TABLE 84.2 Surviving Sepsis Guidelines for Initial Resuscitation and Infection Control Priorities for Septic Shock and Severe Sepsis |
![]() |
PATHOPHYSIOLOGY OF SURGICAL INFECTIONS
In many respects, the diagnosis and treatment of a surgical infections is fundamentally different than diagnosis of “medical infections.” With nonsurgical infections the diagnostic principles center upon identification of the infecting organism. Generally, there is only one microbial species responsible for “medical” infections. Once identified, the appropriate treatment involves selection of the antimicrobial agent(s) needed to treat this organism. In contrast, the organisms responsible for surgical infections are frequently known, at least in a general sense, based on the anatomic location of the surgical intervention or infection, and knowledge of the organisms present. In the ICU setting, clinicians caring for surgical patients must consider the entire range of surgical, “medical,” and hospital-acquired infectious etiologies in this population.
Resident endogenous bacteria are always present on the skin and throughout the gut. Following admission to the hospital or ICU, the composition of the endogenous flora changes significantly, with a greater proportion of drug-resistant species. Despite the presence of bacteria, humans normally do not become infected because a balance of three factors exists: First, the preponderance of organisms, especially outside the hospital, are nonvirulent bacteria; second, the major reservoirs of micro-organisms (skin and GI tract) are intact; third, host defense mechanisms destroy bacteria that gain access to sterile areas of the body. However, any or all of these factors may be altered by the pre-existing conditions, surgical interventions, nosocomial colonization, indwelling catheters, or prior antibiotic treatment. Knowledge of the community and ICU bacterial flora and the polymicrobial nature of surgical infections informs the selection of antimicrobial agents.
Under normal circumstances, the overwhelming numbers of nonpathogenic bacteria constitute a robust “defense” against infection, because infection is proportionately less likely if >99% of the inoculum is incapable of producing infection. This concept of adherent resident bacteria preventing invasion has been termed colonization resistance (4). As mentioned above, not all microbes are pathogenic, that is, capable of initiating invasion of the body. For example, Escherichia coli (gram-negative aerobe) and Bacteroides fragilis (gram-negative anaerobe) are frequently cultured from intra-abdominal infections (IAIs), yet they constitute 0.01% and 1% of the colonic flora, respectively. These organisms cause infection because they possess virulence factors that favor growth, invasion, or prevent their eradication. This knowledge forms the basis for the selection of empiric antimicrobial therapy, which must have activity against the anticipated pathogens. It has been shown that more than 99% of the colonic microflora are “nonpathogenic” anaerobes on admission to the ICU, but within 3 days the microflora shifts to a much higher proportion of aerobic pathogens (5). Changes in the gut flora within the ICU are harmful in two ways (6): (1) There are a greater proportion of pathogenic and/or resistant bacteria; and (2) there are far fewer nonpathogenic bacteria, organisms which ordinarily suppress overgrowth of aerobic pathogens.
Surgical patients in the ICU have additional factors that put them at increased risk for infection. Postoperative pain and limited mobility, coupled with the lingering effects of general anesthesia, increase the risk for respiratory complications and pneumonia. Vigorous pulmonary toilet, early mobilization (if possible), and adequate analgesia will mitigate, but unfortunately cannot eliminate, this increased risk. Suboptimal nutrition can impair host defenses and innate immunity, and surgical patients often have pre-existing nutritional deficiencies (e.g., in the context of cancer). Postoperative nutritional support is more difficult in surgical patients because of slow return of bowel function coupled with the increased metabolic demand imposed by healing. Patients admitted to the ICU after solid organ transplantation have overt immunosuppression. Immunosuppression and an increased risk of infection have also been well documented in trauma and burn patients, especially those requiring blood transfusions.
The general principles of surgical care, critical care, and infection control cannot be overemphasized. Resuscitation must be rapid, yet precise. Infection prevention measure must sometimes be sacrificed under the chaotic conditions of acute resuscitation, but every attempt should be made so that this is not the case. If central venous catheters (CVCs) were inserted under nonsterile conditions, they must be removed and, if necessary, replaced by a fresh puncture at a new site as soon as the patient’s condition permits. Detailed evidence-based guidelines for the general prevention of infection (7,8) and the prevention of ventilator-associated pneumonia have been published (9). All who provide critical care must be familiar with the guidelines and adhere to them insofar as possible.
APPROACH TO SURGICAL PATIENT WITH SUSPECTED INFECTION
Identification of an ICU infection in surgical and trauma patients is challenging for several reasons. All postoperative and injured patients have a baseline degree of inflammatory response as part of the endogenous reaction to the physiologic insult of surgery and/or the underlying process that required a surgical intervention. Diagnosis of infection is also complicated by the presence of potential signs of infection, such as tachycardia, tachypnea that arise from pain, immobility, or fluid shifts. Elevated temperatures and leukocytosis are also seen commonly for the first few days (<96 hours) after surgery. In the vast majority of new surgical ICU admissions, such early signs and symptoms are not associated with infection. These signs will tend to dissipate with time after the surgical intervention or injury. That said, development of a new fever more than 4 days postoperatively should warrant an investigation for an infectious etiology. A rare, but important, exception to this approach is a very high fever associated with significant systemic toxicity arising in the immediate (<24 hours) postoperative period. Such a presentation can arise from group A β-hemolytic Streptococci or Staphylococcus aureus (toxic shock syndrome); in such a setting it’s important to examine the surgical incisions—which can be diagnostic.
The presence of a new temperature elevation can be a very useful clinical sign in identifying patients with infection. Fever is an elevated temperature above baseline and rises when the hypothalamic “set point” is altered in response to physiologic stimuli. A patient with a core temperature ≥38.3°C (≥101°F) is considered to be febrile and such patients may warrant further evaluation to determine if infection is present. However, the mere presence of fever has a relatively poor positive- and negative-predictive value. It can often be difficult to determine if an abnormal temperature is a reflection of a physiologic process, a drug, or an environmental influence (Table 84.3). It has been noted that fever is due to a noninfectious cause in more than 50% of surgical patients (10,11), and it is now clear that factors released from damaged or ischemic tissue activate the identical toll-like receptors (TLRs) and induce an identical innate immune response with elaboration of pro- and anti-inflammatory cytokines (12,13) as do infectious agents. This may explain why the presence of large amounts of damaged tissue from trauma, burns, acute vascular insufficiency, etc. result in a clinical syndrome that can be indistinguishable from the host response to microbial infection (13). Finally, a minority of patients with impaired immune responsiveness may not manifest fever in response to infection. As a general rule, greater temperature elevations are more likely to arise from a new infection or infectious complication.
TABLE 84.3 Noninfectious Causes of Fever |
![]() |
Performing a careful and focused history and physical examination will assist immeasurably in terms of diagnosing surgical infections. Laboratory tests or imaging studies to search for infection should be performed only after a clinical assessment (history and physical examination) indicates that infection might be present. In postoperative patients, surgical wounds and incisions should be examined. A careful assessment of the respiratory system, including recent alterations in oxygenation or character of sputum, should be done. Examination should focus on identifying the most frequent ICU-acquired infections; catheter-associated urinary tract infection (CA-UTI), pneumonia, and central line–associated blood stream infections (CLABSI), and eliminating noninfectious causes of fever, such as deep vein thrombosis or pulmonary embolism.
Blood cultures should be obtained from patients with a new fever when clinical evaluation suggests an infectious cause (11,14). The site of venipuncture should be cleaned with either 2% chlorhexidine gluconate in 70% isopropyl alcohol, or 1% to 2% tincture of iodine, and the site allowed to dry for at least 30 seconds (15). A 20 to 30 mL sample of blood per culture should be drawn from each single site. Paired blood cultures should always be obtained to avoid false-positive cultures arising from contamination. It is also critical to always try to obtain blood cultures before the initiation of antimicrobial therapy (3). The sensitivity of blood culturing for detection of true bacteremia or fungemia is related to many factors, most importantly, the volume of blood drawn and obtaining the cultures before initiation of anti-infective therapy (16,17). Each culture should ideally be drawn by separate venipuncture or through a separate intravascular device, but not through multiple ports of the same intravascular catheter (18). At least one culture should be drawn from central or longer-term catheters, as differential quantitative bacterial levels may aid in diagnosis of CLABSIs (19,20).
PRINCIPLES OF ANTIBIOTIC THERAPY
Antibiotic Prophylaxis
Widespread application of prophylactic antibiotics, in conjunction with surgical procedures, is common in surgical patients. Nonsurgeons may not be familiar with the appropriate use and goals of prophylactic antibiotics, but it’s important that critical care practitioners understand their use. Antibiotics are used “prophylactically” to prevent infection(s) that could develop in conjunction with an invasive procedure, most often to prevent infection at the site of the surgical procedure (surgical site infections [SSIs]) (21). The goal of antibiotic prophylaxis is to protect the operative site (incision and operative field) during the procedure, when the site is most vulnerable to microbial inoculation. Prophylactic antibiotics are not a panacea; if not administered properly, antibiotic prophylaxis is ineffective and may even be harmful. Antibiotic prophylaxis does not prevent postoperative nosocomial infections, which may occur at an increased rate after prolonged prophylaxis (22), selecting for more resistant pathogens when infection does develop (23).
Surgical wounds can be classified based on the degree to which there is a potential for bacterial contamination (Table 84.4) (21). Antibiotic prophylaxis is indicated for most clean-contaminated and contaminated (or potentially contaminated) operations. Antibiotic prophylaxis of clean surgery is controversial. Where bone is incised (e.g., craniotomy, sternotomy) or a prosthesis is inserted, antibiotic prophylaxis is generally indicated. Some controversy persists with clean surgery of soft tissues (e.g., breast, hernia) (24–27). Lower extremity arterial reconstruction with prosthetic grafts is an example of clean surgery where there is demonstrated benefit of prophylactic antibiotics (28).
Antimicrobial agents for prophylaxis should:
- be a safe agent, as the majority of patients will never develop an infection;
- possess a narrow spectrum of coverage that targets the relevant pathogens that may be present at the surgical site;
- not be an antibiotic relied upon for therapy of established infections; and
- be administered in the optimal manner to prevent infection (29).
The optimal time to give parenteral antibiotic prophylaxis is within 1 hour prior to incision (30). Antibiotics given sooner are ineffective, as are agents given after the incision is closed. The goal is to insure effective concentrations of antibiotic are present in the tissues for the duration of the procedure. Antibiotics with short half-lives (<2 hours, e.g., cefazolin or cefoxitin) should be redosed if the operation is prolonged or bloody (31). Higher doses should be given in obese individuals and patients receiving aggressive resuscitation (e.g., trauma patients). While most guidelines specify a 24-hour limit for prophylaxis, single-dose prophylaxis (with intraoperative redosing, if indicated) has shown equivalency to multiple doses for prevention of SSI (32). Antibiotics should not be given to “cover” indwelling drains or catheters, in lavage or irrigation fluid, or as a substitute for poor surgical technique.
It has now been clearly shown that administration of prophylactic antibiotics beyond 24 hours can be harmful. Prolonged prophylaxis increases the risk of nosocomial infections unrelated to the surgical site, and the emergence of multidrug-resistant (MDR) pathogens. Both pneumonia and vascular catheter–related infections have been associated with prolonged prophylaxis (33,34). Continuation of prophylactic antibiotics resulted in a higher proportion of methicillin-resistant S. aureus (MRSA) isolates from SSI (23). Disruption of the normal balance of gut flora from longer-duration prophylaxis has been shown to result in overgrowth of the enterotoxin-producing Clostridium difficile (35).
TABLE 84.4 Surgical Wound Classification | ||
![]() |
Therapeutic Antibiotics
When considering antimicrobial therapy for surgical infections, it is critical to remember that surgical infections generally require a “surgical” intervention for resolution, resection, debridement, drainage—operative or percutaneous—removal of foreign body, etc. Further, surgical infections are frequently polymicrobial, especially necrotizing skin and soft tissue infection (SSTI) and IAI. Although antimicrobial therapy is the mainstay of some infections, and required as an adjunct to the surgical therapy of others, the widespread overuse—and misuse—of antibiotics has led to an alarming increase in MDR pathogens. New agents may allow shorter courses of therapy and prophylaxis, which are desirable for cost savings and control of microbial flora, but are not always available.
Empiric Antibiotic Therapy
Empiric antibiotic therapy must be administered carefully. Injudicious therapy could result in undertreatment of established infection, or unnecessary therapy when the patient has only inflammation or bacterial colonization; either may be deleterious. Inappropriate therapy—delay, therapy misdirected against usual pathogens, failure to treat MDR pathogens—leads unequivocally to increased mortality (36–39).
Strategies have been promulgated to optimize antibiotic administration, including reliance upon physician prescribing patterns, computerized decision support (40), administration by protocol (41–47), and formulary restriction programs. Owing to the increasing prevalence of MDR pathogens, it is crucial for initial empiric antibiotic therapy to be targeted appropriately, administered in sufficient dosage to ensure bacterial killing, narrowed in spectrum (de-escalation) (48) as soon as possible based on microbiology data and clinical response, and continued only as long as necessary. Appropriate antibiotic prescribing not only optimizes patient care, but also supports infection control practice and preserves microbial ecology (48). Combining these strategies into a unified antibiotic stewardship program has been proven to decrease the incidence of resistant gram-negative health care–associated infections in trauma and surgical ICUs (49).
Choice of Antibiotic
Antibiotic choice is based on several interrelated factors (Table 84.5). Narrow-spectrum coverage is always desired, but the activity of the agent against the identified or likely (for empiric therapy) pathogens is paramount. Estimation of likely pathogens depends on the disease process believed responsible, whether the infection is community-, health care–, or hospital-acquired, and whether MDR organisms are present. Local knowledge of antimicrobial resistance patterns is essential, even at the unit-specific level. Patient-specific factors of importance include age, debility, immunosuppression, intrinsic organ function, prior allergy or other adverse reaction, and recent antibiotic therapy. Institutional factors of importance include guidelines that may specify a particular therapy, formulary availability of specific agents, outbreaks of infections caused by MDR pathogens, and antibiotic control programs.
TABLE 84.5 Factors Influencing Antibiotic Choice |
![]() |
TABLE 84.6 Appropriate Antibacterial Agents for Empiric Use |
![]() |
Numerous agents are available for therapy (Table 84.6) (50–52); these may be chosen based on spectrum, whether broad or targeted (e.g., antipseudomonal, antianaerobic), in addition to the above factors. If a nosocomial gram-positive pathogen is suspected (e.g., wound or SSI, CLABSI, pneumonia) or MRSA is endemic, empiric vancomycin (or linezolid) is appropriate. Some authorities recommend dual-agent therapy for serious Pseudomonas infections (i.e., an antipseudomonal β-lactam drug plus an aminoglycoside), but evidence of efficacy is lacking (53). It is important to use at least two antibiotics for empiric therapy of any infection that may be caused by either a gram-positive or gram-negative infection (e.g., nosocomial pneumonia) (54).
Role of Antifungal Therapy
The incidence of invasive fungal infections is increasing among critically ill surgical patients. Several conditions are predictors for invasive fungal infection complicating critical illness, including ICU length of stay; altered immune responsiveness; and the number of medical devices placed. Neutropenia, diabetes mellitus, new-onset hemodialysis, total parenteral nutrition, broad-spectrum antibiotic administration, bladder catheterization, azotemia, diarrhea, and corticosteroid therapy are also associated with invasive fungal infection (55,56).
Duration of Antimicrobial Therapy
The endpoint of therapy is largely undefined, in part because quality data are few (41,57,58). If cultures are negative, empiric antibiotic therapy should be stopped in most cases. Unnecessary antibiotic therapy in the absence of infection clearly increases the risk of MDR infection; therefore, therapy beyond 48 to 72 hours with negative cultures usually is unjustifiable. The morbidity of antibiotic therapy includes allergic reactions, development of nosocomial superinfections—fungal, enterococcal, and C. difficile–related infections—organ toxicity, promotion of antibiotic resistance, reduced yield from subsequent cultures, and induced vitamin K deficiency with coagulopathy or accentuation of warfarin effect.
If bona fide evidence of infection is evident, then treatment is continued as indicated clinically. Some infections can be treated with therapy lasting 5 days or less. Every decision to start antibiotics must be accompanied by a decision regarding the duration of therapy (59); a reason to continue therapy beyond the predetermined endpoint must be compelling. Bacterial killing is rapid in response to effective agents, but the host response may not subside immediately. Therefore, the clinical response of the patient should not be the sole determinant for continuation of therapy. If a patient still has systemic inflammatory response syndrome (SIRS) at the predetermined end of therapy, it is more useful to stop therapy and obtain new cultures to look for persistent or new infection, resistant pathogens, and noninfectious causes of SIRS. Seldom should antibacterial therapy continue for more than 7 to 10 days. This concept was analyzed in the Study to Optimize Peritoneal Infection Therapy (STOP-IT) trial published in the NEJM (60). The two study arms consisted of antibiotic treatment until 2 days after resolution of fever, leukocytosis, and ileus for maximum of 10 days (control group) versus a fixed, short course of antibiotics of 4 ± 1 days (experimental group). The primary outcomes of SSI, recurrent IAI, or death were not different between the experimental group (21.8%) and the control group (22.3%) (95% confidence interval [CI], −7.0 to 8.0; p = 0.92). However, there was a significant difference in the median duration of antibiotic therapy of 4.0 days [4.0, 5.0] in the experimental group versus 8.0 days [5.0, 10.0] in the control group (95% CI, −4.7 to −3.3; P < 0.001). These results suggest that after an adequate source control procedure, the beneficial effects of systemic antimicrobial therapy are limited to the first few days after intervention. Further, the delay in manifestation of infectious complications in the control group increases the overall time to resolution of all infections. Implementation of this strategy in patients with an IAI who have had adequate source control can effectively cut the duration of antibiotics by half. Keeping this in mind, there are some bacterial infections that require more than 14 days of therapy such as tuberculosis of any site, endocarditis, osteomyelitis, and selected cases of brain abscess, liver abscess, lung abscess, postoperative meningitis, and endophthalmitis.
SPECIFIC CLINICAL SYNDROMES
Surgical Site Infections
According to criteria established by the United States Centers for Disease Control and Prevention (CDC), an SSI is an infection related to an operative procedure that involves only the skin and subcutaneous tissue of the incision. Additionally, the patient must have pain, tenderness, localized swelling, erythema, or heat AND at least one of the following: (1) purulent drainage from the superficial incision; (2) organisms identified from an aseptically obtained specimen from the superficial incision or subcutaneous tissue by a culture or nonculture-based microbiologic testing method which is performed for purposes of clinical diagnosis or treatment; or (3) superficial incision that is deliberately opened by a surgeon and culture or nonculture-based testing is not performed. It is important to remember that a culture- or nonculture-based test that has a negative finding does not meet the criterion for SSI (61). SSIs are classified generally as superficial SSI, deep SSI, and organ space SSI (Fig. 84.1) (21).

FIGURE 84.1 Classification of surgical site infections by depth of invasion or location. (Redrawn from Horan TC, Gaynes RP, Martone WJ, et al. CDC definitions of nosocomial surgical site infections, 1992: a modification of CDC definitions of surgical wound infections. Infect Control Hosp Epidemiol. 1992;13:606–608.)
Pathogenesis of Surgical Site Infection
SSIs are among the most frequently encountered complications in surgical patients, regardless of specialty. Although any patient undergoing an operation is at risk for developing an SSI, rates vary according to the type of operation, with the highest rate seen with abdominal surgery (62). In the 2013 report by the National Healthcare Safety Network (NHSN), coagulase-negative staphylococci and S. aureus were the most prevalent SSI pathogens for most types of surgery, whereas SSI occurring from abdominal operations had a predominant gram-negative rod flora (63).
The spectrum of bacterial contamination of the surgical site has been well described (64). The vast majority of SSI arises from the patient’s endogenous organisms, typically those present at the site of the procedure. Clean surgical procedures affect only integumentary and musculoskeletal soft tissues. Clean-contaminated procedures open a hollow viscus (e.g., alimentary, biliary, genitourinary, respiratory tract) under controlled circumstances (e.g., elective colon surgery). Contaminated procedures involve extensive introduction of bacteria into a normally sterile body cavity, but too briefly to allow infection to become established during surgery (e.g., penetrating abdominal trauma, enterotomy during adhesiolysis for mechanical bowel obstruction). Dirty procedures are performed to control established infection (e.g., colon resection for perforated diverticulitis).
Numerous factors determine whether a patient will develop an SSI, including factors related to the patient, the environment, and the therapy (Table 84.7) (65). As incorporated in the NHSN (66,67), the most recognized factors are the wound category (see Table 84.4), the American Society of Anesthesiologists (ASA) Class ≥ 3 (class 3: chronic active medical illness), and prolonged operative time (procedural time longer than the 75th percentile for each such procedure). According to the NHSN, the risk of SSI increases with an increasing number of risk factors present, irrespective of the type of operation (64). Laparoscopic surgery decreases the incidence of SSI under most circumstances (14) for several reasons, including decreased wound size; limited use of cautery in the abdominal wall; and a diminished stress response to tissue injury.
TABLE 84.7 Risk Factors for Development of Surgical Site Infections |
![]() |
Host-derived factors contribute importantly to the risk of SSI. Identified risk factors include increased age (14), obesity, malnutrition, diabetes mellitus (4,68), hypocholesterolemia (69), and numerous other factors that are not accounted for specifically by the NHSN system. In one 6-year study of 5,031 patients undergoing noncardiac surgery, the overall incidence of SSI was 3.2% (70). Independent risk factors for the development of SSI included ascites; diabetes mellitus; postoperative anemia; and recent weight loss, but not chronic obstructive pulmonary disorder, tobacco use, or corticosteroid use. In another prospective study of 9,016 patients, 12.5% of patients developed an infection of some type within 28 days after surgery (71). Multivariable analysis showed that decreased serum albumin concentration, increased age, tracheotomy, and amputations were associated with an increased probability of an early infection. Factors associated with readmission due to infection included a dialysis shunt, vascular repair, and an early infection. The 28-day mortality was most influenced by advanced age, low serum albumin concentration, elevated serum creatinine concentration, and early SSI.
In the modern operating room, a lapse in sterile technique has also been shown to increase the rate of SSI. Proper sterilization, ventilation, and skin preparation techniques require continuous vigilance. The operating team must also be attentive to the patient’s personal hygiene, as well as their own (e.g., hand scrubbing, hair). Recent data indicate that a brief rinse with soap and water followed by use of an alcohol gel hand rub was equivalent to the prolonged (and ritualized) session at the scrub sink (72). Although this study only yielded Level III evidence, it demonstrated the importance of reviewing the performance of the operating room personnel (including surgeons) and implementing educational strategies if protocol breaches were observed (73).
Broadly speaking, a number of “environmental” factors also have been shown to impact the development of SSI. Hypothermia during surgery is common if patients are not warmed actively, owing to evaporative water losses, administration of room temperature fluids, and other factors (74). Maintenance of normal core body temperature is unequivocally important for decreasing the incidence of SSI. Mild intraoperative hypothermia is associated with an increased rate of SSI following elective colon surgery and diverse operations (75,76). The role of supplemental oxygen in the postoperative period had previously been shown to improve wound healing (77,78), but the role of oxygen on SSI had received much less study. Oxygen has been postulated to have a direct antibacterial effect (78) and it is also important for neutrophil-mediated bacterial killing. However, clinical trials have had conflicting results (79,80). The ischemic milieu of fresh surgical incisions are potentially vulnerable to bacterial invasion. Supplemental oxygenation administration specifically to reduce the incidence of SSI remains plausible, and further studies are needed.
Closure of a contaminated or dirty incision is widely believed to increase the risk of SSI, but few good studies exist to help sort out the multiplicity of wound closure techniques available to surgeons. “Open abdomen” techniques of temporary abdominal closure for management of trauma or severe peritonitis are utilized increasingly. Retrospective studies indicate that antibiotics are not indicated for prophylaxis of the open abdomen, but if infection of the abdominal wall occurs, it is associated with a very high morbidity (81). Inability to achieve primary abdominal closure has been associated with several infectious complications (pneumonia, bloodstream infection, and SSI). Infectious complications, in turn, significantly increased costs from prolonged length of stay, but not mortality (82).
Drains placed in incisions probably cause more infections than they prevent. Drains prevent epithelialization and can become a portal for invasion by pathogens colonizing the skin. Several studies of drains placed into clean or clean-contaminated incisions show that the rate of SSI is not reduced (83,84) and other investigations showed that the incidence of SSI rate was increased (85–88). The preponderance of data supports the concept that drains should be used as little as possible and removed as soon as possible (89). Under no circumstances should prolonged antibiotic “prophylaxis” be administered to “cover” indwelling drains.
Clinicians continue to study modifications of old methods and the utility of new modalities to impact SSI. The use of wound irrigation to reduce the risk of SSI is highly controversial. Routine low-pressure saline irrigation of an incision does not reduce the risk of SSI (90), but lavage with dilute povidone–iodine was reported to reduce the risk of SSI compared with nonantiseptic lavage (91). An increasing body of the literature suggests that intraoperative topical antibiotics can minimize the risk of SSI (92–94), but the use of antiseptics, rather than antibiotics, may minimize the development of resistance. Another recent intervention aimed at reducing SSI is application of negative-pressure wound therapy (NPWT) to closed incisions. Although the evidence for the use of NPWT for the management of open wounds has been described, the data for its use in closed surgical incisions is limited and general recommendations cannot be made at this time, although one meta-analysis did find that closed surgical incision NPWT reduced postoperative wound complications (95).
Diagnosis of Surgical Site Infection
SSI remains largely a clinical diagnosis. Presenting signs and symptoms depends on the depth of infection, typically as early as postoperative day 4 or 5. Clinical signs range from local induration only, to the hallmarks of infection: erythema, edema, tenderness, warm skin, and pain-related immobility. Any or all of these signs may precede wound drainage. In cases of deep incisional SSIs, tenderness may extend beyond the margin of erythema, and crepitus, cutaneous vesicles, or bullae may be present (96). With ongoing infection, the signs of the SIRS herald the development of sepsis. For infections that involve intracavitary space (organ/space), symptoms and signs that are specific to that organ system usually predominate, such as prolonged postoperative ileus, persistent respiratory distress, or altered neurologic status.
Cultures are not mandatory for management of superficial incisional SSIs, particularly if drainage and wound care alone will suffice without antibiotics. In cases of deeper infection or infection that has arisen in the hospital, exudates or drainage specimens should be sent for analysis. Culturing the surgically opened wound (as opposed to the already opened wound, which becomes colonized) by the swab method has been shown to be reliable. Aspiration of a sufficient volume of purulent material using an aseptic technique yields better pathogen identification that swab culturette (21). Computed tomography (CT) and magnetic resonance imaging (MRI) are more sensitive in detecting small amounts of gas in soft tissues than plain radiographs, and CT-guided aspiration or drainage often facilitates treatment, and may serve as definitive source control for an organ/space SSI.
Treatment of Surgical Site Infection
Local surgical control of the infection remains the crucial aspect of therapy, oftentimes by simply opening and draining the incision in cases of superficial incisional SSI. Infections extending below the superficial fascia (deep incisional SSIs) invariably require formal surgical debridement and open wound care to resolve the infection. Vacuum-assisted closure (VAC) and antimicrobial therapy also improved outcomes, but MDR pathogens may complicate resolution of ostensibly simple infections in the postoperative period (97), especially among patients hospitalized for a period before surgery, particularly if they required antimicrobial therapy.
When faced with a potential SSI, the first steps in management are to remove the appropriate sutures/staples, open and examine the suspicious portion of the incision, and decide about further surgical treatment (98). If the infection is not confined to the skin and superficial underlying subcutaneous tissue, urgent surgical exploration and debridement are essential to obtain local control of the infection, remove necrotic tissue, and restore aerobic conditions to prevent further spread of the infection. SSI must also be considered the cause of delayed or failed wound healing and prompt the same decisions as described above (98).
Superficial SSIs (functionally subcutaneous abscesses) rarely lead to systemic infection and usually do not make patients seriously ill. Antibiotic therapy is not indicated for patients who do not have systemic signs of infection. If infection extends to superficial fascia or beneath, or extensive tissue necrosis and liquefaction beyond the obvious limits of the cutaneous signs, there is the possibility of a necrotizing infection and such wounds may necessitate formal exploration in the operating room. Necrotizing soft tissue infections (NSTIs) are true emergencies that need immediate surgical attention. Even modest delays can increase patient mortality substantially. Freischlag et al. (99) showed that mortality increased from 32% to 70% when therapy was delayed more than 24 hours. With an established diagnosis of NSTI, immediate and widespread operative debridement is indicated without waiting for precise determination of the causative pathogen or the identification of a specific clinical symptom. These patients often require planned, sequential, repetitive surgical debridement sessions to control the infection. Broad-spectrum antimicrobial therapy should be given empirically to cover likely pathogens and de-escalated following microbiology speciation.
Organ/space SSIs occur within a body cavity, are directly related to a surgical procedure, and may manifest as intra-abdominal, intrapleural, or intracranial infections. They may remain occult or present with symptoms that mimic incisional SSIs, leading to inadequate initial treatment. Occasionally, organ/space SSI only become apparent when a major complication ensues. The diagnosis of organ/space SSI usually requires some form of imaging (CT, ultrasound, MRI) to confirm the site and extent of infection; adequate source control requires a drainage procedure, whether open or percutaneous.
Experimentally, the value of VAC was first appraised by Morykwas et al. (100) in a swine model in 1997. VAC optimizes blood flow, decreases tissue edema, and removes fluid from the wound bed, thereby facilitating the removal of bacteria from the wound. Mechanical deformation of the wound promotes tissue expansion to cover the defect, and subatmospheric pressure in the milieu may trigger a cascade of intracellular signals that increases the rate of cell division and formation of granulation tissue (101). The clinical value of VAC systems has been described only in small case series and cohort studies, mostly for sternal infections following cardiac surgery, abdominal wall dehiscence, management of complex perineal wounds, or as a method to secure skin grafts (102,103). The absence of well-designed randomized controlled trials precludes more specific recommendations.
SINUSITIS
The paranasal sinuses are normally sterile, but bacterial overgrowth occurs when drainage is impeded. The etiologic agents responsible for most cases of nosocomial sinusitis are those that colonize the naso-oropharynx at high frequency among critically ill patients (104,105). Gram-negative bacilli (particularly Pseudomonas aeruginosa) constitute 60% of bacteria isolated from nosocomial sinusitis, whereas gram-positive cocci (typically S. aureus and coagulase-negative staphylococci) comprise one-third of isolates, and fungi the remaining 5% to 10% (104,106,107). Sinusitis acquired while in the ICU is most often polymicrobial.
Pathogenesis of Sinusitis
In the ICU, nosocomial sinusitis is an uncommon closed-space infection that is often clinically occult, but that can have serious consequences (108). Although sinusitis should always be part of the differential diagnosis of fever, the incidence is low in comparison to other nosocomial infections in the ICU and the diagnosis can be difficult. The likely pathogenesis of sinusitis is anatomic obstruction of the ostia draining the facial sinuses, especially the maxillary sinuses. Transnasal endotracheal intubation is the leading risk factor, with an incidence of sinusitis estimated to be about 33% after 7 days of intubation. Maxillofacial trauma, with obstruction of drainage by retained blood clots, is another clear risk factor. Nasogastric intubation, nasal packing for epistaxis, and corticosteroid therapy have also been implicated, but the evidence is less convincing.
Diagnosis of Sinusitis
Nosocomial sinusitis is a dangerous, closed-space infection that is increasing in incidence, is difficult to diagnose, and is, therefore, controversial as to its actual incidence and importance (109). Complaints of facial pain or headache may be impossible to elicit and purulent nasal discharge is present in only 25% of proved cases of sinusitis. In the ICU, acute sinusitis is diagnosed most efficiently by CT of the facial bones, followed by sampling using antiseptic technique if mucosal thickening or sinus fluid is documented (110). Microbial analysis of fluid obtained by minimally invasive sinus puncture and aspiration under antiseptic conditions is definitive for the diagnosis. Although less well studied, endoscopic-guided middle meatal tissue culture is a safe alternative for patients who are not candidates for antral puncture (e.g., coagulopathy) (111). Pathogen identification and susceptibility testing permit focused, narrow-spectrum antimicrobial therapy. However, specimen collection is susceptible to contamination by bacteria colonizing the overlying mucosa if rigorous antisepsis is not practiced when obtaining the specimen.
Treatment of Sinusitis
Sinusitis should be suspected in any patient with sepsis, particularly if initial cultures (e.g., blood, sputum, urine, indwelling vascular catheters) are unrevealing. When sinusitis is suspected, the diagnosis is confirmed by maxillary antral tap, lavage, and culture using aseptic technique. Gram-positive cocci, gram-negative bacilli (including P. aeruginosa), and fungi (incidence, 8%) are possible pathogens, so initial therapy should be directed at these pathogens, based on local susceptibility patterns. Most antibiotics achieve adequate tissue penetration, so treatment consists of antibiotic therapy, removal of tubes blocking the ostia, and mobilization of the patient (112). The optimal duration of therapy is unknown, so treatment is most often determined by the clinical response. Refractory cases may require repetitive lavage of the sinus, or a more formal drainage procedure.
The role of nasal decongestant agents (NDCA) in the prevention of infectious nosocomial sinusitis has not been convincingly proven. However, one prospective randomized trial in patients at high risk for sinusitis demonstrated a reduction in radiologic sinusitis in those treated with locally applied NDCA and corticosteroids (113). Investigations into prevention should be continued as sinusitis is a predisposing factor for VAP, and may be a source of lower respiratory tract pathogens. There is 85% concordance between pathogens of sinusitis and pneumonia in patients who develop VAP subsequently, lending credence to the hypothesis that purulent sinus drainage inoculates the lower airway.
CENTRAL LINE–ASSOCIATED BLOODSTREAM INFECTION
Critically ill patients often require reliable large-bore central venous access (e.g., femoral, internal jugular, or subclavian vein), but the catheters are highly prone to infection. Strict adherence to infection control and proper insertion technique are crucial for prevention (114), because surgical, and especially trauma, patients are at high risk. When placed under elective (controlled) circumstances, optimal insertion technique includes chlorhexidine skin preparation (not povidone–iodine) (115), draping the entire bed into the sterile field, and donning a cap, a mask, and sterile gown and gloves (116). If there is a break in sterile technique, the risk of infection increases exponentially. Catheters placed emergently without sterile precautions should be removed and replaced (if still needed) at a different site using strict asepsis and antisepsis as soon as the patient’s condition permits, but certainly within 24 hours. Infection risk for femoral vein catheters is the highest, whereas catheters placed via the subclavian route have the lowest rates of infection (81). Peripheral vein catheters, peripherally inserted central catheters (PICCs), and tunneled CVCs (e.g., Hickman, Broviac) pose less risk of infection than percutaneous CVCs (117). Information campaigns, educational initiatives (118), and strict adherence to insertion protocols have all been shown to be effective in decreasing the risk of CLABSI. The role of antibiotic- and antiseptic-coated catheters is controversial, but they may help decrease the risk of infection in units that have CLABSI rates exceeding the National Healthcare Safety Network surveillance data targets (119,120).
Pathogenesis of CLABSI
The pathogens isolated in CLABSI are predominantly gram-positive cocci, most commonly methicillin-related Staphylococcus epidermidis (MRSE), MRSA, and enterococci. Unfortunately, MRSE is both the most common cause of CLABSI and the most common cause of false-positive blood cultures because of contamination during the collection process. As noted in the section on evaluation of surgical patients with suspected infection, it’s critically important to obtain sufficient volume for blood cultures, to obtain samples from multiple sites, and to obtain at least one culture from any central line (11).
Diagnosis of CLABSI
The diagnosis of CLABSI is usually a diagnosis of exclusion. However, in a patient with bacteremia or fungemia with erythema and purulence at the site of central line insertion, the central line can be considered as the source. Another early sign of CLABSI can be the inability to aspirate from the line, as the infected line becomes more thrombogenic. Most authorities consider the isolation of MRSE from a single blood culture to be a contaminant and do not treat, especially if the patient has no indwelling hardware that might become infected secondarily (e.g., prosthetic joint or heart valve). Gram-negative bacillary pathogens are less common (but seldom are contaminants), and fungal CLABSIs are less common in surgical patients than medical patients.
Treatment of CLABSI
The initial treatment for CLABSI involves removal of the suspected catheter (for peripheral or percutaneous CVCs) and parenteral antibiotics. It is not clear whether a positive catheter culture requires therapy beyond catheter removal, absent local signs of infection, or a true-positive blood culture. The historic practice of performing routine guidewire exchange at predefined intervals does not decrease the rate of CLABSI and may even increase the incidence. Catheter-related bloodstream infections caused by S. aureus requires at least 2 weeks of therapy, although some authorities argue for a longer course (4 to 6 weeks) because of the risk of metastatic infection (e.g., pneumonia, endocarditis). Vancomycin or linezolid is appropriate choice for MRSA (or MRSE when treatment is indicated), with daptomycin as an alternative. Therapy for enterococcal or gram-negative should be dictated by bacterial susceptibility, with no clear consensus as to duration of therapy. Beyond removal of the catheter, treatment of fungal CLABSI is controversial; some authorities recommend at least 2 weeks of systemic antifungal therapy.
C. DIFFICILE–ASSOCIATED DISEASE (CDAD)
C. difficile–associated disease, formerly pseudomembranous colitis, develops because antibiotic therapy disrupts the balance of colonic flora, allowing the overgrowth of C. difficile, present in the fecal flora of about 3% of normal hosts. Any antibiotic can induce this selection pressure, even when given appropriately as surgical prophylaxis, although clindamycin, third-generation cephalosporins, and fluoroquinolones have a predilection (121). Paradoxically, even antibiotics used to treat CDAD (e.g., metronidazole) have been associated with CDAD.
Pathogenesis of CDAD
CDAD is unquestionably a nosocomial infection. Spores can persist on inanimate surfaces for prolonged periods, and pathogens can be transmitted from patient to patient by contaminated equipment (e.g., bedpans, rectal thermometers) or on the hands of health care workers. The alcohol gel that is used increasingly for hand disinfection is not active against spores of C. difficile; therefore, hand washing with soap and water is necessary when caring for an infected patient, or generally during outbreaks.
Diagnosis
The clinical spectrum of CDAD is wide, ranging from asymptomatic (8% of affected patients do not have diarrhea) to life-threatening transmural pancolitis with perforation and septic shock. The typical patient will have fever, abdominal distention, copious diarrhea, and leukocytosis. Colon hemorrhage is rare, and if observed should prompt an alternative diagnosis.
Although there is more than one method for detecting C. difficile, the nucleic acid amplification tests (NAAT) for C. difficile toxin genes such as PCR are superior to toxins A+B EIA testing as a standard diagnostic test for CDI (122). Most strains of C. difficile produce toxin A, but 2% to 3% of stains produce only toxin B, so an assay that detects both toxins A and B is preferred (123). Cultures for C. difficile are technically demanding, and are not specific in distinguishing toxin-positive strains, toxin-negative strains, and asymptomatic carriage (121,124). Cultures may be useful in the setting of nosocomial outbreaks to identify isolates for epidemiologic purposes (125). The North American pulse-field gel electrophoresis type 1 (NAP1) strain, now epidemic in many hospitals in the United States, Canada, and Europe, is associated with serious complications (toxic megacolon, leukemoid reactions, septic shock, and death) (126,127). Direct visualization of pseudomembranes is nearly diagnostic of CDAD, but only about 70% of seriously ill patients and 25% of patients with mild disease have pseudomembranes by direct visualization (128), diminishing the role of endoscopy for routine diagnostic use. However, a role for direct visualization may exist if false-negative C. difficile toxin assays are suspected (125).
Treatment of CDAD
Treatment of mild cases consists of withdrawal of the putative offending antibiotic. Oral antibiotic therapy is often prescribed, but may or may not be necessary. More severe cases may require parenteral metronidazole or oral or enteral vancomycin (by gavage or enema, if ileus precludes oral therapy); parenteral vancomycin is completely ineffective. Some patients with severe disease may require a colectomy, usually a total abdominal colectomy (127). Colectomy for severe disease has traditionally been the only surgical option for CDAD that is unresponsive to medical therapy. However, more recent reports have shown that minimally invasive surgical techniques coupled with antegrade colonic lavage is associated with improved outcomes (129). In patients with nonfulminant CDAD, a loop ileostomy is formed and the colon is lavaged with warm polyethylene glycol intraoperatively, while postoperative therapy is completed using vancomycin instilled through the colon via the ileostomy. While there are no large trials that demonstrate efficacy, one small trial reported a significant improvement in mortality and colon salvage in the ileostomy and antegrade colonic lavage group compared to historic controls (129). While these early data are promising, questions still remain regarding patient selection and optimal timing of the operation, but is currently not recommended in patients with fulminant or perforated disease (130).
The use of fecal microbiota transplantation (FMT) given to patients with CDAD to repopulate the normal colonic flora is also gaining in popularity as a therapeutic option. Small, retrospective analyses have identified increased cure rates in patients with recurrent CDAD that were treated with FMT and one prospective clinical trial comparing combinations of antibiotics (vancomycin), colonic lavage, and FMT was stopped early because of the superiority of cure rates within the FMT group (131). The delivery of FMT has been a rate-limiting step in its widespread use, but there are currently investigations into delivery via a pill form.
The prevalence of severe disease has increased markedly with the emergence of a new strain of C. difficile. This strain has a mutant gene that suppresses toxin production, such that far more toxin is elaborated, resulting in clinically severe disease (126). As this disease continues to evolve, the roles of traditional antibiotic therapy and the role of novel surgical and medical (i.e., FMT) treatments will continue to evolve as well.
PERIANAL ABSCESS AND INFECTION
Perianal abscesses and/or infection, although rare, can occur in the ICU setting. Critical care practitioners should be familiar with perianal infection for several reasons:
- Such infections can easily be missed if clinicians do not have a high index of suspicion and institute a directed examination of the anus and surrounding area.
- Perianal infection is frequently missed by diagnostic modalities employed during workup of sepsis or fever.
- Perianal infections are seen more frequently in immunosuppressed patients.
- These infections can arise in the setting of diarrhea or suboptimal perineal hygiene in immobilized ICU patients.
- Frequently these infections can be treated with relatively simple bedside drainage procedures, although more extensive infections may require formal operative or percutaneous drainage interventions.
Pathogenesis of Perianal Infections
Perianal infections usually arise from the mucosa secondary to obstruction of an anal gland (132,133). In a minority of patients, perianal infections arise from the anoderm or surrounding skin. Immunosuppressed leukemic or bone marrow transplantation patients have been noted to be at increased risk for these infections. In a report of 1,102 patients with leukemia, 6.7% were found to have perianal infection (134); furthermore, perianal abscesses can develop even in the presence of profound granulocytopenia (135).
Overall, the organisms responsible for perianal infection may be changing. Classically, these infections are polymicrobial and arise from endogenous enteric bacteria (132). However, more recent reports show that as many as 37% of ambulatory patients with perianal abscess grew MRSA when the wounds were cultured (136). Perianal infections caused by MRSA were more likely to be associated with extensive induration and erythema on examination, and scant purulence with drainage. The microbiology of perianal infection in immunosuppressed patients also showed a much higher proportion of gram-negative pathogens, including E. coli, Bacteriodies, Enterococcus, and Klebsiella, than seen in the nonhospitalized population (135).
Diagnosis of Perianal Infections of Perianal Infections
Once identified, the treatment of perianal infections is relatively straightforward. Physical examination is usually sufficient to make the diagnosis, but ultrasound, CT, or MRI may be useful adjuncts in patients with deep-seated infections, ischiorectal infection, or to completely characterize complex fistula (137). Confusion can be avoided when describing the location of an abscess near the rectum by employing standard anatomic terminology, such as right, left, anterior, and posterior—rather than referring to the position of a “clock face” (132).
Treatment of Perianal Infections
Once identified, the treatment of perianal infections is relatively straightforward. Drainage is the mainstay of treatment for perianal abscess (132,133,137). Untreated perianal infections frequently result in development of fistula-in-ano, a complication that may progress to necrotizing perineal infections. Whenever possible, incisions to drain the abscess should be placed as close to the anus as possible, to minimize the impact if an anorectal fistula develops. In the ambulatory population, there’s little role for culturing these infections, but cultures should be obtained in ICU setting because of the dramatically altered bacterial flora present in these patients. Antibiotics should also be considered with MRSA infections or if patients have extensive cellulitis (137). Drainage can usually be performed at the bedside under local anesthesia, but some patients may need to be transported to the operating room, or may require more extensive surgical drainage procedures. The role of packing is controversial; practice guidelines from the American Society of Colon and Rectal Surgeons do not recommend routine packing (137). In a small prospective randomized study, no packing of drained perianal abscesses was associated with more rapid resolution of the infection (138).
Key Points
- Surgical patients have multiple sites for potential infection.
- Stresses of surgery plus critical illness often result in significant malnutrition and/or alterations in glucose homeostasis.
- Surgical infections are often polymicrobial, with community organisms during first 72 hours and hospital organisms after 3 days.
- Surgical infections often require an intervention (surgical, procedural, percutaneous), in addition to antibiotics, for resolution.
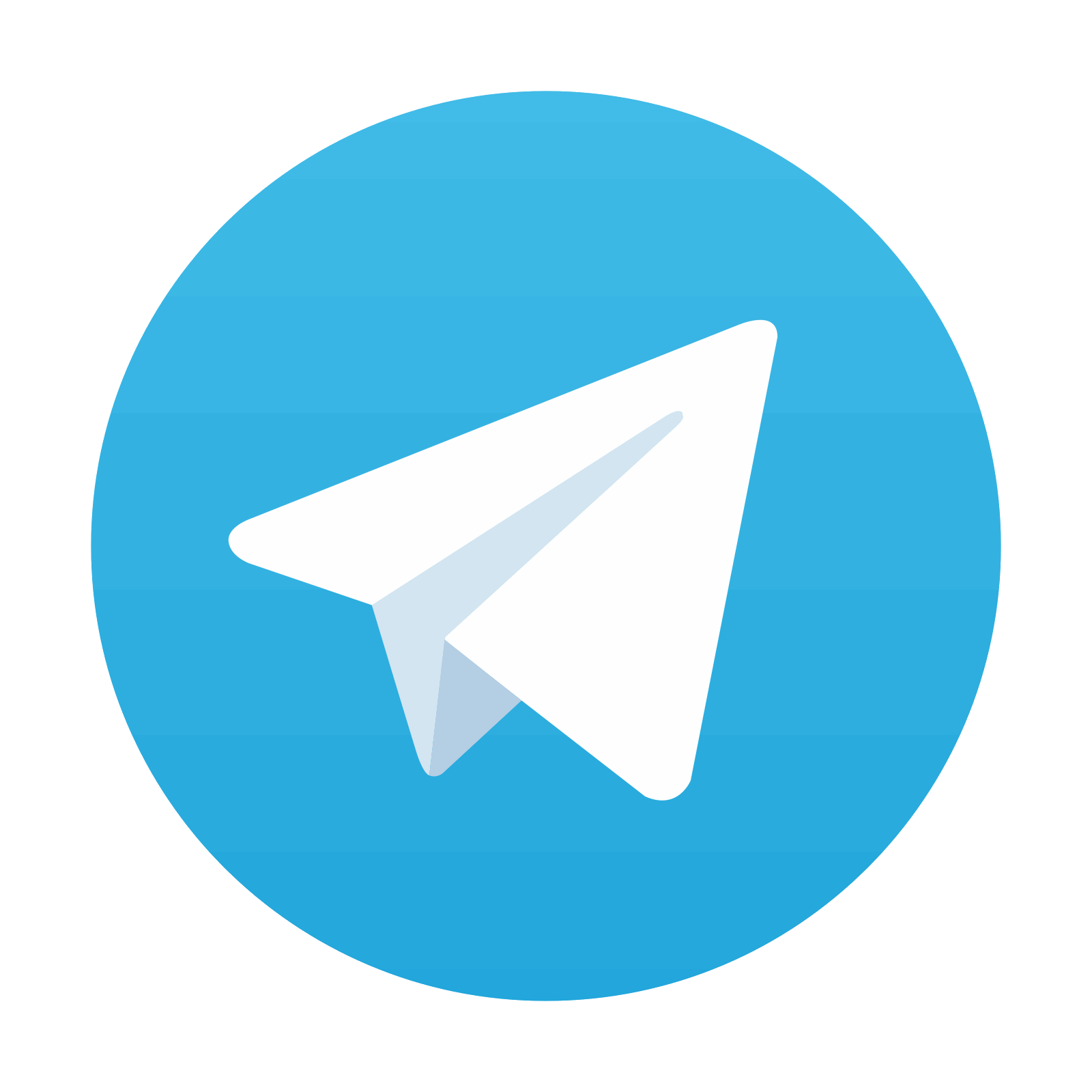
Stay updated, free articles. Join our Telegram channel

Full access? Get Clinical Tree
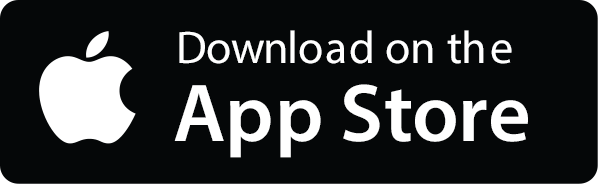
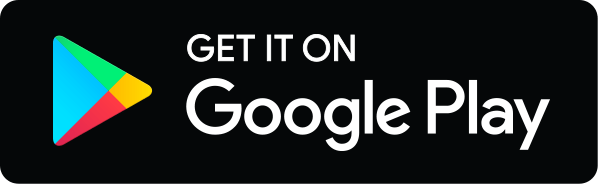