Spinal (Subarachnoid) Blockade
Mikko Pitkänen
History
Spinal anesthesia creates an intense sensory and motor block that can effectively be achieved with a small amount of local anesthetic. In the late nineteenth century, soon after the discovery of the local anesthetic properties of cocaine, spinal anesthesia was introduced into clinical practice. In the first experiments and clinical use, the local anesthetic used was cocaine (Table 10-1) (1). The use of spinal cocaine caused a high frequency of central nervous system (CNS) side effects, muscle spasms, and pain. Therefore, the use of spinal anesthesia was limited to a few enthusiasts until safer local anesthetics—procaine (2) and later tetracaine (3)—caused a widespread interest in its use. In the ensuing years, the popularity of spinal blockade has waxed and waned. The development of modern general anesthesia using muscle relaxants and an endotracheal tube, together with the fear of neurologic complications, decreased the interest in spinal anesthesia in 1940s and 1950s (6). As operations became more radical, their duration and extensiveness were often incompatible with spinal anesthesia. Dedicated enthusiasts, however, continued to use the method and believe in its safety (7), and, about 40 years ago, spinal anesthesia started to regain its place in anesthesia care. Since the 1980s, modern disposable needles and especially the use of bupivacaine has greatly increased the interest in spinal anesthesia in many countries (8,9). The fact that general anesthesia also has its risks and problems has made spinal anesthesia a valuable option for surgery of the lower abdomen and extremities. It is used in short ambulatory procedures as a restricted saddle block or as unilateral anesthesia to provide a fast, limited, short-lasting, and reliable anesthesia with good-quality postoperative pain relief (10). Combined spinal anesthesia with epidural catheter (CSE) is widely used for anesthesia in obstetrics and vascular and orthopedic surgery (11). Continuous spinal anesthesia (CSA) using a spinal catheter can be used in hemodynamically unstable patients, for complicated operations lasting for several hours, and may be continued for postoperative pain relief (12).
Indications
All operations in the lower abdomen, perineum, and lower extremities can be performed under spinal anesthesia. The spinal anesthesia technique and dose, and the properties of the local anesthetic can be adapted to obtain optimal spread and duration of the anesthesia for the planned surgery.
Specifically, spinal anesthesia is appropriate in patients who choose to remain conscious or whose medical condition requires consciousness during the surgery, but who still need high-quality anesthesia. Patients with respiratory problems or difficult airways may not need an endotracheal tube if spinal anaesthesia is employed. The risk of vomiting and aspiration is diminished (but not eliminated) during spinal anesthesia. A full stomach is not as great a risk as with general anesthesia because the conscious patient may be able to protect the airway, but because of the possibility of untoward reactions or inadequate block needing deep sedation or conversion to general anesthesia with endotracheal intubation, the same guidelines for preoperative fasting should be used as with general anesthesia. In cesarean section, the benefits of spinal anesthesia or the CSE technique are well verified. Endoscopic urologic procedures have a risk of hypervolemia secondary to absorption of irrigating fluid causing transurethral resection (TUR) syndrome; the changes in mental status caused by TUR syndrome can be better observed during spinal anesthesia.
Cholecystectomies and gastrectomies have successfully been performed under spinal anesthesia. However, upper abdominal operations require a T4 level of sensory anesthesia. As a safety margin, the upper level should be still higher, and some unwanted reflexes may appear; thus, spinal anesthesia as a sole anesthetic technique for these operations. It can and has been used as an adjunct that enables lighter general anesthesia. In cardiac surgery, the use of intrathecal narcotics enables faster recovery (13). High spinal anesthesia (37.5 mg of bupivacaine) with general anesthesia has caused less β-receptor dysfunction and a lower stress response during coronary artery bypass graft surgery (14). For renal transplant surgery, combined spinal–epidural anesthesia has been as safe and as well tolerated as general anesthesia (15). An extreme example of the use of spinal anesthesia is a patient with severe lung disease who successfully underwent a laparoscopic cholecystectomy under segmental subarachnoid (spinal) anaesthesia performed at the low thoracic level (16).
In lower extremity surgery, peripheral blocks are used with increasing frequency for surgical anesthesia. Spinal anesthesia has the advantage of being simpler and more rapidly induced, the tolerance of a tourniquet is better, and it is also suitable for bilateral procedures. The number and complexity of nerve blocks needed for good anesthesia for operations on the knees, thigh, and hip are so great that spinal anesthesia is often the preferred technique.
Contraindications
Contraindications for spinal anesthesia are classified usually as absolute or relative. Absolute contraindications are:
Patient’s refusal despite adequate information. There are several indications for which spinal anesthesia would be a better choice than general anesthesia, but rarely is general anesthesia absolutely contraindicated and, in those cases, the patient usually understands the need for regional anesthesia and accepts the choice.
Infections at the site of injection. No needle should pass through an infected area before entering into the subarachnoid space.
Dermatologic conditions (e.g., psoriasis) that preclude aseptic preparation of the skin at the site of injection.
Septicemia or bacteremia. In these circumstances, the infection may spread to the CNS. Central neuronal block is not recommended in patients with untreated systemic infection; however, if appropriate antibiotic therapy has been initiated, patients who have shown a response to therapy may safely undergo single-dose spinal anesthesia (17).
Shock or severe hypovolemia. Since spinal anesthesia causes sympathetic block and vasodilatation, the hypotension in a hypovolemic patient may cause severe problems.
Abnormality in blood clotting mechanism (see Chapter 12).
Increased intracranial pressure. Because of lumbar puncture, the pressure of cerebrospinal fluid (CSF) in the spine may be lowered; therefore, the high intracranial pressure may cause herniation of the medullary vasomotor and respiratory centers.
Lack of skill in spinal anesthesia.
Allergy to local anesthetics. This is extremely rare, and usually another choice of local anesthetic is available.
Relative contraindications include:
Deformities of the spinal column (spinal stenosis, severe arthritis, severe kyphoscoliosis, ankylosing spondylitis). These may cause difficulties in finding the subarachnoid space. Multiple punctures increase the risk of bleeding in the epidural space and, indeed, spinal stenosis was found to be a risk factor for spinal hematoma (18).
Preexisting disease of the spinal cord. The hypothesis is that abnormal nervous tissue is more susceptible to the neurotoxicity of local anesthetics than normal nervous tissue. There are no objective data, but common sense and medicolegal considerations make it prudent to avoid spinal anesthesia in patients with progressive disease involving the cord. Otherwise, the progression of the disease may be blamed on the spinal anesthetic. However, if the neurologic status is stable and there is a common agreement with the patient about the risks and benefits of regional anesthesia, no contraindication exists to proceeding with spinal anesthesia. There are several case reports on the safe use of spinal anesthesia in patients with postpolio syndrome or multiple sclerosis (19,20).
Chronic headache or backache. These can sometimes be aggravated by spinal anesthesia; however, if sufficient information is provided and agreement about the procedure is obtained from the patient, spinal anesthesia can be safely used.
Inability to achieve a spinal tap in three attempts. One should either abandon spinal anesthesia or obtain the help of a more experienced anesthesiologist.
Certain cardiac diseases. Marked aortic stenosis is considered a contraindication to spinal anesthesia. The sympathetic block and vasodilatation causes a sudden decrease in systemic vascular resistance that may lead to a profound decrease in coronary perfusion. However, if only a limited block or continuous techniques with the possibility to titrate the dose carefully are used, spinal anesthesia can safely be used even in patients with aortic stenosis (21).
Tattoo on the back. The recent trend of having tattoos in the lumbar area can cause a concern if the needle has to pass through pigmented tissue. It is possible that the needle may transfer pieces of the pigment into the subarachnoid space. This could theoretically cause inflammation or a granulomatous response. Naturally, the tattoo should not be punctured, and usually there is pigment-free skin in another interspace or paramedially where the needle can be passed. Use of an introducer needle does not abolish this risk. If the pigmented skin must be punctured, a small superficial skin incision before introducing the needle has been suggested (22). A good practice may also be to allow some drops of CSF to drip from the needle before injecting the anesthetic.
Table 10-1 Milestones of spinal anesthesia | ||||||||||||||||
---|---|---|---|---|---|---|---|---|---|---|---|---|---|---|---|---|
|
Pharmacology
Local Anesthetics
Almost all local anesthetics have also been used at some point in spinal anesthesia. This chapter concentrates on their special properties in the subarachnoid space and on possible factors that influence the spread and duration of spinal anesthesia (Table 10-2).
The spinal nerves in the subarachnoid are without the protection of dura mater and arachnoid membranes, since only a thin layer of pia mater covers them. Thus they are more vulnerable to damage than are the peripheral nerves, which have extensions of dura and arachnoid with layers of connective tissues, fat cells, and blood vessels to protect them. The lack of this protection makes fast and intense block with a small amount of local anesthetic possible. On the other hand, it is also possible to produce irreversible injury by administering high concentrations of local anesthetic in a small area. This was observed when relative overdoses of hyperbaric lidocaine, 50 mg/mL, were used for continuous spinal anesthesia through microcatheters. The tip of the catheter was probably located caudally, and the local anesthetic accumulated in a small area and caused permanent neurologic damage (23). This happened even though lidocaine had been safely used for spinal anesthesia for almost 50 years.
Procaine
Procaine is a fast and short-acting local anesthetic. It was the first relatively safe and widely used spinal anesthetic. When the appearance of transient neurologic symptoms was associated
with lidocaine, procaine was again studied to see if it could replace lidocaine as a spinal anesthetic for ambulatory surgery. The transient neurologic symptoms (TNS) frequency with procaine is small, but unfortunately it causes a high frequency of nausea and the quality of the block is not adequate (24,25). The frequency of pruritus when it is used with fentanyl is high (26).
with lidocaine, procaine was again studied to see if it could replace lidocaine as a spinal anesthetic for ambulatory surgery. The transient neurologic symptoms (TNS) frequency with procaine is small, but unfortunately it causes a high frequency of nausea and the quality of the block is not adequate (24,25). The frequency of pruritus when it is used with fentanyl is high (26).
Table 10-2 Drugs used for spinal anesthesia and approximate durations of blocks | ||||||||||||||||||||||||||||||||||||||||||||||||||||||||||||||||||||||
---|---|---|---|---|---|---|---|---|---|---|---|---|---|---|---|---|---|---|---|---|---|---|---|---|---|---|---|---|---|---|---|---|---|---|---|---|---|---|---|---|---|---|---|---|---|---|---|---|---|---|---|---|---|---|---|---|---|---|---|---|---|---|---|---|---|---|---|---|---|---|
|
Lidocaine
Lidocaine, 50 mg/mL, as a hyperbaric solution was widely used for spinal anesthesia since the 1950s. In the early 1990s, however, came reports of cauda equina syndrome when overdoses of anesthetic mixtures containing lidocaine were used for continuous spinal anesthesia through microcatheters (23). Soon thereafter, reports of transient radicular irritation (TRI), later called TNS, were published (27). In a prospective survey from France, 75% of the neurologic deficits after nontraumatic spinal anesthesia occurred in patients who had received hyperbaric lidocaine, 50 mg/mL, at a frequency of 14.4 per 10,000 (28). Plain lidocaine with a reduced concentration (20 mg/mL) and dose is still used. However, the incidence of TNS does not decrease even though the concentration of lidocaine is decreased to 5 mg/mL (29,30).
Tetracaine
Tetracaine was a popular and widely used spinal anesthetic but the amide local anesthetics have replaced it in many places. Tetracaine is long-acting, and epinephrine further increases its duration of action. It is perhaps not as reliable as bupivacaine (31). It does not prevent tourniquet pain as well as bupivacaine, and it seems to cause more hypotension than bupivacaine (32,33). The mechanism for these differences is unknown.
Bupivacaine
Bupivacaine is probably the most widely used spinal anesthetic (9). It is used as a 2.5 to 7.5 mg/mL concentration with and without glucose. Plain solution is often called isobaric but at body temperature it is hypobaric. Therefore the term plain bupivacaine instead of isobaric bupivacaine should be used for additive-free bupivacaine solutions. The duration and extent of anesthesia depend on the dose and baricity. Using a 4 mg hyperbaric solution, an ambulatory arthroscopy of the knee with duration less than 1 hour and with rapid home discharge can be performed (34). On the other hand, using 20 mg plain solution provides anesthesia for bilateral knee arthroplasty with duration of 3 to 4 hours. The spread of plain bupivacaine solution is unpredictable (35). The later discussion of factors affecting the spread of spinal anesthesia is based mostly on studies with plain bupivacaine.
Levobupivacaine
Levobupivacaine is less cardiotoxic than bupivacaine. However, the doses used for spinal anesthesia (maximum of 25 mg) do not cause cardiotoxicity even if accidentally injected intravenously, thus this issue is not a concern in spinal anesthesia. In several experiments in which the spinal anesthetic properties of levobupivacaine and bupivacaine have been compared, they appear identical (36,37). Therefore, there should be no benefit in using levobupivacaine for spinal anesthesia. On the other hand, if bupivacaine is not available, it can be replaced by levobupivacaine (38).
Ropivacaine
Ropivacaine, another levo- isomer of the amide local anesthetics, is less potent and of shorter duration than bupivacaine. In a volunteer study, the potency of ropivacaine for spinal anesthesia appeared to be only half that of bupivacaine (39). When relatively large doses (17.5 mg) of spinal bupivacaine or ropivacaine were compared in patients scheduled for hip arthroplasty, little difference was noted in the duration of the sensory block (median duration at T10, 3 versus 3.5 hours) but the median duration of motor block (Bromage score 3) was shorter after ropivacaine than bupivacaine (2.1 versus 3.9 hours) (40). When ropivacaine doses of 15 and 20 mg were compared to a bupivacaine dose of 10 mg, the conclusion was that the duration of sensory block from ropivacaine was two-thirds and the duration of motor block one-half when compared with bupivacaine, with calculations based on the duration-per-milligram of the local anesthetic (41). When smaller doses were compared, the effective dose of ropivacaine was 1.5 times higher than that for levobupivacaine or bupivacaine (42,43). In herniorrhaphy, 8 mg of hyperbaric levobupivacaine and 12 mg of hyperbaric ropivacaine provided similar unilateral anesthesia (42). Likewise, for arthroscopy of the knee, 5 mg of hyperbaric levobupivacaine and 7.5 mg of hyperbaric ropivacaine provided
adequate blockade. The home discharge criteria were fulfilled faster with ropivacaine (43). In two studies in which hyperbaric and plain spinal ropivacaine (15 mg) were compared, the hyperbaric solution produced a faster onset and recovery as well as better reliability (44,45). For ambulatory surgery, hyperbaric ropivacaine may be a good option, allowing fast recovery of motor function. Unfortunately, hyperbaric solutions are not yet available, and they must be mixed at the bedside, which may pose the risk of possible miscalculations or wrong ingredients being used.
adequate blockade. The home discharge criteria were fulfilled faster with ropivacaine (43). In two studies in which hyperbaric and plain spinal ropivacaine (15 mg) were compared, the hyperbaric solution produced a faster onset and recovery as well as better reliability (44,45). For ambulatory surgery, hyperbaric ropivacaine may be a good option, allowing fast recovery of motor function. Unfortunately, hyperbaric solutions are not yet available, and they must be mixed at the bedside, which may pose the risk of possible miscalculations or wrong ingredients being used.
Table 10-3 Additives to spinal anesthetics and usual doses | |||||||||
---|---|---|---|---|---|---|---|---|---|
|
2-Chloroprocaine
Recently 2-chloroprocaine has gained interest in spinal anesthesia for ambulatory surgery. The neurotoxicity of 2-chloroprocaine or its additives has been the center of a controversy for several years. When preservative-containing preparations were used for epidural anesthesia, permanent neurologic injury after unintentional subarachnoid injection was reported. The additive sodium bisulfite and low pH were claimed to be the reason for neurotoxicity (46,47).
Preservative-free preparations of 2-chloroprocaine have been tested for spinal anesthesia; 40 mg of 2-chloroprocaine, 10 or 20 mg/mL, produces a reliable block for 60 minutes, with a fast onset and rapid recovery (48,49). No TNS has been reported in the preliminary studies. The safety of this preparation needs to be confirmed, since in a neurotoxicity study in rats 2-chloroprocaine caused significant functional impairment and histologic damage and, surprisingly, the additive bisulfite seemed to actually protect the rats from histologic damage (50). On the other hand, in a similar earlier experimental study from the same group, comparable changes in histology were observed with lidocaine and prilocaine (51). In these studies, the 2-chloroprocaine concentration was 30 mg/mL and that for lidocaine and prilocaine 25 mg/mL. Even though no neurologic damage has been observed in these clinical studies, more studies are needed before 2-chloroprocaine can be recommended for widespread use.
Articaine
The need for safe, short-acting agents has caused renewed interest in another old spinal anesthetic, articaine. It has been found comparable to lidocaine, and it has been used in some parts of Europe (52). When it was used for ambulatory surgery, an 84-mg dose of hyperbaric articaine provided anesthesia for 70 minutes and no TNS was observed (53).
Prilocaine
In some countries, prilocaine is used for short-lasting spinal anesthesia. Its properties are similar to those of lidocaine, although it may have a slightly longer duration of action. Transient neurologic symptom frequency is less than with lidocaine (54).
Additives
Different additives (Table 10-3) have been used with local anesthetics for spinal anesthesia, mostly in order to prolong or intensify the block. For example, the duration of tetracaine spinal anesthesia can be prolonged by 25% to 50% with the addition of epinephrine 0.2 to 0.5 mg (31,55). Additives have also been used with the aim of intensifying anesthesia without prolonging recovery. One indication is also the prevention of postoperative pain.
The safety of drugs injected into the subarachnoid space must be affirmed by animal research before they are used clinically (56).
Opioids
The local anesthetics and opioids have synergistic analgesic properties in the intrathecal space (57). This synergism does not affect the motor block. The major effect of intrathecally administered opioids is achieved through binding to μ-opioid receptors (58). Opioids selectively modulate nociceptive afferent input from A- and C-fibers; in addition, other receptors (κ) have analgesic effects (59).
Morphine
Morphine is a hydrophilic opioid with a long duration of action in the intrathecal space because of its low spinal cord distribution volume and slow clearance into plasma (60).
It is used as a 0.1- to 0.5-mg dose in combination with local anesthetic, and it provides good postoperative pain relief for up to 24 hours. Because of its hydrophilicity, morphine spreads in the intrathecal space; therefore, even after lumbar injection it can relieve pain after thoracic surgery. However, there is a delay in onset of analgesia of several hours. It is also carried rostrally to the level of the brainstem, and delayed respiratory depression can occur. Fortunately, this complication is rare but can occur even 24 hours after the injection.
The needed dose depends on the surgery. For example, for cesarean section, 0.1 mg provides good analgesia; 0.2 mg is adequate for hip arthroplasty, but even a dose of 0.3 mg did not relieve the pain after knee arthroplasty (61).
With increasing dose, the risk of side effects, nausea, vomiting, pruritus, and respiratory depression increases.
Morphine has also been used combined with bupivacaine for continuous intrathecal analgesia after orthopedic surgery (62). Intrathecal morphine cannot be recommended for ambulatory surgery. With a small dose (0.05 mg), the pain relief was enhanced but nausea, pruritus, and delay in voiding prevent the use of intrathecal morphine in ambulatory surgery (63).
Lipophilic Opioids
Fentanyl and sufentanil are lipophilic opioids which, after intrathecal injection, rapidly penetrate to the spinal cord and also
through the dura to the epidural fat. Late respiratory depression is not a risk, but fast rostral spread may cause respiratory depression immediately (20–30 minutes) after the injection. Because of the high lipophilicity, the main effect of these opioids is on those dermatomes close to the injection (64,65).
through the dura to the epidural fat. Late respiratory depression is not a risk, but fast rostral spread may cause respiratory depression immediately (20–30 minutes) after the injection. Because of the high lipophilicity, the main effect of these opioids is on those dermatomes close to the injection (64,65).
Fentanyl
Fentanyl is typically used for ambulatory surgery. Small doses (10–25 μg) intensify the block without markedly prolonging it. With the use of fentanyl, the dose of local anesthetic can be diminished; the recovery of sensory and motor block is fast, without risking an excessive level of analgesia during surgery. When 4 mg intrathecal bupivacaine was compared to 3 mg intrathecal bupivacaine plus 10 μg fentanyl for arthroscopic surgery of the knee (66), the level of analgesia was equal but recovery of motor block was faster and time in the post anesthesia care unit shorter in the fentanyl group. In another study, the addition of 10 μg fentanyl to 5 mg of hyperbaric bupivacaine increased the success rate of spinal anesthesia from 75% to 100% (67). In ambulatory surgery, intrathecal fentanyl causes a high frequency of pruritus, which sometimes can be disturbing (68).
Side Effects of Intrathecally Administered Opioids
Respiratory Depression
Respiratory depression is the most feared complication of the use of intrathecal opioids. As already discussed, the risk differs depending on the properties of the opioid (hydro- versus lipophilic).
Pruritus
The pathophysiology and possibilities for treatment of this sometimes troublesome side effect are summarized in an extensive review by Szarvas and co-workers (68). This side effect is most commonly observed in obstetric and ambulatory patients. The incidence has been reported to be between 30% and 100%, and it seems to be equally common after fentanyl, sufentanil, and morphine. Different treatments and prevention modes have been tried, but it seems that naloxone is the best treatment. 5-HT3 receptor antagonists have been tested with variable success (69).
Antihistamines provide relief from the itching but do not prevent pruritus (70).
Nausea
Regardless of the path of administration—perorally, intravenously, or intrathecally—opioids may cause nausea and vomiting. Nausea is common after intrathecal morphine (35%–75%), and it seems to be dose dependent. In a volunteer study, intrathecal morphine caused a dose-related increase in emesis (71). Similarly, in clinical studies, the frequency of nausea increased with the dose of morphine used (72). If small doses of intrathecal morphine (<100 μg) have been used, the frequency of nausea is smaller. When small doses of sufentanil or fentanyl have been used in ambulatory surgery, nausea and vomiting is not a significant problem. Similar results have been reported when these lipophilic opioids have been used for labor pain or during cesarean delivery.
Vasoconstrictors
The mechanism of the vasoconstrictors in prolonging the effect of local anesthetics is reduction of the blood flow, which decreases the absorption of local anesthetics into the systemic circulation. Thus, the local anesthetics remain longer in the spinal space and their action is prolonged. There has been concern that the vasoconstrictors produce ischemia of the spinal cord and may lead to neurologic complications. This has been observed in animal experiments (73). On the other hand, in several controlled clinical studies in which spinal anesthesia with or without vasoconstrictives have been compared, no neurologic complications were attributed to the use of vasoconstrictors.
The recommended dose for epinephrine is 0.2 to 0.3 mg, and for phenylephrine 2 to 5 mg (74). They both prolong the duration of tetracaine spinal anesthesia and, in rigidly controlled studies, even a dose-response effect can be seen (75). When equipotent doses of epinephrine and phenylephrine are used, the prolongation is equal. When epinephrine has been used with lidocaine or bupivacaine, the duration of the block has not markedly changed (76,77). The reason for this difference is likely the different intrinsic vasodilatation/constriction properties of the local anesthetics. On the other hand, with hyperbaric lidocaine, epinephrine prolonged surgical anesthesia but also, significantly, prolonged the time required to regain the ability to void (78). When epinephrine was added to 2-chloroprocaine, it increased the duration of the block, but the use of epinephrine and 2-chloroprocaine caused vague, nonspecific flu-like symptoms and therefore is not recommended (79).
The use of vasoconstrictors is not advisable in ambulatory surgery. Vasoconstrictors may be used to prolong tetracaine spinal anesthesia in major operations, but they do not prolong the effect of bupivacaine (80).
α2-Adrenergic Agonists
Clonidine was initially introduced as an antihypertensive drug. Now its main indication is in pain therapy. Its action is mediated by α2-adrenergic receptors. These receptors are found in the substantia gelatinosa (81) of the dorsal horn of the spinal cord and also in primary afferent terminals and within several brainstem nuclei implicated in analgesia (82), thus explaining clonidine’s analgesic effect after systemic, intrathecal, and local administration. In spinal anesthesia, clonidine intensifies and prolongs both sensory and motor block. Clonidine also potentiates the effects of intrathecal opioids in a synergistic way. Because clonidine is an antihypertensive drug, hypotension, bradycardia, and sedation are common side effects.
Even a small dose of clonidine (15 μg) prolongs markedly the sensory block if a large dose of local anesthetic is used (bupivacaine 17.5 mg) (83). The same dose has also improved analgesia for inguinal herniorrhaphy (84). Doses of up to 300 μg have been used intrathecally; however, these doses have caused marked hemodynamic changes and sedation (85). Interestingly, oral doses of 150 to 300 μg as premedication also have intensified spinal anesthesia, although, unfortunately hypotension is more common than after intrathecal use (86,87). If 3 μg/kg of clonidine is given intravenously after the spinal injection of bupivacaine (10 to 50 minutes), it may increase the duration of spinal anesthesia by 60 to 80 minutes (88).
Dexmedetomidine, another α2-adrenergic receptor agonist, has also been studied in spinal anesthesia with bupivacaine. Dexmedetomidine at a dose of 3 μg had a similar effect in prolonging the block as 30 μg of clonidine when injected with 12 mg of bupivacaine (89).
Mechanism of Spinal Anesthesia
Uptake
The nerves of the subarachnoid space do not have the protection of dura or arachnoid. Therefore even a small amount of local anesthetic in the CSF will cause a profound block of nerve transmission. Local anesthetic for spinal anesthesia is usually injected into the subarachnoid space between the spinous processes of the third and fourth lumbar vertebra. The needle will enter the dura in the area of the cauda equina, where the nerve roots cross the subarachnoid space from the spinal cord to their points of exit through the dura. The surface area of the nerve roots is considerable, thus making them vulnerable to the effects of local anesthetic (90). The nerve roots differ markedly in their size. The smallest roots have the relatively largest surface area, and local anesthetic penetrates them rapidly. Large individual variability exists among the properties of spinal anesthesia (Table 10-4). One reason for this may be the size of nerve roots, which differ greatly among individuals (e.g., the area for posterior L5, the most variable root, ranges from 2.2 mm2 to 7.4 mm2) (91).
The spinal cord itself also takes up local anesthetic, mostly by diffusion through the pia mater. This is a slow process and affects only the superficial portions of the cord. Local anesthetics can also penetrate to the deeper structures of the cord through the extensions of the subarachnoid space, which exist around those blood vessels that make their way through the pia mater. Because of the easy accessibility of local anesthetic to the nerve roots, it would be logical to assume that concentrations of local anesthetics are higher in nerve roots than in the cord. However, in experiments of the neuraxis of the dog, it seems that the opposite is true (90), because local anesthetics are lipid soluble, and highly myelinated fibers in the spinal cord will take up high concentrations.
Differential Sensitivity of Nerve Fibers
The major anesthetizing effect is caused by the local anesthetic in spinal nerve roots and dorsal root ganglia. The spread of local anesthetic in the spinal space is determined by diffusion, movement of CSF and, most importantly, baricity (relationship of the density of the local anesthetic and CSF). When the distance from the injection site increases, the amount of local anesthetic decreases and fewer molecules are available to produce a block of nerve transmission. This, together with the fact that the nerve fibers differ in their sensitivity to the blocking agents, provides the explanation for the zones of differential blockade seen in spinal anesthesia. Traditionally, the extent of the spinal block has been differentiated between sympathetic block, sensory block, and motor block. Several reports note that the measured loss of cold-temperature discrimination (sensitive C-fibers and comparatively sympathetic fibers) occurs two to four spinal segments above the level of pin-prick sensory blockade (Aδ-fibers), which again is one or two segments higher than the level of anesthesia to light touch (Aβ-fibers) (92,93). The correlation of different modalities of sensation and fiber size appears accurate. Sensation of touch correlates with Aβ-fiber function, sensation of pin-prick with Aδ-fibers, and sensation of cold with C-fiber function. This correlation of fiber function and sensation during recovery was reported by Liu and co-workers after lidocaine spinal anesthesia in volunteers (94). They determined cutaneous current-perception thresholds at different frequencies and found a good correlation between recovery of the different nerve fibers and touch, pin-prick, and cold, respectively. Return of Aβ current-perception threshold (touch) correlated with duration of surgical anesthesia as assessed using an electrical stimulation model.
Table 10-4 Uptake and elimination of local anesthetics from cerebrospinal fluid | |
---|---|
|
The block and recovery of sensory fibers occurs in this order: The most sensitive sensory fibers—C-fibers (sensation to cold)—are blocked first and remain blocked longest; Aδ-fibers (pin-prick) are the second to be blocked and recover; and Aβ-fibers (touch) are the last to block and first to recover (Fig. 10-1).
The preganglionic sympathetic fibers (B-fibers) are most sensitive to the local anesthetics. Traditionally, the spread of
sympathetic block during spinal anesthesia was supposed to extend as high as the block of cold sensation (C-fibers). However, the sympathetic block during spinal anesthesia is more complex, because sympathetic fibers are not necessarily blocked when the sense to cold is blocked. In studies in which sympathetic function during spinal anesthesia has been measured by thermography, laser Doppler flowmetry, or skin conductance responses (95,96,97), an inconsistency is noted in the extent of the sympathetic block compared to the block of pin-prick or touch. In another, more recent study, a high-level spinal anesthesia with hyperbaric lidocaine, bupivacaine, or tetracaine attenuated sympathetic function but did not produce complete sympathectomy when tested by cold pressor test (98).
sympathetic block during spinal anesthesia was supposed to extend as high as the block of cold sensation (C-fibers). However, the sympathetic block during spinal anesthesia is more complex, because sympathetic fibers are not necessarily blocked when the sense to cold is blocked. In studies in which sympathetic function during spinal anesthesia has been measured by thermography, laser Doppler flowmetry, or skin conductance responses (95,96,97), an inconsistency is noted in the extent of the sympathetic block compared to the block of pin-prick or touch. In another, more recent study, a high-level spinal anesthesia with hyperbaric lidocaine, bupivacaine, or tetracaine attenuated sympathetic function but did not produce complete sympathectomy when tested by cold pressor test (98).
The motor fibers (Aα, the largest fibers) are less sensitive to local anesthetics than are sensory fibers. The level of motor block is supposed to be two to four dermatomes lower than the level of sensory anesthesia.
This difference between sensory and motor block seems inconsistent. Motor function is better preserved because more local anesthetic is needed to anesthetize the thick motor fibers. This is well observed in ambulatory surgery, when very small doses of local anesthetics are used. Despite adequate sensory block, the amount of local anesthetic is too small to cause significant motor blockade (99).
These zones of differing reaction to spinal anesthesia remain constant also during the emergence from spinal anesthesia.
Elimination
As local anesthetic molecules are absorbed by neuronal tissues, the concentration in the CSF decreases. This is a time-consuming procedure, since free local anesthetic can be detected in the CSF over 60 minutes after the injection. (The level of anesthesia changes after the repositioning of the patient when plain or hyperbaric bupivacaine is used [100,101].) However, even a small amount of free local anesthetic in the CSF can cause block—an important consideration since increase in the level of anesthesia can also increase the risk of hypotension.
The duration of spinal anesthesia is dependent on the rate of elimination of the local anesthetic. Elimination occurs entirely by vascular absorption (102,103). No intrathecal metabolism occurs. The duration of the block and rate of elimination depend on the extent of spinal anesthesia. Because of large individual variability, a dose of 15 mg of bupivacaine can cause a block to a level of T2 or L2. If the block is at the T2 level, a much larger area of absorption is present and therefore faster elimination and shorter duration of the block occurs in the upper dermatomes. The vascular absorption happens within the subarachnoid space, principally through vessels in the pia mater. The free local anesthetic in the CSF passes also through the dura outward to the epidural space, where the rich vasculature rapidly eliminates the local anesthetic to the systemic circulation (104). A difference exists in the absorption kinetics of lidocaine and bupivacaine. Lidocaine is absorbed through a single first-order absorption process (half-life, 71 minutes), whereas the more lipophilic bupivacaine binds to the epidural fat and its absorption can be characterized by two parallel first-order absorption processes (half-lives of 50 and 408 minutes). The first absorption equals that of lidocaine; the second, slower one is caused by the slow absorption from epidural fat (105). The plasma concentrations after spinal anesthesia are small: When 15 mg of plain or hyperbaric bupivacaine (5 mg/mL) was used, mean peak plasma concentrations of 63 ng/mL were observed (103). Interestingly, the times to peak concentration were shorter when hyperbaric bupivacaine was used (103,106). This reflects the shorter duration of the block with hyperbaric solutions, in which the baricity or glucose concentration interferes with the absorption.
Increasing age affects the pharmacokinetics of intrathecally injected local anesthetics. The total plasma clearance of intrathecally administered bupivacaine is decreased and the elimination half-life increased with increasing age (107,108). The most probable reason for this is a decrease in hepatic enzyme activity. With local anesthetic doses used for spinal anesthesia, the plasma concentrations will not increase to systemic toxic levels.
Factors Affecting Intrathecal Spread
The spread of spinal anesthesia, especially with plain local anesthetic solutions (local anesthetic solutions without additives), is unpredictable (35). Fortunately, several things can help the anesthesiologist to decide the required technique, dose, and characteristics of the local anesthetic solution to anesthetize the area for a planned surgery for sufficient duration. Unnecessarily high or low blocks can be avoided by choosing the appropriate technique.
Greene, in his classical review from 1985, listed several factors that may influence the distribution of local anesthetic solution in the subarachnoid space (109). Some of these factors are controllable and others (e.g., patient characteristics) cannot be controlled. Thus, when we know the characteristics of the patient, we can modify our technique in order to get a good and safe spinal anesthesia. Some important factors are presented in Table 10-5.
Patient Characteristics
Age
It seems that, with advancing age, the nerves become more vulnerable to local anesthetics. The number of myelinated nerves decreases (110) and conduction velocity, especially in motor nerves, slows (111). There is sensory neuron loss, distal axonal degeneration, axonal atrophy, and an accumulation of multiple mitochondrial DNA mutations in muscle, and physical inactivity and deconditioning occur (112). With increasing age, the volume of CSF decreases, and its specific gravity increases (113). The results of different studies are not uniform but it seems that there is a tendency for spinal anesthesia, administered using the same technique and dose, to cause a faster onset, higher level of blockade, and longer lasting anesthesia with increasing age (108,114,115).
Height
In normal-sized adults, height does not influence the spread of anesthetics, but when extremes are compared, height plays a role. For example, when an identical dose of local anesthetic is injected into the spinal space of an individual 210 cm tall, the spread cannot be expected to be the same as in an individual 130 cm tall. Theoretically, the length of vertebral column would be even more important than height, but, within the limits of normal-sized adults, this parameter does not seem to affect the spread of anesthesia (116).
Weight
No linear correlation exists between obesity and spread of spinal anesthesia. If body mass index (BMI; an index of relative weight [kg/m2]) instead of weight is used, some effect on anesthetic spread can be observed. When the spread of spinal anesthesia using 15 mg plain bupivacaine in thin (BMI <21 kg/m2) versus obese (BMI >30 kg/m2) patients was compared, the spread was higher in obese patients (mean T5 versus T10). However, a large individual variability was also observed (T9–C7 versus L2–T4) (117).
Table 10-5 Factors affecting distribution of local anesthetic in cerebrospinal fluid | |||||||||||||||||||||
---|---|---|---|---|---|---|---|---|---|---|---|---|---|---|---|---|---|---|---|---|---|
|
It is possible that the abdominal mass in obese patients decreases the CSF volume and thus leads to a larger spread of spinal anesthesia (see the section on Cerebrospinal Fluid Properties) (118,119).
The influence of these patient characteristics (age, weight, height) is not uniform. Some correlation can be observed, as shown in a study by Pargger and co-workers (116), who performed spinal anesthesia using 18 mg plain bupivacaine in 100 patients over 49 years of age. In a multiple regression analysis age, weight, and height were found to significantly correlate with sensory level. However, the predictive value was low.
Cerebrospinal Fluid Properties
According to the traditional literature, the volume of CSF in the spinal subarachnoid space varies between 25 and 35 mL. Modern technology has allowed us to accurately measure the volume of CSF. In 25 healthy volunteers, the volume of CSF was measured using magnetic resonance images (MRI) (118). The mean volume from the T11–T12 disc to the sacral terminus of the dural sac was 49.9 ± 12.1 mL. Large individual variability occurs, with a range of 28.0 to 81.1 mL. Interestingly, this volume was significantly less in relatively obese subjects (42.9 ± 9.5 mL) than in nonobese subjects (53.5 ± 12.9 mL). In another MRI study, slightly smaller values of CSF volume (10–61 mL) were observed (119). A correlation was again noted with obesity; patients with spinal stenosis also had smaller CSF volumes. In a volunteer study, an inverse correlation between CSF volume measured by MRI and peak sensory height of hyperbaric lidocaine spinal anesthesia was observed (Fig. 10-2) (120). The same observations were recently repeated in a clinical study using plain bupivacaine (121). Lumbosacral CSF volume inversely correlated with peak sensory block level (p = 0.65, P <0.0001).
These studies confirm that, if the volume of CSF is small, there will be more extensive spread of the local anesthetic in the intrathecal space, and maximum spread of anesthesia will be higher.
It is speculated that the decreased CSF volume is caused by abdominal pressure, which compresses the epidural space and displaces the CSF. Abdominal compression does diminish the CSF volume (118,122). As mentioned earlier, a correlation does exist between the volume of CSF and BMI. The CSF volume is also diminished in the elderly. Thus, it seems that at least part of the reason for the larger spread of spinal anesthesia in obesity or pregnancy is the compression of the epidural space, which diminishes the CSF volume.
Density of CSF has a minor effect on the maximum spread of plain bupivacaine (121,123). When 15 mg of plain bupivacaine was used for spinal anesthesia, a correlation was noted between the maximum extent of the block and CSF density (123). Different components of CSF (glucose, proteins) affect its density, but the effect of these components on the spread of the block has not been verified (123,124).
Site of Injection
When spinal anesthesia is performed at the L4–L5 level compared to L2–L3 level, the spread after the former injection is lower, but the variation is large (125). However, the routine use of L2–L3 or higher levels for spinal anesthesia is not recommended. This difference in the spread of anesthesia is therefore theoretical. When injections to L3–L4 and L4–L5 have been compared, little difference in the properties of the block have been noted (126).
If the needle is inserted through the L2–L3 interspace into the subarachnoid space, it should not reach the conus in most adults. Unfortunately, the ability to accurately identify the lumbar interspaces is not good. In 100 patients scheduled for lumbar MRI, anesthesiologists were asked to identify and mark the lumbar interspace. The marker was one space higher than assumed in 51% of cases and the interspace was identified correctly in only 29%. Obesity made it more difficult to place the markers accurately. In 19% of patients, the spinal cord extended lower than L1 (127).
In another MRI study, the margin between the conus medullaris and the Tuffier line was measured. The conus medullaris was at the L1 (median; range of T12–L2) level in men and L1–L2 (median; range of T12–L3) in women. The Tuffier line was at L4–L5 (L3–S1) and L4–L5 (L4–5), respectively (128).
Thus, there should be a two- to four-segment difference between the Tuffier line and the conus medullaris but, because of the difficulty in correctly identifying this line and considerable differences in the extent of the conus medullaris, spinal puncture above L3 is not recommended. Indeed, seven patients with neurologic damage were reported after introducing the spinal needle at a level that was believed to be the L2–L3 level. All experienced pain during insertion of the needle. On MRI, conus damage, fluid collection, and hemorrhage were observed (129).
Previous Spinal Anesthesia
If adequate information is available about the properties (spread, duration) of a previous spinal anesthesia, this knowledge can be used to obtain a second successful spinal anesthesia. It has been observed that a subsequent spinal anesthesia using exactly the same technique and dose of local anesthetic (plain bupivacaine) in an individual behaves in manner similar to a previous one (130,131). This observation supports the fact that the properties of the patient (height, weight, CSF volume, etc.) play a role in the spread of spinal anesthetic.
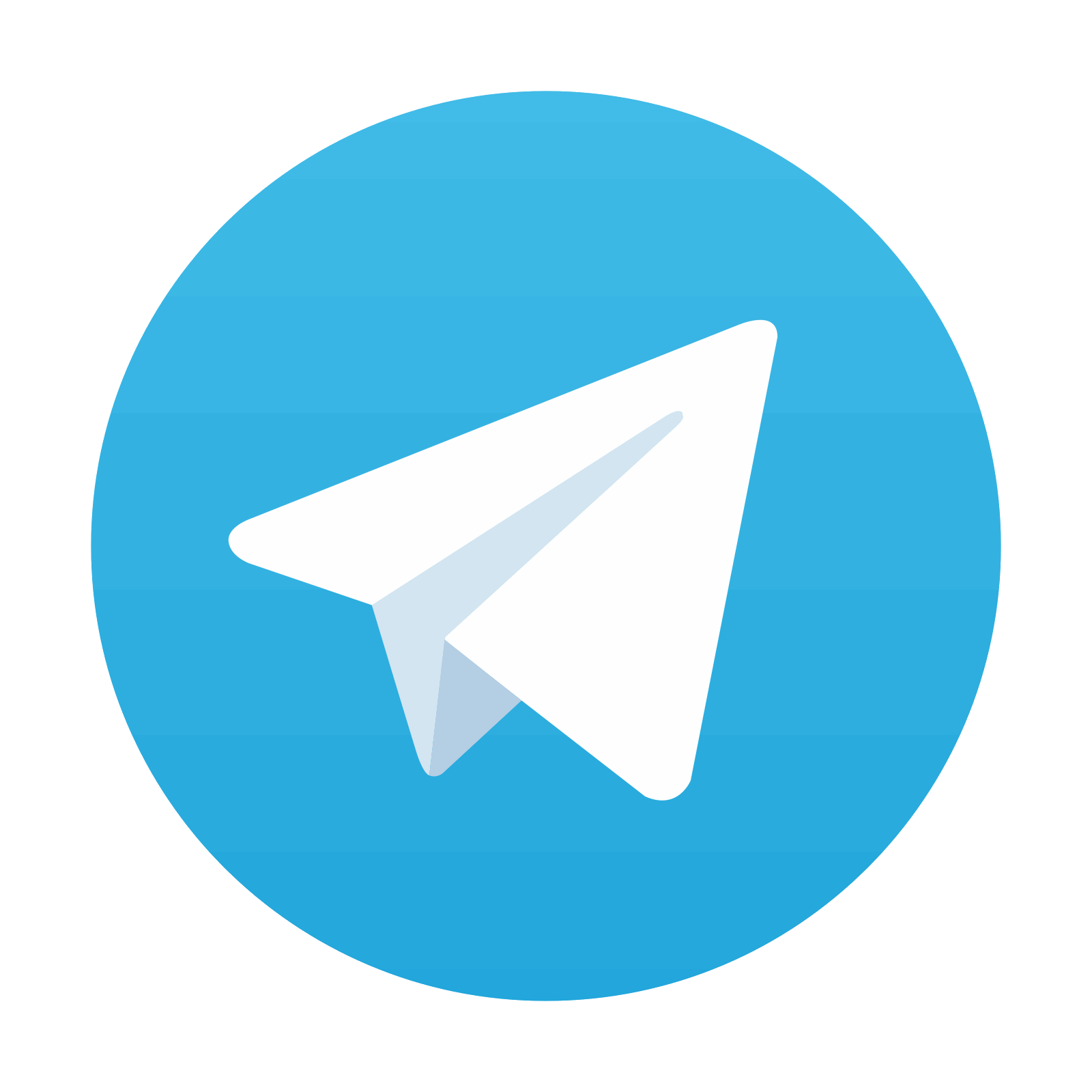
Stay updated, free articles. Join our Telegram channel

Full access? Get Clinical Tree
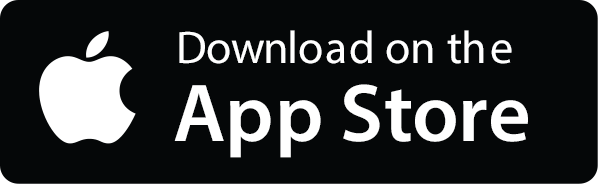
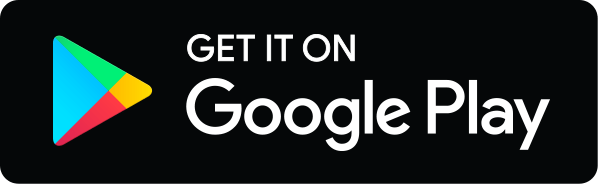
