Specific Considerations with Liver Disease
Matthew C. Gertsch
Wilton C. Levine
I. HEPATIC ANATOMY
A. Liver Structure
1. The anatomic unit of the liver is lobule. The lobule is composed of hexagonal plates of hepatocytes and portal triads (terminal portal vein, hepatic artery, and bile duct branch) surrounding a central hepatic vein.
2. Hepatocytes are classified by their position in relation to their portal triad. Those closest to the triad are labeled Zone 1 cells (periportal). These cells receive the most oxygen and nutrients and are responsible for most nitrogen metabolism, oxidation, and gluconeogenesis. Zone 2 is a transitional area. Zone 3 hepatocytes (pericentral) are the farthest from the triad and are at the greatest risk for ischemic injury.
B. Hepatic Blood Supply. The liver composes only 2% of the total body mass, but it receives 20% to 25% of the cardiac output.
1. The hepatic artery supplies 25% of the total liver blood flow and 50% of the liver’s oxygen requirement.
2. The portal vein drains the stomach, spleen, pancreas, and intestine. It supplies 75% of the hepatic blood flow and 50% of hepatic oxygen.
3. Total hepatic blood flow depends greatly on venous return from the pre-portal organs. Flow in the hepatic artery is regulated by sympathetic tone and local adenosine concentration and is inversely related to portal vein flow (PVF). A reduction in PVF will lead to an increased adenosine concentration in the liver, which causes local arteriole dilation and an increase in hepatic artery flow. Total hepatic blood flow may be reduced in diseases causing increased hepatic vascular resistance (e.g., cirrhosis, infiltrative disease as in metastatic liver disease, and Budd-Chiari syndrome).
II. HEPATIC FUNCTION
A. Synthesis and Storage
1. Proteins. The normal adult liver produces 12 to 15 g of protein per day including the following:
a. Albumin is manufactured exclusively in the liver and has a half-life of approximately 20 days. It comprises 50% of all circulating plasma proteins and is the most important drug-binding protein, especially for organic acids such as penicillins and barbiturates. Albumin contributes to oncotic pressure and also serves as a carrier protein for bilirubin and hormones.
b. α1-Acid glycoprotein is an “acute phase reactant” and is responsible for binding basic drugs such as amide local anesthetics, propranolol, and opioids.
c. Pseudocholinesterase is responsible for the degradation of succinylcholine, mivacurium, and ester-type local anesthetics. In the presence of severely depressed hepatocellular function or a
genetically mediated enzyme deficiency, decreased plasma levels may cause profound clinical effects.
genetically mediated enzyme deficiency, decreased plasma levels may cause profound clinical effects.
d. All proteinaceous clotting factors are produced in the liver with the exception of factor VIII, which is produced in the vascular endothelium. Synthesis of factors II (prothrombin), VII, IX, and X as well as proteins C, S, and Z are vitamin K dependent; deficiency of vitamin K or disturbances of liver function may lead to deficiencies of clotting factors and excess bleeding. Factor VII has the shortest half-life (4 to 6 hours) and therefore declines at nearly the same rate as protein C (9 hours). Because factor VII is located in the extrinsic pathway, which is assessed by the prothrombin time (PT), this early decline in the factor VII activity prolongs the PT even though other pathways have not yet been affected. Factors II, IX, and X have half-lives of approximately 60, 24, and 36 hours, respectively. Consequently, it takes 4 to 6 days before their activities are at a minimum level, and an antithrombotic effect is achieved that correlates with the INR.
2. Carbohydrates. The liver is actively involved in the homeostatic regulation of plasma glucose levels (glycogen synthesis and gluconeogenesis). The normal liver can store enough glycogen to provide glucose during a fast of 12 to 24 hours. After that time, glucose is derived by gluconeogenesis from amino acids, glycerol, and lactate.
3. Lipids. Most of the body’s lipoproteins, as well as cholesterol and phospholipids, are formed in the liver.
4. Heme and bile.
a. The liver is the primary erythropoietic organ of the fetus and continues to be a major site of hematopoiesis until approximately 2 months of age. In healthy adults, the liver is responsible for 20% of heme production. Abnormalities in heme synthesis can result in porphyria.
b. The liver forms approximately 800 mL of bile per day. Bile salts are detergents that aid in absorbing, transporting, and excreting lipids. Bile also carries metabolic waste products and drug metabolites to the intestine from the liver. As an emulsifier, bile facilitates fat absorption by the small intestine. Failure to produce or release bile causes jaundice and an inability to absorb fat and fat-soluble vitamins (A, D, E, and K) and can result in steatorrhea, vitamin deficiencies, and coagulopathy.
B. Degradation
1. Proteins. The liver is the major site of protein degradation where amino acids are broken down through a process that generates urea for the elimination of ammonia. Patients with liver disease may lack the ability to form urea, resulting in rapidly rising plasma ammonia levels and hepatic encephalopathy.
2. Steroid hormones. Cholesterol is degraded principally by the liver, and its by-products serve as a substrate for the production of bile salts, steroid hormones, and cell membranes. The liver also serves as the major site of steroid hormone degradation, and hepatic failure results in steroid excess. Elevations of serum aldosterone and cortisol result in increased resorption of sodium and water, and loss of potassium in the urine, contributing to the edema, ascites, and electrolyte abnormalities frequently seen with liver disease. Decreased metabolism of estrogens and impaired conversion to androgens causes other clinical stigmata of liver disease including spider angiomata, gynecomastia, palmar erythema, and testicular atrophy.
3. Heme and bile. Bilirubin that is bound to albumin is delivered to hepatocytes where it then undergoes conjugation with glucuronic acid, which makes it water soluble. These products are excreted within the bile and are eliminated via the feces or urine.
C. Drug Metabolism
1. The liver has a dual blood supply, receiving blood from the hepatic artery and the portal veins. The hepatic extraction ratio (HER) is a measure of the efficiency of the liver in removing drugs from hepatic inflow. It is defined as the fraction of a drug concentration flowing into the liver that is removed through hepatic elimination and metabolism. High-extraction drugs are absorbed from the gut and delivered to the liver, where they may be metabolized before reaching the systemic circulation (first-pass metabolism). Drugs with a high extraction ratio and significant first-pass metabolism have low oral bioavailability.
2. Hepatic clearance = HER × hepatic blood flow rate. Some drugs are extensively metabolized by the liver and have an HER of close to 1.0 (propofol). In these cases, hepatic metabolism depends predominantly on hepatic blood flow, and moderate changes in hepatic function have little effect on clearance. Other drugs have an HER of less than 1.0, and clearance depends on both hepatic function and changes in hepatic blood flow.
3. Protein binding. The degree of protein binding depends on the specific drug’s affinity for protein binding and the protein concentration. Decreased concentrations of plasma proteins, as often seen in liver disease, will result in a greater proportion of unbound drug. Only free, unbound drug is pharmacologically active and is available for conversion to a less active form. A decrease in plasma proteins may therefore affect the potency and/or elimination of a drug.
4. Volume of distribution is often increased in patients with liver disease, and portal-systemic shunting permits orally administered drugs to bypass the liver, reducing the first-pass effect. Both can alter drug effects and metabolism.
5. Enzyme induction/cytochrome P450 enzymes are produced in the liver and are responsible for much drug metabolism. Certain drugs such as barbiturates, ethanol, and phenytoin induce cytochrome P450 enzymes. Induction of cytochrome P450 increases tolerance to a drug’s effect and tolerance to other drugs that are also metabolized by the cytochrome P-450 system.
6. Two steps are involved in hepatic drug elimination:
a. Phase I reactions change a compound’s structure via oxidation, reduction, or hydrolysis (primarily via cytochrome P450 enzymes). The products of this phase may be metabolically active. Drugs that have a high affinity for the P450 complex (e.g., ciprofloxacin) may decrease the metabolism of concurrently administered drugs.
b. Phase II reactions may or may not follow Phase I reactions and are enzymatically enhanced conjugations with glucuronide, sulfate, taurine, or glycine. These conjugations increase the water solubility of the metabolite for excretion via urine.
III. METABOLISM OF ANESTHETICS
A. Intravenous Anesthetics
1. Induction agents
a. Propofol is metabolized by the liver (HER ˜ 1) to water-soluble compounds that are excreted by the kidneys. Extrahepatic metabolism of propofol also contributes to total propofol clearance.
b. Barbiturates have a duration of action that is determined by redistribution and hepatic metabolism and may have prolonged effects in patients with liver disease. Hypoalbuminemia, as seen in patients with altered liver function, may reduce protein binding and increases the free active fraction of these drugs. Therefore, barbiturates must be titrated carefully in patients with liver disease.
c. Ketamine is metabolized by the hepatic microsomal enzyme system to norketamine, which has approximately 30% of the activity of the parent drug. Ketamine has an HER of approximately 1.
d. Etomidate is metabolized by the liver through ester hydrolysis to inactive metabolites. Similar to ketamine, etomidate has a high HER, so clearance is affected by conditions that reduce hepatic blood flow. Recovery from an initial induction dose is primarily due to rapid redistribution.
2. Benzodiazepines and opioids are metabolized primarily by the liver and have significantly increased half-lives in patients with liver disease. Additionally, they have increased potency in cases of hypoalbuminemia as these drugs, which are usually protein bound, are now free in the plasma resulting in higher drug levels. This issue may confound the clinical picture of hepatic encephalopathy and should be titrated carefully.
3. Neuromuscular blocking agents. Patients with liver disease often demonstrate resistance to nondepolarizing neuromuscular blockers, probably because of increased volume of distribution or increased neuromuscular receptors. However, a slower elimination time may decrease the requirement for maintenance dosing.
a. Pancuronium is excreted primarily in the urine but 10% to 20% is metabolized in the liver. The 3-hydroxymetabolite has neuromuscular blocker activity. Approximately 30% of pancuronium is eliminated through hepatobiliary mechanisms, and its effect may be prolonged in patients with biliary obstruction or cirrhosis.
b. Intermediate-acting neuromuscular blocking drugs, vecuronium and rocuronium, are highly dependent on hepatobiliary excretion and metabolism (both are excreted, 50% unchanged, in the bile). This translates to a decreased clearance and a prolonged effect in patients with liver disease. Vecuronium undergoes hepatic metabolism to several compounds, one of which is 3-desacetylve-curonium that 50% of vecuronium’s neuromuscular blocker activity. Cisatracurium and atracurium are degraded via Hofmann elimination and are unaffected by liver disease.
c. Succinylcholine and mivacurium (not available in the United States) are completely metabolized in the plasma by pseudocholinesterase. Cholinesterase production may be depressed in severe liver disease, and its duration may be prolonged in patients with hepatic dysfunction.
IV. LIVER DISEASE
A. Liver disease is classified by time course and severity.
1. Parenchymal
a. Acute hepatocellular injury has many etiologies, including viral infection (hepatitis A, B, C, D, and E; Epstein-Barr virus; cytomegalovirus; herpes simplex virus; ECHO virus; and coxsackievirus), drugs, chemicals and poisons (including alcohol, halothane, phenytoin, propylthiouracil, isoniazid, tetracycline, and acetaminophen), and
inborn errors of metabolism (e.g., Wilson disease and α1-antitrypsin deficiency).
inborn errors of metabolism (e.g., Wilson disease and α1-antitrypsin deficiency).
b. Chronic parenchymal disease may be associated with varying degrees of functional impairment. Cirrhosis may result from many insults, including chronic active hepatitis, alcoholism, hemochromatosis, primary biliary cirrhosis, and congenital disorders. End-stage hepatic fibrosis causes significant resistance to portal blood flow, leading to portal hypertension and esophageal varices. Further complications from the combination of portal hypertension and decreased hepatic function include ascites, coagulopathy, gastrointestinal bleeding, and encephalopathy.
2. Cholestasis occurs most frequently in cholelithiasis and acute or chronic cholecystitis. Primary biliary cirrhosis and primary sclerosing cholangitis also begin as cholestatic diseases, ultimately leading to parenchymal damage and liver failure. Hyperbilirubinemia is an important marker for hepatobiliary disease. Unconjugated hyperbilirubinemia is due to excess bilirubin production (e.g., massive transfusion, absorption of large hematomas, or hemolysis) or impaired uptake of unconjugated bilirubin by the hepatocyte (e.g., Gilbert syndrome). Conjugated hyperbilirubinemia generally occurs with hepatocellular disease (e.g., alcoholic or viral hepatitis and cirrhosis), disease of the small bile ducts (e.g., primary biliary cirrhosis and Dubin-Johnson syndrome), or obstruction of the extrahepatic bile ducts (e.g., pancreatic carcinoma, cholangiocarcinoma, and gallstones).
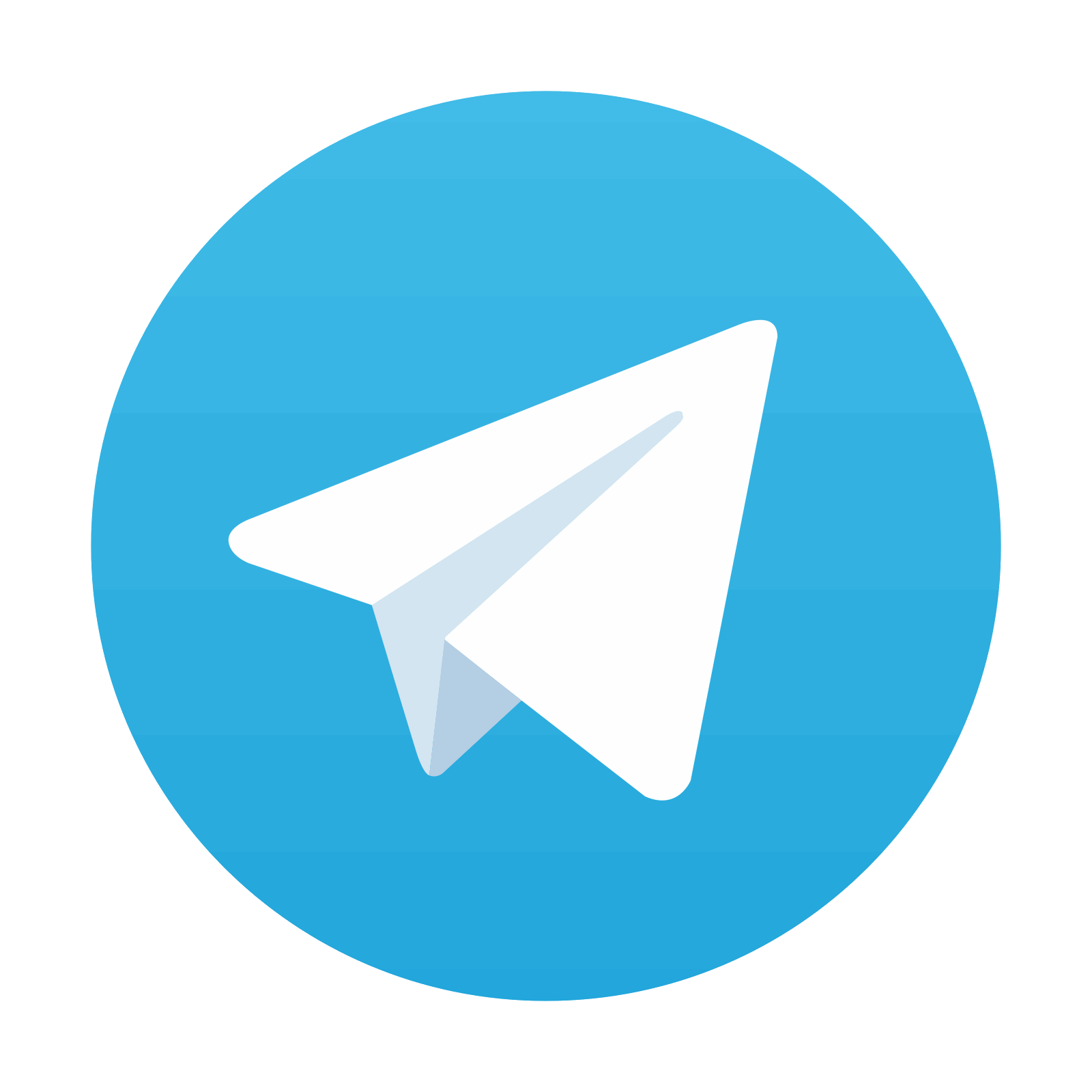
Stay updated, free articles. Join our Telegram channel

Full access? Get Clinical Tree
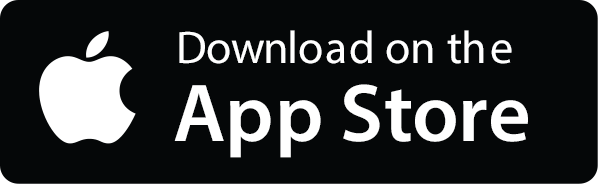
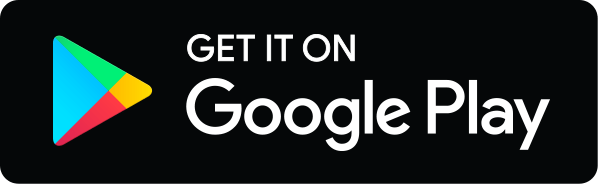