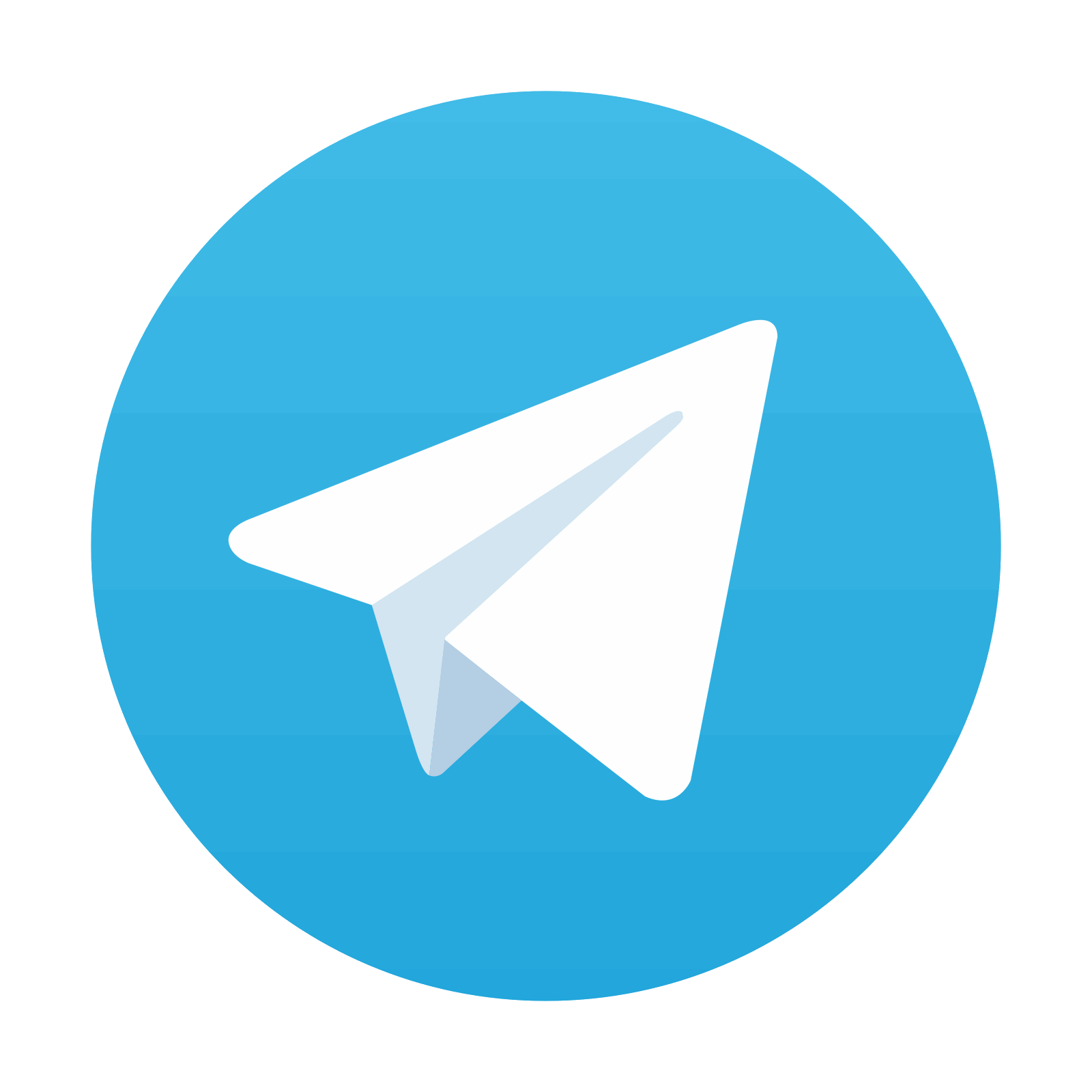
Stay updated, free articles. Join our Telegram channel

Full access? Get Clinical Tree
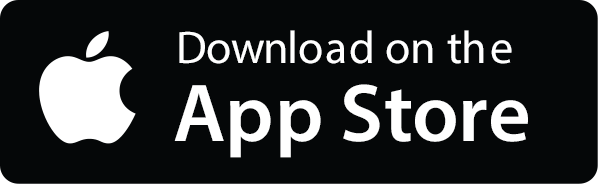
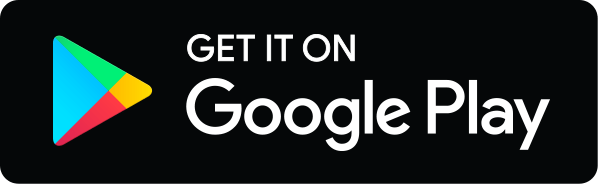