Status Asthmaticus
Michael T. Bigham
Richard J. Brilli
KEY POINTS
Epidemiology
Mechanism of Disease: Core Pathophysiology

Clinical Presentation and Differential Diagnosis





Asthma is the most common chronic disease of childhood and accounts for nearly 155,000 pediatric hospitalizations each year (1). Although the prevalence of asthma has reached a plateau, asthma-related mortality remains high. Consequently, national organizations continue to update guidelines that describe optimal treatment for this common childhood illness (2). A description of the pathophysiology, clinical presentation, and current treatment for children hospitalized in the PICU with status asthmaticus is the focus of this chapter.
DEFINITION
The word “asthma” is derived from the Greek verb “aazein,” meaning to exhale with open mouth, to pant. The first recorded use of this word was 2700 years ago in Homer’s Iliad, where it was used to describe hard breathing or the shortdrawn breaths of a warrior who died after a furious battle. Asthma was first used in a clinical context by Hippocrates (circa 460-360 BC) in describing respiratory findings, such as dyspnea, tachypnea, and orthopnea. The best clinical description of asthma in antiquity was by the Greek physician Aretaeus, ˜500 years after Hippocrates. He devoted an entire chapter to detailed descriptions of asthma, suggesting that the cause of asthma was “a coldness and humidity of pneuma but the result was a thick viscid humor.” Today, discussion does not include pneuma or viscid humor; rather, asthma is defined as “a chronic inflammatory disorder causing recurrent episodes of wheezing, breathlessness, chest tightness, (and) coughing associated with airflow obstruction that is often reversible”
(2). Status asthmaticus, also known as acute severe asthma, is a condition of progressively worsening bronchospasm and respiratory dysfunction due to asthma, which is unresponsive to standard conventional therapy and may progress to respiratory failure and the need for mechanical ventilation (3). For this review, status asthmaticus is defined as acute severe
asthma that fails to respond to inhaled β-agonists, oral or IV steroids, and O2, and that requires admission to the hospital for treatment.

asthma that fails to respond to inhaled β-agonists, oral or IV steroids, and O2, and that requires admission to the hospital for treatment.
EPIDEMIOLOGY
Worldwide, an estimated 300 million people suffer from asthma (4). In children, the prevalence of asthma varies significantly by country, with the highest rates in the United Kingdom, Australia, and New Zealand, and the lowest in Eastern Europe, China, and Indonesia. In the United States, asthma affects 9.1% (6.5 million) of children <18 years of
age and is the leading cause of chronic illness in children (1). The economic burden of asthma is substantial, with ˜10 million missed school days and $726 million (US dollars) lost because of missed work. Asthma accounts for 470,000 (adults and children) annual hospital admissions across the United States and is the leading cause of hospital admission for children <18 years of age (5). It is estimated that 13%-55% of all dollars spent on asthma care are hospital related, and status asthmaticus remains a leading cause of PICU admission (6).

Asthma-related death rates in American children nearly doubled between 1980 and 1995, but recently have stabilized or even decreased, though some of the apparent decrease after 1999 may reflect changes to the International Classification of Diseases between the ninth and tenth editions (7). The number of asthma deaths in all age groups in the United States is ˜5000 per year, with 150-200 of those occurring in children < 18 years of age (1). In a clinical review of ventilated asthmatics from eight different tertiary PICUs, mortality was 4% (8).
Most of the deaths were associated with cardiac arrest prior to PICU admission (8). These data support prevailing experience that most asthma-related deaths in children occur as a result of respiratory failure or cardiopulmonary arrest that occurs prior to obtaining medical care. Models that seek to predict fatal asthma focus on identifying clinical, psychosocial, and ethnic risk factors (9). Clinical risks include a past history of ICU admission, respiratory failure, or rapid, sudden, severe deterioration. Psychosocial and ethnic risk factors include poor compliance with outpatient medical treatment, failure to perceive the severity of asthma attack, inner-city residence, denial of disease severity, and nonwhite race. African American children are four to six times more likely to die of asthma than are white children. Despite the aforementioned risk factors, nearly half of fatal asthma exacerbations occur in children with mild asthma. Some suggest that these deaths occur in a subpopulation of patients with decreased sensitivity to dyspnea or another group with “sudden asphyxial asthma” (10). This latter entity is characterized by rapid, sudden, severe airway obstruction that progresses to hypoxemic respiratory arrest over a short period, usually before presentation to medical care.

MECHANISM OF DISEASE: CORE PATHOPHYSIOLOGY
Genetics
No single asthma gene has been identified. It is likely that the disease is both polygenetic and environmentally influenced. More than 20 chromosomal regions have been linked to asthma. The most consistently linked regions have been on chromosomes 2q, 5q, 6p, 12q, and 13q (11). It is known that asthma inheritance is likely related to the inheritance of atopy. Studies in twins with asthma reveal significant genetic similarities but not identical phenotypic manifestations of asthma. This variation in disease severity may occur because airway hyperresponsiveness may be genetically distinct from atopy and is further confounded by gene-gene and geneenvironmental interactions. The gene polymorphisms of the β2-adrenergic receptors at the amino acid positions 16 and 27 have been examined in depth in an effort to clarify the phenotypic variability of airway hyperresponsiveness. In a metaanalysis of pediatric asthmatic ADRB2 gene polymorphisms, the Arg16Arg was associated with favorable clinical response to β2-adrenergic agonist therapy compared with Arg16Gly or Gly16Gly. No association was found between amino acid position 27 and clinical response in the same meta-analysis (12). In another study, critically ill pediatric patients with asthma who had improved β2-adrenergic response and decreased ICU length of stay (LOS) were associated with Gly16Gly and Gln27Glu or Glu27Glu polymorphisms (13). The clinical application of specific genetic profiles for both asthma prevention and treatment remains uncertain.
Inflammation and Immunobiology

The autonomic nervous system also contributes to bronchoconstriction through parasympathetic activation of M3 receptors by acetylcholine and excitatory nonadrenergic, noncholinergic pathways mediated by tachykinins. Similar parasympathetic pathways stimulate mucous production and concomitant airway obstruction.
Pulmonary Mechanics and Gas-Exchange Abnormalities
Pulmonary mechanical dysfunction occurs as a result of pathologic changes in bronchial smooth muscle contraction, mucosal edema, and increased mucous production, which cause decreased airway diameter and increased airflow resistance. During inspiration, negative pleural pressure causes physiologic intrathoracic airway dilation; however, during
expiration, pleural pressure approaches zero, causing physiologic intrathoracic airway narrowing. In status asthmaticus, with pathologically narrowed and obstructed airways, these physiologic differences in airway diameter result in easier air entry during inspiration but airflow obstruction during expiration, causing air trapping with each breath and lung hyperinflation (Fig. 46.1). With higher end-expiratory lung volumes, coupled with bronchospasm leading to increased airway resistance and reduced expiratory flow, expiration becomes an active rather than a passive process, which results in high energy expenditure and increased work of breathing (WOB). Diaphragmatic flattening from hyperinflation causes additional mechanical disadvantages for the muscles of expiration and additional energy expenditure. Both forced expiratory volume and forced vital capacity are decreased in status asthmaticus as a result of high airway resistance. Total lung volumes are increased because of increased functional residual capacity.
expiration, pleural pressure approaches zero, causing physiologic intrathoracic airway narrowing. In status asthmaticus, with pathologically narrowed and obstructed airways, these physiologic differences in airway diameter result in easier air entry during inspiration but airflow obstruction during expiration, causing air trapping with each breath and lung hyperinflation (Fig. 46.1). With higher end-expiratory lung volumes, coupled with bronchospasm leading to increased airway resistance and reduced expiratory flow, expiration becomes an active rather than a passive process, which results in high energy expenditure and increased work of breathing (WOB). Diaphragmatic flattening from hyperinflation causes additional mechanical disadvantages for the muscles of expiration and additional energy expenditure. Both forced expiratory volume and forced vital capacity are decreased in status asthmaticus as a result of high airway resistance. Total lung volumes are increased because of increased functional residual capacity.
![]() FIGURE 46.1. Flow-time waveform showing persistence of airflow at end expiration and resultant air trapping. |

Cardiopulmonary Interactions in Asthma
Dynamic hyperinflation in severe asthma can have significant cardiopulmonary consequences. First, high lung volumes stretch the pulmonary vasculature, increasing pulmonary vascular resistance and increasing right ventricular afterload, which compromises right ventricular function. In addition, fluctuations in pleural pressures produce significant effects on the intrathoracic vessels and right atrial venous return. During the large, negative intrathoracic pressure observed during inspiration, left ventricular afterload is increased and systolic blood pressure is decreased. Exaggerated variation in systolic blood pressure associated with intrathoracic pressure variation during inspiration is termed pulsus paradoxus (16) (Fig. 46.2). Inspiratory systolic blood pressure decreases of >10-15 torr are associated with declining respiratory function in children with status asthmaticus (16).
CLINICAL PRESENTATION AND DIFFERENTIAL DIAGNOSIS
Differential Diagnosis
Children with moderate-to-severe status asthmaticus have varying degrees of respiratory distress and gas-exchange abnormalities. Wheezing is the clinical hallmark of asthma; however, it is critical to consider the broad differential of the wheezing child prior to embarking on a diagnostic and therapeutic
course to treat status asthmaticus. The differential diagnosis of wheezing is large, but the possibilities can be grouped into diagnoses that require differentiation at the time of first evaluation and those that may allow a more deliberative evaluation. Four disparate clinical entities must be distinguished when the patient is first evaluated because each of these entities results in different diagnostic and therapeutic approaches. These entities are asthma, pneumonia, foreign-body aspiration, and congestive heart failure (Fig. 46.3).

Other diagnostic considerations in the differential diagnosis of wheezing can be divided into upper and lower airway diseases. These clinical entities are more likely to present with
chronic or recurrent symptoms, and, as such, a more contemplative evaluation may be appropriate. Upper airway obstruction, while usually presenting with stridor, can also present with wheezing. Diagnostic considerations include fixed anatomic lesions, such as vocal cord paralysis, anatomic webs, and airway hemangiomas, or dynamic airway obstruction, such as laryngomalacia, tracheomalacia, or bronchomalacia. Congenital anomalies, such as complete tracheal rings and bronchial slings, may also present with wheezing. Lower airway diseases that should be considered in addition to infection and congestive heart failure include cystic fibrosis and α1 antitrypsin deficiency.
chronic or recurrent symptoms, and, as such, a more contemplative evaluation may be appropriate. Upper airway obstruction, while usually presenting with stridor, can also present with wheezing. Diagnostic considerations include fixed anatomic lesions, such as vocal cord paralysis, anatomic webs, and airway hemangiomas, or dynamic airway obstruction, such as laryngomalacia, tracheomalacia, or bronchomalacia. Congenital anomalies, such as complete tracheal rings and bronchial slings, may also present with wheezing. Lower airway diseases that should be considered in addition to infection and congestive heart failure include cystic fibrosis and α1 antitrypsin deficiency.
History
Children with status asthmaticus typically present with respiratory distress, cough, and wheezing that has progressed over 1-2 days. Allergen exposure and upper respiratory tract infection are often triggers for the onset of illness and are frequently identified by the child or family as inciting events. In contrast, foreign-body aspiration will usually present with an abrupt onset of clinical symptoms. Assessing the time course of a wheezing episode is important to help distinguish diagnostic considerations and to determine the urgency of required therapeutic interventions. The presence of fever suggests lower respiratory infection, though asthma and pneumonia can occur together. When time permits, it is important to determine the presence of high-risk factors for asthma severity and fatality, including previous severe sudden deterioration, past PICU admissions, and previous respiratory failure with the need for mechanical ventilation.
Physical Exam
The rapid assessment of a child with status asthmaticus should focus on determining the severity of airway obstruction. The “rapid 30-second cardiopulmonary assessment,” as described by the American Heart Association, focuses on quick determination of general appearance, airway patency, effectiveness of respiratory effort, and adequacy of circulation (17). Children with severe status asthmaticus often appear lethargic and diaphoretic, are unable to phonate, and have severe retractions with paradoxical thoracoabdominal breathing. Poor air movement found on chest auscultation is an ominous sign of impending respiratory or cardiopulmonary failure.
Wheezing, which reflects turbulent airflow in obstructed airways, is usually equally audible on both hemithoraces. Asymmetric wheezing may imply unilateral atelectasis, pneumothorax, or foreign body. Expiratory wheezing alone is found in mild-to-moderate illness, whereas expiratory plus inspiratory wheezing is present in moderate-to-severe status asthmaticus. The “silent chest” is an ominous sign and may indicate either pneumothorax or the complete absence of airflow due to severe airway obstruction and imminent respiratory failure. Hypoxemia, as estimated by measured pulse oximetry, is another sign of asthma severity. Several clinical asthma scores have been used to objectively assess the severity of status asthmaticus. The Becker asthma score (Table 46.1), modified by DiGiulio, determines severity by rating the acuity of four clinical characteristics—respiratory rate, wheezing, inspiratory:expiratory ratio, and accessory muscle use—and has the advantage of not requiring a PaO2 measurement, which is used in the Wood/Downes score (18). A Becker score >4 is considered moderate status asthmaticus and has been used as entry criteria for several inpatient asthma treatment trials. Children with scores ≥7 should be admitted to the ICU. While such scores are valuable in clinical studies, they are not effective in predicting progression of clinical illness. Serial measurements allow an objective determination of disease progression, though significant interobserver variability is often associated with assigning clinical scores, which limits the usefulness of scoring systems in the active clinical environment.
TABLE 46.1 BECKER PULMONARY INDEX SCORE FOR ASTHMA | |||||||||||||||||||||||||
---|---|---|---|---|---|---|---|---|---|---|---|---|---|---|---|---|---|---|---|---|---|---|---|---|---|
|
Pulmonary Function Test and Exhaled Nitric Oxide
The fraction of exhaled nitric oxide (FeNO) is a noninvasive marker of acute eosinophilic airway inflammation and has been investigated as a tool in the diagnosis and management of childhood asthma (19). The pediatric data describing the use of FeNO to predict acute asthma exacerbations or to tailor inhaled corticosteroid therapy do not yet show convincing therapeutic value. Further, FeNO monitoring during acute asthma exacerbation in adults does not predict therapeutic response. At present, FeNO
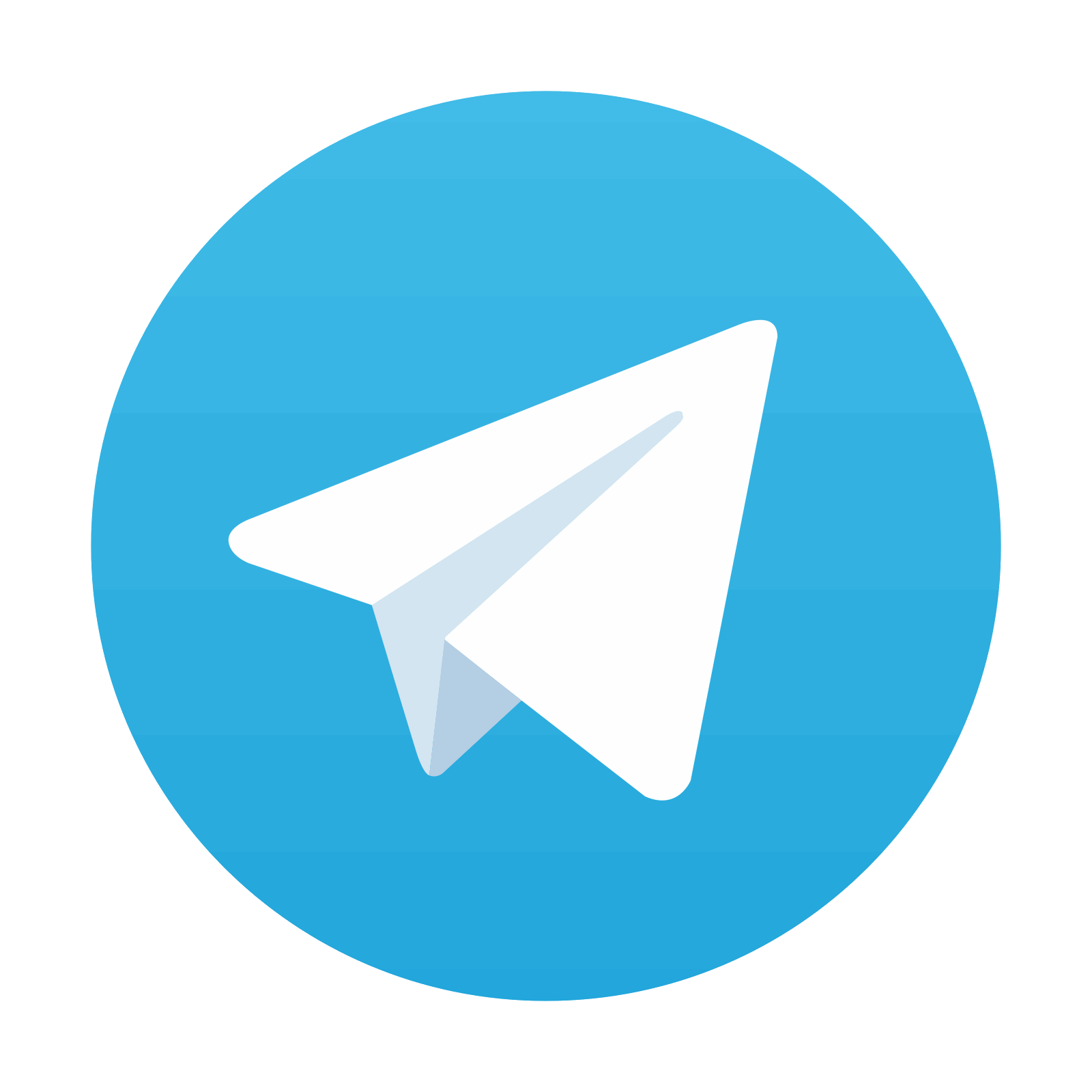
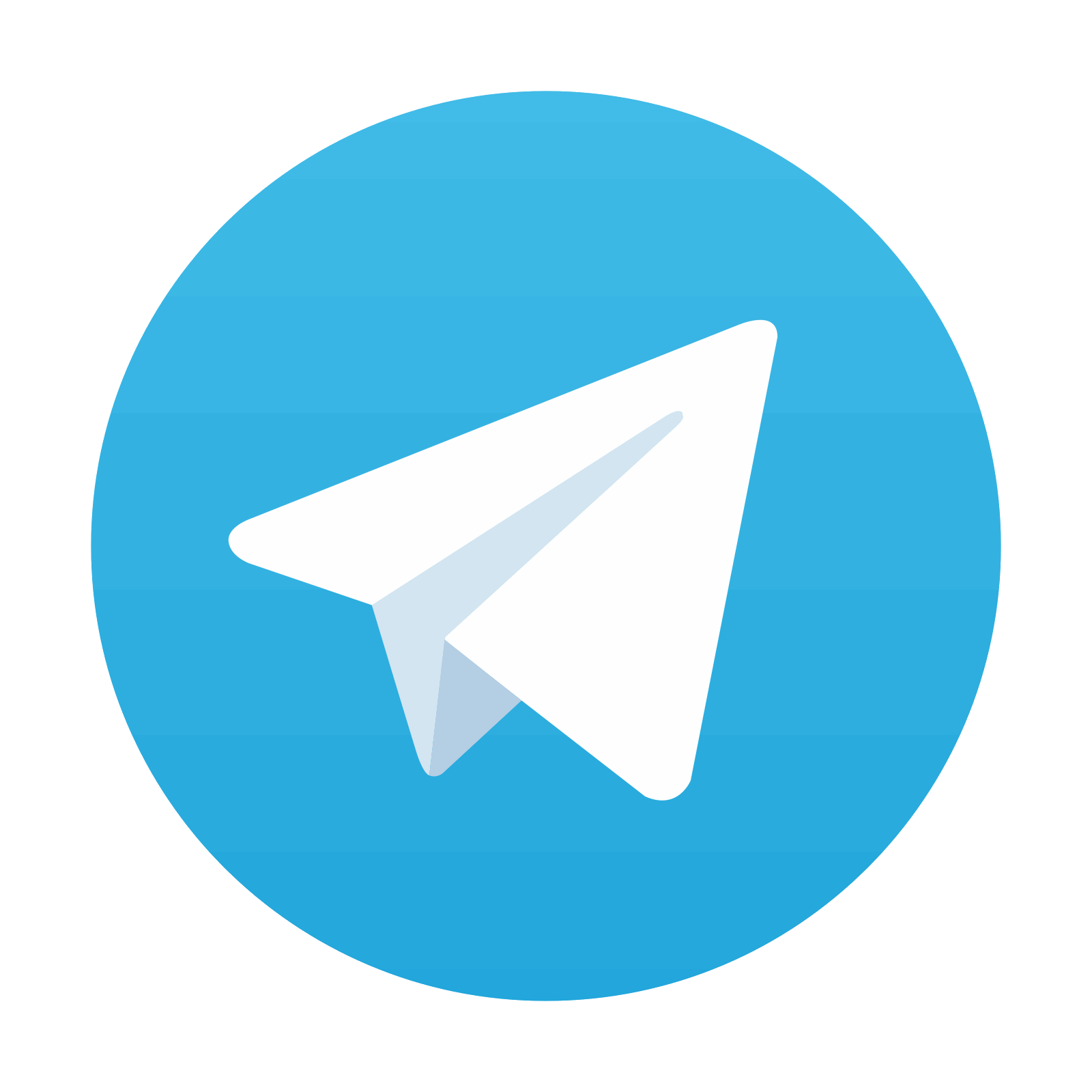
Stay updated, free articles. Join our Telegram channel

Full access? Get Clinical Tree
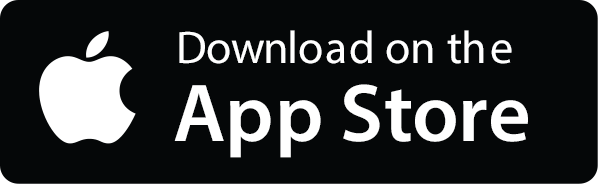
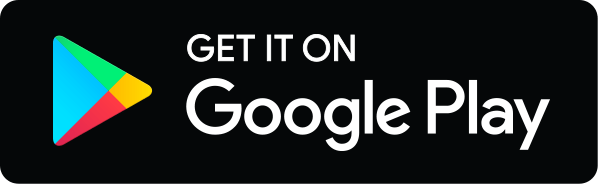
