FIGURE 14.1 A: View of a typical vertebra from above; (B) Lateral view; (C) Ligaments of the spine. (Reprinted from Stier GR, Schell RM, Cole DJ. Spinal cord injury. In: Cottrell J, Smith D, Eds. Anesthesia and Neurosurgery. 4th Ed. Philadelphia, PA: Mosby Inc., 2001, with permission.)
b. The vertebral column is stabilized by (from posterior to anterior) the supraspinous, interspinal, ligamentum flavum, posterior longitudinal, and anterior longitudinal ligaments (Fig. 14.1). The ligaments provide flexibility and limit excessive movement that could damage the cord.
c. The spinal cord begins at the foramen magnum and terminates at the conus medullaris (L2 in adults). Below the termination of the spinal cord, the lumbar and sacral roots form the cauda equina. The spinal cord is surrounded by the meninges, which are layers of tissue (the dura mater and arachnoid membranes) that have cerebrospinal fluid (CSF) between them providing further protection for the spinal cord. The anterior portion of the cord gives rise to the motor nerves. Nerves that originate posteriorly are sensory in function.
2. Blood supply
a. Anterior spinal artery. A single vessel, formed from the union of the two anterior spinal branches of the vertebral arteries, descends down the entire length of the anterior portion of the spinal cord. This artery supplies perfusion to the anterior two-thirds of the cord (the anterior column and most of the lateral column).
b. Posterior spinal arteries. Two vessels originating from the posterior inferior cerebellar arteries supply the posterior one-third of the cord (the entire posterior column and the balance of the lateral column).
c. Radicular arteries. These vessels originate from branches of the vertebral, deep cervical, intercostal, and lumbar arteries. They anastomose with the anterior and posterior spinal arteries. The artery of Adamkiewicz (arteria radicularis magna), the major radicular artery, is most commonly located in the lower thoracic or upper lumbar region and provides most of the blood supply to the lower cord distal to its level of entry.
3. Regulation of spinal cord blood flow (SCBF) (Fig. 14.2). Average SCBF is estimated at 60 mL/100 g/minute, with a three- to four-fold gray matter to white matter blood flow differential. Autoregulation maintains SCBF by altering vascular resistance in response to changes in MAP.
FIGURE 14.2 Effect of changes in PaCO2, PaO2, and MAP on SCBF. (Reprinted from Stier GR, Schell RM, Cole DJ. Spinal cord injury. In: Cottrell J, Smith D, Eds. Anesthesia and Neurosurgery. 4th Ed. Philadelphia, PA: Mosby Inc., 2001, with permission.)
a. Normally, autoregulation exists for a MAP between 60 and 120 mm Hg.
b. Failure of autoregulation occurs below a MAP of 50 mm Hg, leading to progressive tissue ischemia.
c. Above a MAP of 120 mm Hg, SCBF increases, leading to progressive tissue edema and cellular disruption.
d. The autoregulatory range of individuals who have chronic hypertension is shifted to the right (higher range of MAP).
e. Alterations in the partial pressure of carbon dioxide (PaCO2) and oxygen (PaO2) affect SCBF in the spinal cord much as they do in the brain. Between 20 and 80 mm Hg, SCBF is linearly related to PaCO2. SCBF is well maintained at varying degrees of oxygenation until PaO2 falls to 50 mm Hg; below this value, SCBF increases significantly.
II. SPINAL CORD INJURY
A. Introduction. The reported annual incidence of traumatic spinal cord injury (SCI) in the United States is estimated at 12 to 55 patients per million population, with approximately 11,000 new cases of SCI per year. The estimated prevalence of SCI is within a range of 225,000 to 296,000 cases. SCI occurs with greatest frequency in those individuals between the ages of 15 and 34 years of age, with a male-to-female ratio of approximately 4:1. Common causes of SCI include motor vehicle accidents (40% to 56%), falls (15% to 20%), violence (5% to 20%), sports injuries (5% to 16%), and other causes (5% to 10%). Among persons age 65 and older, falls cause most SCIs. SCI from spinal trauma occurs most frequently in the cervical spine (55%), followed by the thoracic spine (30%) and the lumbar spine (15%). Most injuries occur at the midcervical (C4–C6) or thoracolumbar (T12) region and are often associated with concomitant injuries. Isolated SCI is present in only 20% of cases, while additional injuries, typically of the head, thorax, and abdomen, are present in 20% to 50% of cases. It has been estimated that up to half of all patients who sustain an acute SCI have an associated head injury, while 5% to 10% of head-injured patients have an associated acute SCI. Of those individuals sustaining an SCI, the distribution of injury consists of incomplete tetraplegia, 34.5%; complete paraplegia, 23.1%; complete tetraplegia, 18.4%; and incomplete paraplegia, 17.5%. The probability of death during the first year after an SCI is 6.3%, decreasing to 1.8% during the second year. The 12-year survival rate in SCI-injured patients is 85%. Determinants of survival include Glasgow Coma Scale score on admission, age, level of injury, presence of multiple injuries, respiratory failure, cardiac complications, pulmonary emboli, and infection.
The perioperative medical care of patients during the acute phase of SCI is of extreme importance in determining the outcome. Thus, strategies that prevent further SCI, limit the extension of an existing SCI injury, or salvage even a few dermatomal levels can affect morbidity, mortality, long-term disability, quality of life, and health-care costs significantly. Surgical treatment for SCI is aimed at immobilization, operative decompression, and spinal stabilization. Care during the intermediate and chronic phases of SCI is also important.
B. Pathogenic correlates of spinal cord injury
1. Primary SCI, caused by the mechanical forces of the trauma, results in direct neuronal disruption and destruction from shearing forces, lacerations, acute stretching of axonal fibers, and sudden acceleration–deceleration injuries. Petechial hemorrhages and hematomyelia also occur. Histologic changes consist of hemorrhage and protein extravasation into the central gray matter that spread to the adjacent white matter. Primary injury rarely causes actual transection of the cord.
2. Secondary SCI is due to microvascular damage in combination with the activation of biochemical and enzymatic processes. The severity of damage is in proportion to the magnitude of the initial injury. Progressive hemorrhagic necrosis, loss of cellular membrane integrity, vasogenic and cytotoxic edema, inflammation, arachidonic acid release, lipid peroxidation, and loss of vascular autoregulation all contribute to and determine the extent of secondary SCI. This is manifest as vascular stasis, decreased SCBF, ischemia, and cell death. The traumatized areas eventually undergo cavitating necrosis and ultimately glial scar formation. Spinal cord edema is maximal at 48 hours and may persist for up to 2 weeks.
C. Anatomical correlates of spinal cord injury (Table 14.1). Flexion injuries may cause anterior subluxation or fracture dislocations of the vertebral bodies, traumatic disk herniation, and disruption of the posterior longitudinal ligament. Hyperextension injuries are associated with transverse fractures of the vertebrae, disruption of the anterior longitudinal ligament, and posterior dislocations. Vertical compression produces burst fractures, teardrop fractures, and ligamentous rupture. Rotational injuries may result in fractures of the vertebral peduncles and facets. The designation of an SCI as stable or unstable considers the potential for further spinal injury and the failure to heal properly, if untreated.
TABLE 14.1 Spinal Injury, Clinical Finding, and Indicated Treatment
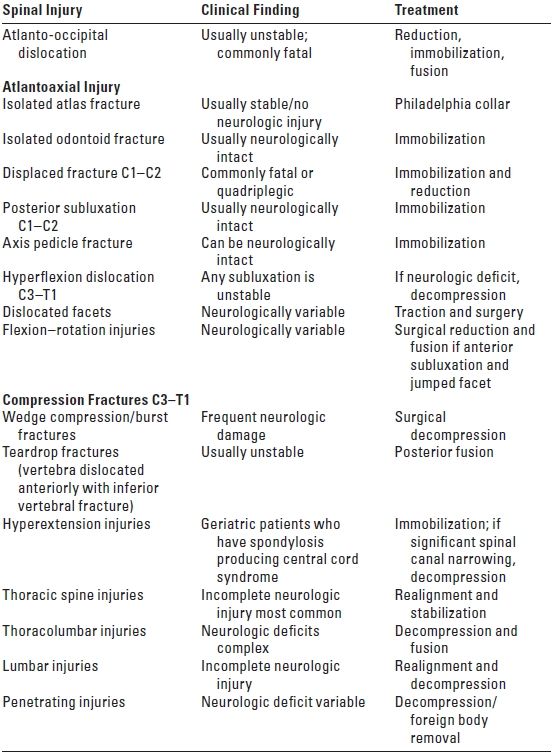
D. Clinical correlates of spinal cord injury (Table 14.2). SCI can result in complete (total loss of sensory and motor function distal to the injury) or incomplete (presence of any non-reflex function distal to the injury; see Table 14.2) loss of neurologic function. A complete SCI has less than a 10% chance of full return of normal neurologic function. An incomplete SCI has a 59% to 75% chance of recovering lost function. The degree of cardiac and respiratory dysfunction is dependent on the spinal level of the acute SCI (Table 14.3). Medical problems include the following:
TABLE 14.2 Incomplete SCI Syndromes
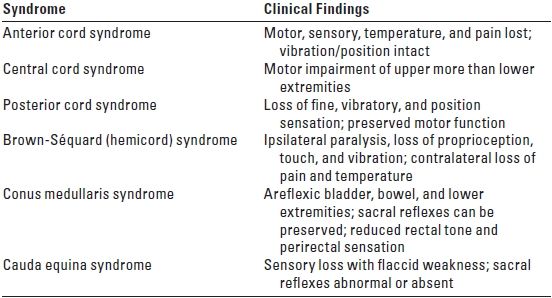
The cardiovascular effects of an acute SCI depend on the level of injury (Table 14.3). For SCIs below T6, the major problem involves varying degrees of hypotension resulting from the functional sympathectomy. With complete SCI above T6, more significant cardiovascular abnormalities occur including bradycardia, hypotension, ventricular dysfunction, and dysrhythmias.
TABLE 14.3 Level of SCI and Pulmonary/Cardiac Function
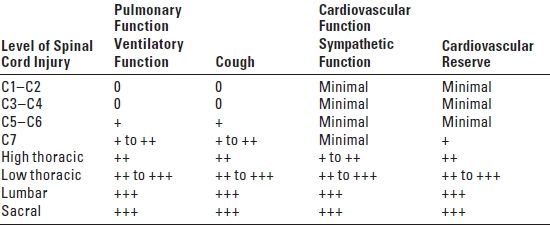
Scale is 0 (no function) to +++ (normal); SCI, spinal cord injury.
1. Spinal shock, seen most commonly with physiologic or anatomic transection of the spinal cord above C7, results from a total loss of impulses from higher central nervous system (CNS) centers as an immediate consequence of injury.
a. Spinal shock is characterized by flaccid paralysis, paralytic ileus, and loss of reflexes below the level of the lesion, visceral and somatic sensations, vascular tone, and the vasopressor reflex.
b. This syndrome, including the signs and symptoms of autonomic dysfunction, lasts from days to weeks and is prolonged by serious infection.
2. Neurogenic shock refers to the cardiovascular manifestations of SCI, particularly above T6, which include hypotension, bradycardia, and hypothermia. The higher the spinal level of injury, the more severe the physiologic derangements encountered. Neurogenic shock is caused by the loss of supraspinal sympathetic control of cardiovascular functions including coronary blood flow, cardiac contractility, and heart rate. The disruption of vascular tone and blood pressure control leads to low resting blood pressure, orthostatic hypotension, and bradycardia from unopposed vagal tone. Cardiovascular abnormalities after an acute cervical SCI include the following:
These cardiovascular derangements remain most problematic during the first 2 weeks after acute cervical SCI and may persist for up to 5 weeks after injury.
1. Bradycardia, nearly universal after complete acute cervical SCI, results from the functional sympathectomy with interruption of cardiac accelerator nerves (T1–T4) and unopposed vagal innervation. Bradycardia usually resolves over a 2- to 5-week period. More profound degrees of bradycardia, as well as cardiac arrest, may occur during stimulation of the patient (e.g., turning the patient, tracheal suctioning). Familiarity with the factors precipitating bradycardia should lead to institution of preventive interventions such as sedation, anticholinergics, 100% oxygen before suctioning, and limiting the time allowed for suctioning. Although severe bradycardic episodes may be effectively treated with atropine alone, in some instances (30%) a temporary pacemaker is necessary. In the context of traumatic hemorrhage, it is important to remember that the heart rate will be less useful in differentiating between neurogenic shock (bradycardia) and hemorrhagic shock (tachycardia). Thus, in patients who have evidence of hemorrhage, an inappropriately slow heart rate should prompt suspicion of an associated SCI.
2. Hypotension, defined as a systolic blood pressure < 90 mm Hg or systolic blood pressure of 30% below baseline, is seen in 60% to 80% of patients after acute cervical SCI. Hypotension occurs because the loss of sympathetic tone causes peripheral vasodilatation with vascular pooling of blood. Early intervention to maintain MAP at 85 mm Hg for the first 7 days after injury is recommended to preserve neurologic function as autoregulation is impaired. Hypovolemia from coexisting hemorrhagic shock should be treated with prompt blood replacement and administration of isotonic crystalloid. Strict attention to the total volume of fluid administered is warranted, however, as pulmonary edema and cardiac decompensation are more likely to occur in the setting of an acute cervical SCI.
a. If hypotension persists despite adequate fluid administration, vasopressor therapy is indicated. The vasopressor should have beta-agonist properties (e.g., dopamine or dobutamine). Supplementation with an alpha 1 agonist such as phenylephrine might be necessary. Caution should be exercised, however, when selecting a more potent alpha agonist, such as norepinephrine, because the potent vasoconstricting properties may substantially increase cardiac afterload, diminish cardiac output, and precipitate frank left ventricular failure.
b. Invasive central hemodynamic monitoring is recommended in acute cervical SCI as an aid in guiding fluid therapy. A CVP of 8 to10 mm Hg or a pulmonary artery occlusion pressure of 12 to 16 mm Hg appears to optimize spinal cord perfusion most effectively. Newer technologies that analyze the arterial pulse wave contour and record stroke volume variation are also useful as tools in guiding fluid management. In patients who have suffered multiple traumatic injuries, the presence of neurogenic shock might conceal the symptoms of hemorrhagic shock making invasive hemodynamic monitoring an appropriate choice. Operative intervention for SCI should be postponed until the patient’s hemodynamic status has been stabilized.
3. Disturbances of cardiac rhythm are common after SCI above the T6 level, and include bradycardia, primary asystole, supraventricular dysrhythmias (atrial fibrillation, re-entry supraventricular tachycardia), and ventricular dysrhythmias. An acute autonomic imbalance resulting from a disruption of sympathetic pathways in the cervical cord is causative. The arrhythmias typically resolve within 2 to 3 weeks after the SCI.
4. Left ventricular impairment has been noted in complete cervical SCI and is attributed to the functional sympathectomy and resulting autonomic imbalance. These patients are also more prone to the development of pulmonary edema.
5. Autonomic dysreflexia occurs in 85% of patients who have spinal cord transection above T6. The onset of signs and symptoms occurs 5 days to 3 weeks after injury. This syndrome is secondary to autonomic vascular reflexes, which usually begin to appear as spinal shock resolves.
a. Afferent impulses originating from cutaneous, proprioceptive, and visceral stimuli (bladder or bowel distention, childbirth, manipulations of the urinary tract, or surgical stimulation) are transmitted to the isolated spinal cord. These impulses elicit a massive sympathetic response from the adrenal medulla and sympathetic nervous system which is no longer modulated by the normal inhibitory impulses from the brain stem and hypothalamus. Vasoconstriction occurs below the level of the spinal cord lesion. Reflex activity of carotid and aortic baroreceptors produces vasodilatation above the lesion, which is often accompanied by bradycardia, ventricular dysrhythmias, and even complete heart block.
b. Common signs and symptoms include extreme hypertension, bradycardia, hyper-reflexia, muscle rigidity and spasticity, diaphoresis, pallor, flushing above the lesion, and headache. Horner’s syndrome, pupillary changes, anxiety, and nausea occur less frequently. Systolic pressures in excess of 260 mm Hg and diastolic pressures of 220 mm Hg have been reported.
c. Adverse effects of autonomic dysreflexia include myocardial ischemia, intracranial hemorrhage, pulmonary edema, seizures, coma, and death.
d. Treatment involves cessation of the offending stimulus and a change to the upright position to enhance pooling of blood in the lower extremities. Pharmacologic intervention includes direct-acting vasodilators (e.g., sodium nitroprusside), beta blockers (e.g., esmolol), combination of alpha and beta blockers (e.g., labetalol), calcium channel blocking drugs (e.g., nicardipine), and ganglionic blocking drugs. As the attacks are often paroxysmal, drugs of rapid onset and short duration are preferred.
E. Acute care of the patient after spinal cord injury. Preservation of spinal cord function involves maintaining adequate oxygenation and MAP, stabilizing the spine, decreasing spinal cord edema, and decreasing secondary biochemical processes that worsen neurologic injury. The initial care of the patient who has an SCI is as follows:
1. External splinting and immobilization. Immobilization of the spine is accomplished in the field by placing the patient on a spine board with sandbags on either side of the head to prevent rotation.
2. Neurologic examination. In patients who have sustained spinal trauma, a neurologic examination is important to establish the patient’s baseline neurologic function and to facilitate management decisions. Performed after the primary trauma survey, the neurologic evaluation includes an appraisal of mental status, an examination of the spinal column, and an assessment of the sensorimotor function of the extremities. During examination, it is important to take spinal precautions at all times (e.g., log rolling, neutral neck position). The neurologic examination should be accurately detailed in the medical record preferably by using the American Spinal Injury Association (ASIA) Impairment Scale (Fig. 14.3). The use of the ASIA chart standardizes the neurologic evaluation process and ensures the accuracy of the neurologic examination, which includes the following:
FIGURE 14.3 American Spinal Injury Association. (Reprinted from International Standards for Neurological Classification of Spinal Cord Injury, revised 2011, Atlanta, GA, 2011, with permission.)
a. Mental status: The Glasgow Coma Scale score is determined by considering the following—does the patient follow commands, open his/her eyes, track appropriately, and speak coherently? Altered mental status may signify coexisting brain injury.
b. Determination of sensorimotor function: In the ASIA evaluation method, neurologic assessment is performed by testing key muscles and sensory points. For motor testing, 10 groups of muscles are tested. The function of these muscles is graded on a 6-point scale, with 0 assigned for total paralysis and 5 points assigned for normal strength. In total, there are 100 points when the right and left sides are assessed. The second part of the ASIA neurologic examination is the sensory score, which is based on the evaluation of 28 sensory dermatomes on the right and left sides of the body. At each of these key points, both sensitivity to pin prick and to light touch are tested and scored on a scale of 0 to 2 (0—absent sensation; 1—impaired sensation; 2—normal sensation). In addition, voluntary motor contraction of the external anal sphincter is tested by digital examination. Perirectal sensation and the bulbocavernosus reflex (anal cutaneous reflex) are also tested. A normal response indicates the preservation of distal function, known as “sacral sparing.” This identifies the SCI as incomplete and is thus indicative of a more favorable prognosis.
c. The ASIA Impairment Scale is used to identify the severity of the SCI (Table 14.4). The terms sensory level and motor level are used to define the most caudal segment of the cord that has normal sensory and motor function, respectively, on both sides of the body.
TABLE 14.4 Muscle Group with Corresponding Level of Innervations
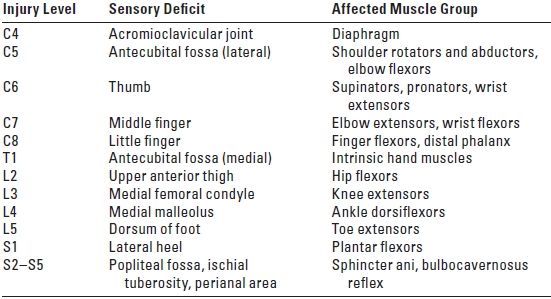
3. Radiologic evaluation. The goal of radiologic imaging after spine trauma is to recognize and determine the extent of the spinal injury, as well as to aid in prognosis. Although plain radiography has been the traditional modality used to identify spine injuries, CT is rapidly supplanting plain radiography as the primary screening modality for spinal imaging.
Regardless of the technique utilized, the results of radiologic imaging and the identified neurologic deficits are combined with knowledge of the mechanism of injury to determine the final pathologic state. The incidence of cervical spinal injury in comatose trauma patients is 7%. Therefore, radiologic assessment of the spine is essential after traumatic head injury, particularly in those individuals who also have signs and symptoms referable to a spinal injury.
a. Plain radiographs. The three-view cervical spine series composed of lateral, anteroposterior (AP), and open-mouth odontoid view, will detect most cervical spinal injuries. However, the abnormality will not be detected on the plain film in as many as 15% to 20% of patients who have a cervical SCI. When clinical suspicion is high, a more comprehensive radiologic evaluation is often indicated. For cervical spine injuries, a useful method of analysis of the plain radiographs is as follows:
1. Adequacy and quality of the occipital–cervical junction (C1–C2), C3–C7, and the C7–T1 junction.
2. Alignment of the anterior edge of the vertebral body, the posterior edge of the vertebral body, the spinolaminar junction, and the tips of the spinous processes (Fig. 14.4).
FIGURE 14.4 The lateral cervical spine. Spinal column stability after traumatic injury is often based on a system that divides the spine into four longitudinal lines from anterior to posterior, starting with the anterior edge of the vertebral body (AV), the posterior edge of the vertebral body (PV), the spinolaminar junction (SL), and the tips of the spinous process (SP). Each line provides the border for three columns (I, II, and III). Disruption of two or more of these columns indicates spinal instability.
3. Bones. Look for fractures of the vertebral body, pedicle, lamina, and spinous process.
4. Cartilage. Analyze the disk space and the facet joints.
5. Soft tissue space. Observe for edema and other abnormalities.
b. Computerized tomography (CT) has superior spatial resolution of the spine as compared with plain radiographs, and the axial format provides better evaluation of the spinal canal. CT imaging of C1 and C2 and the lower cervical spine is indicated when there are poorly visualized or suspicious areas on plain films. The negative predictive value of the combination of a normal three-view cervical spine series supplemented with CT through “suspicious” areas and areas that are difficult to visualize is 98% to100%. CT is therefore more helpful to:
1. Define bony and soft tissue abnormalities
2. Evaluate the lower C-spine
3. Measure spinal canal and neuroforaminal diameter
4. Provide detailed anatomy of the facet joints
5. Detect hematoma formation
6. Determine compression of the spinal canal and spinal stability
7. Detect a unilateral jumped facet or bone fragments in the canal or root foramen
8. Confirm complete reduction of a dislocation
9. Demonstrate hyperdense acute blood or retropulsed vertebral body fragments
c. Magnetic resonance imaging (MRI) has inherent advantages in the setting of spinal trauma, including the ability to image the spinal cord in any orientation and to visualize soft tissues and spaces. MRI is indicated within 48 hours of injury for symptomatic SCI and in comatose trauma patients who may have a cervical spine or ligamentous injury. MRI is helpful to:
1. Determine the degree of injury to the spinal cord parenchyma such as hemorrhage, edema, myelomalacia, and lacerations
2. Identify ligamentous injury, nerve root compression, and spinal canal size
3. Visualize the epidural and subarachnoid spaces
4. Detect elevation of the anterior or posterior longitudinal ligaments
5. Predict functional neurologic outcome in the subacute stage of SCI
d. Flexion–extension films performed under fluoroscopic guidance are recommended as an alternative to MRI to evaluate ligamentous stability in awake patients who have symptoms of cervical spinal injury or in comatose trauma patients.
4. Medical management. Identification of associated injuries is crucial. These include the following:
a. Airway
1. SCI presents several problems in airway management. Many maneuvers used for intubation can cause displacement and worsening of the injury, especially at the level of the cervical spine (C-spine). For this reason, all patients who have head trauma, multiple traumatic injuries, or decrease in level of consciousness should be considered as having a spinal injury until proven otherwise and the airway managed appropriately (Fig. 14.5).
FIGURE 14.5 Airway management algorithm for the patient who has suspected C-spine injury.
2. Patients who have a normal level of consciousness, an intact “gag reflex,” and a patent airway may be managed conservatively with supplemental oxygen. However, these patients should be observed closely to detect progressive loss of ventilatory ability as a result of developing diaphragmatic or intercostal paralysis. As profound reductions in forced vital capacity (FVC) and expiratory flow rates are observed immediately after injury, nearly all patients who have an acute cervical SCI will require mechanical ventilation within 24 to 48 hours of hospital admission.
3. The initial airway management of the cervical spine-injured patient includes consideration of the following issues:
a. The urgency of the need for airway intervention
b. Whether there is time available for radiologic evaluation of the spinal injury
c. The presence of associated facial injuries or soft tissue injuries
d. The presence of basilar skull fracture or midface fractures that would contraindicate nasal intubation
e. Whether or not the patient is awake and cooperative
f. The skill of the operator in using different airway techniques
4. Maintenance of a patent airway and adequate oxygenation in a patient who has a cervical spine injury may require bag and mask ventilation, an oral or nasal airway, or oral or nasotracheal intubation.
5. Direct laryngoscopy remains the method of choice for emergent airway control in patients who have confirmed or possible C-spine injuries. Mouth opening for laryngoscopy may be facilitated by removing the cervical collar and neck movement minimized by manual inline immobilization (MILI) without axial traction. During direct laryngoscopy, minimal displacement of the cervical spine typically occurs in the subaxial segments (C2–C5), while some extension occurs at the C1–C2 articulations. Thus, the upper cervical spine may be at greater risk for secondary injury during laryngoscopy than the lower cervical spine. The use of cricoid pressure may be utilized to reduce the risk of pulmonary aspiration of gastric contents.
a. Specific blades available for direct laryngoscopy have minimal differences with respect to the effects on spinal movements. However, a newer laryngoscopic device, the Glidescope, utilizes a video camera at the tip of the blade that transmits images to a display monitor. Compared with a Macintosh blade, the Glidescope improves the typical laryngeal view by one grade in patients who have cervical collars and reduces associated spine movements by 50%, although time to intubation is lengthened.
b. Neck-stabilizing cervical orthotic devices, such as soft and hard collars, do not limit spinal movements or offer significant spine protection; in addition, such collars interfere with mouth opening. As such, these collars should be removed in combination with MILI to facilitate tracheal intubation.
c. Excessive flexion and extension of the neck during maintenance of the airway and intubation might lead to catastrophic exacerbation of the spinal injury. Therefore, MILI should be utilized whenever traditional laryngoscopy is indicated. MILI is accomplished by having an assistant hold the sides of the neck and the mastoid process, preventing significant movement of the neck during direct laryngoscopy. If necessary, the airway can be opened using a careful jaw thrust technique with the head maintained in a neutral position through prevention of significant flexion and extension.
6.
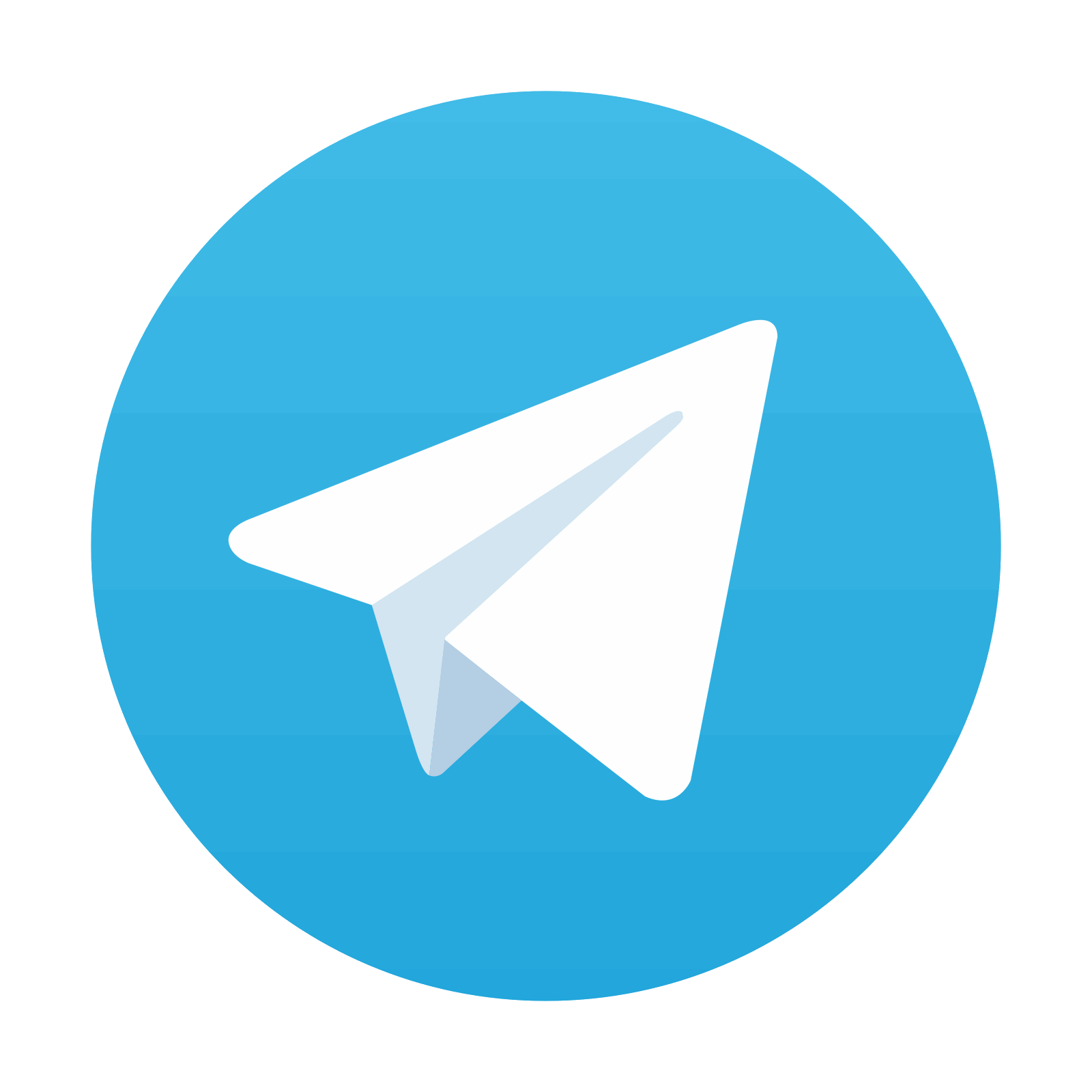
Stay updated, free articles. Join our Telegram channel

Full access? Get Clinical Tree
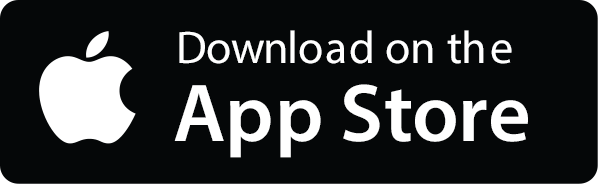
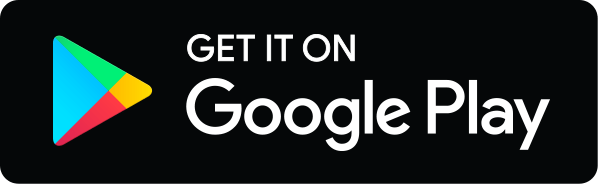