Thoracic paravertebral block (TPVB) is the technique of injecting local anesthetic alongside the thoracic vertebral body close to where the spinal nerves emerge from the intervertebral foramen. This produces unilateral (ipsilateral), segmental, somatic, and sympathetic nerve blockade in multiple contiguous thoracic dermatomes, 1,2 which is effective for managing acute and chronic pain of unilateral origin from the thorax and abdomen. 2 TPVB can also be used for surgical anesthesia in patients undergoing inguinal herniorrhaphy 3 and breast surgery 4–6 with improved postoperative outcomes. 2,5 TPVB is traditionally performed using surface anatomical landmarks. 2 Recently there has been an increase in interest in the use of ultrasound for peripheral nerve blocks, 7–9 including TPVB. 10–18 However, published data on ultrasound-guided (USG) TPVB are limited. 10–20 This chapter describes the sonoanatomy relevant for USG TPVB.
The thoracic paravertebral space (TPVS) is a wedge-shaped space 2,21 that lies on either side of the vertebral column (Fig. 11–1). It is wider on the left than on the right. 22 The parietal pleura forms the anterolateral boundary. The base is formed by the vertebral body, intervertebral disc, and the intervertebral foramen with its contents (Fig. 11–1). 21,23 The superior costotransverse ligament (SCTL), which extends from the lower border of the transverse process above to the upper border of the transverse process below (Figs. 11–2 and 11–4), forms the posterior wall of the TPVS. Also interposed between two transverse processes is the intertransverse ligament (Figs. 11–2 and 11–4). The SCTL is continuous laterally with the internal intercostal membrane, which is the medial extension of the internal intercostal muscle, medial to the angle of the rib (Fig. 11–4). The apex of the TPVS is continuous with the posterior intercostal space lateral to the tips of the transverse processes (Fig. 11–4). 21,23
Interposed between the parietal pleura anteriorly and the superior costotransverse ligament posteriorly is a fibroelastic structure, 24 the “endothoracic fascia” 25–30 (Figs. 11–1 and 11–2), which is the deep fascia of the thorax 2,25–27 and lines the internal aspect of the thoracic cage (Figs. 11–5 and 11–6). 24–30 The presence of the endothoracic fascia in the TPVS was until recently ignored in the paravertebral literature. We have drawn attention to the presence of the endothoracic fascia in the TPVS 27 and proposed that it may play a role in explaining the variable expressions of a TPVB. 27 In the paravertebral location, the endothoracic fascia is loosely applied to the ribs 23 (Fig. 11–2) and fuses medially with the periosteum at the midpoint of the vertebral body 28 (Fig. 11–1). There is an intervening layer of loose areolar connective tissue, “the subserous fascia,” 25–28 between the parietal pleura and the endothoracic fascia (Figs. 11–1 and 11–2). The endothoracic fascia therefore divides the TPVS into two potential fascial compartments, 30 the anterior “extrapleural paravertebral compartment,” and the posterior “subendothoracic paravertebral compartment” (Figs. 11–1 and 11–2). 2,27,30 The TPVS contains fatty tissue 2,23,24 within which lie the intercostal (spinal) nerve, the dorsal ramus, intercostal vessels, rami communicantes, and anteriorly the sympathetic chain (Figs. 11–1 and 11–5). 2,23 The spinal nerves in the TPVS are segmented into small bundles lying freely among the fat and devoid of a fascial sheath, which make them susceptible to local anesthetic block. 31 The intercostal nerve and vessels are located behind the endothoracic fascia, 2,30,32,33 and the sympathetic trunk is located anterior to it 2,28,30,33 in the TPVS (Figs. 11–1 and 11–5).
The TPVS is continuous with the epidural space medially via the intervertebral foramen, 23,34–36 the intercostal space laterally, 26,28,31,34,35,37,38 and the contralateral TPVS via the epidural 23 and prevertebral space. 2,26,27,29 The cranial extension of the TPVS is still not defined, but we have observed direct paravertebral spread of radio-opaque contrast medium from the thoracic to the cervical region (unpublished data) indicating that there is a direct anatomical continuity between the thoracic and cervical paravertebral regions. Ipsilateral Horner syndrome after thoracic paravertebral injections has also been reported. 29,36,39,40 The anatomical pathway for cranial spread of an injectate from the thoracic to the cervical paravertebral space is still not clear.
The caudal boundary of the TPVS is formed by the origin of the psoas major muscle, 41 and inferior (lumbar) spread through the TPVS is thought to be unlikely. 41 Ipsilateral lumbar spinal nerves are also occasionally involved after a lower thoracic paravertebral injection 1,42 Saito and colleagues have demonstrated ipsilateral thoracolumbar spread of colored dye in cadavers. 43 We have also reported ipsilateral thoracolumbar anesthesia and radiological spread of contrast below the diaphragm. 44 These observations challenge the concept of lumbar nerve root sparing following TPVB. 41 The exact mechanism for the ipsilateral thoracolumbar spread of local anesthetic or contrast medium is not clear, but we have proposed that it occurs via the subendothoracic fascial compartment 44 to the retroperitoneal space anterior to the psoas major and quadratus lumborum muscle where the ilioinguinal and iliohypogastric nerves are located (Fig. 4-50). 44
Figs. 11–11 to 11–14.
FIGURE 11–13
Transverse T2-weighted MRI of the thoracic spine showing the anatomical relationship of the inferior articular process of the vertebra to the intervertebral foramen (IVF) and the thoracic paravertebral space (TPVS). Note the spinal nerve root as it exits the IVF. SP, spinous process; VB, vertebral body; PSM, paraspinal muscles; SCTL, superior costotransverse ligament.

Position:
Patient: An ultrasound scan of the thoracic paravertebral region can be performed in the transverse (axial scan) or longitudinal (sagittal scan) axis with the patient in the sitting (Fig. 11–15), lateral decubitus (Fig. 11–16), or prone position. The prone position is useful in patients presenting for a chronic pain procedure when fluoroscopy can also be used in conjunction with ultrasound imaging. Currently there are no data demonstrating an optimal axis or position for the ultrasound scan or the paravertebral injection. It is often a matter of individual preference and experience.
Operator and ultrasound machine: The operator sits or stands behind the patient, and the ultrasound machine is placed directly in front on the contralateral side (Fig. 11–17) for an USG TPVB.
Transducer selection: The transducer used for the ultrasound scan depends on the body habitus of the patient. High-frequency ultrasound provides better resolution than low-frequency ultrasound, but its penetration is poor. Moreover if one has to scan at a depth using high-frequency ultrasound, then the field of vision is also significantly narrow. Under such circumstances it may be preferable to use a low-frequency curved array transducer (5–2 MHz) with a divergent beam and a wider field of vision. Published data suggest that a high-frequency linear transducer (13–6 MHz) is frequently used for scanning the thoracic paravertebral region. 10,11,14,18 This may be because the transverse process, costotransverse ligament, and the pleura in the midthoracic region are located at a relatively shallow depth and lend themselves to ideal conditions for imaging with a high-frequency linear array transducer. However, ultrasound imaging of the TPVS is not similar at all thoracic levels, and high-frequency transducers are generally not suitable in the upper thoracic region. Recently we have used a low-frequency curved array transducer (5–2 MHz) to perform a transverse scan of the thoracic paravertebral region (at all levels) with great success (Fig. 11–17, see details later).
Sonoanatomy:
Transverse sonoanatomy of the thoracic paravertebral region:
A transverse scan of the thoracic paravertebral region can be performed using a linear (high-frequency) or curved (low-frequency) array transducer. In slim individuals a high-frequency linear array transducer will suffice, but in those with a larger body habitus, a curved array transducer is preferable. The high-frequency linear array transducer is positioned lateral to the thoracic spinous process at the target level (Figs. 11–15 and 11–18). On a transverse sonogram the paraspinal muscles are clearly delineated and lie superficial to the transverse process (Figs. 11–19 to 11–21). The transverse process is seen as a hyperechoic structure, anterior to which there is a dark acoustic shadow that completely obscures the TPVS (Figs. 11–19 and 11–20). Lateral to the transverse process, the hyperechoic pleura that moves with respiration and exhibits the typical “lung sliding sign,” 45 which is the sonographic appearance of the pleural surfaces moving relative to each other within the thorax. Comet tail artifacts, which are reverberation artifacts, may also be seen deep to the pleura and within the lung tissue, and are often synchronous with respiration. 45 A hypoechoic space is also seen between the parietal pleura and the internal intercostal membrane (Figs. 11–19 to 11–21), which is the medial extension of the internal intercostal muscle and is continuous medially with the superior costotransverse ligament (SCTL, Fig. 11–4). This hypoechoic space represents the medial limit of the posterior intercostal space or the apex of the TPVS, and the two communicate with each other (Figs. 11–19 to 11–21). Therefore, local anesthetic injected medially into the TPVS can often be seen to spread laterally to distend this space; vice versa, local anesthetic injected laterally into the posterior intercostal space can also spread medially to the paravertebral space and is the basis of the intercostal approach for USG TPVB 10,18 where the needle is inserted in the plane of the ultrasound beam from a lateral-to-medial direction. From the scan position described earlier (ie, over the transverse process), if one now slides the transducer slightly cranially or caudally, it is possible to perform a transverse scan of the paravertebral region with the ultrasound beam being insonated between the two transverse processes (intertransverse space) and over the inferior articular process medially (Fig. 11–22). The ultrasound signal is now not impeded by the transverse process or the costotransverse junction, and parts of the parietal pleura and the “true” TPVS can be faintly visualized (Fig. 11–23). However, one must note that the inferior vertebral notch and the intervertebral foramen are located immediately anterior to the inferior articular process (Figs. 11–22 and 11–23). The SCTL, which forms the posterior border of the TPVS, is also visible and it blends laterally with the internal intercostal membrane, which forms the posterior border of the posterior intercostal space (Fig. 11–23). The communication between the TPVS and the posterior intercostal space is also clearly visualized (Figs. 11–19 and 11–23).
A low-frequency (5–2 MHz) curved array transducer (authors’ choice) can also be used to perform a transverse scan of the thoracic paravertebral region and USG TPVB. To the best of our knowledge there are limited published data describing the use of a low-frequency ultrasound transducer for sonography during TPVB, 17 and there are no published data describing the detailed sonoanatomy of the thoracic paravertebral region using a low-frequency curved array transducer. Our preliminary experience is that satisfactory ultrasound images of the paravertebral region are obtained using a low-frequency transducer. Also the wide field of vision produced by the divergent ultrasound beam is an added advantage when compared to the narrow rectangular field of view produced by a linear array transducer during USG TPVB. Furthermore the ability to image at a depth with a low-frequency curved array transducer is an advantage in the upper thoracic region because the thoracic paravertebral space is at a greater depth. Using a curved array transducer the transverse scan can be performed with the ultrasound beam being insonated at four different locations (Fig. 11–24): (1) midline over the spinous process, (2) at the level of the rib and costotransverse articulation/junction, (3) at the level of the transverse process, and (4) at the level of the articular process. Corresponding cadaver anatomical sections are presented in Figs. 11–25 to 11–27 to demonstrate the anatomy visualized during the ultrasound scan.
Each of these four ultrasound scan windows produces a distinct sonogram reflecting the different osseous and musculoskeletal structures that are visualized in the sonograms. On a transverse sonogram in the midline (position 1, Fig. 11–24), the spinous process is visualized as a bright hyperechoic dot with a corresponding acoustic shadow anteriorly (Fig. 11–28). Due to the steep caudal angulation of the thoracic spinous processes in the midthoracic region, the spinous process that is visualized on the sonogram arises from the vertebra above rather than that from which the transverse process, lamina, and the articular process arise (Fig. 11–29). Because the spinous process and transverse process cast a large acoustic shadow, visualization of the paravertebral anatomy is limited in this ultrasound scan window. Also the acoustic shadow of the spinous process, lamina, transverse process, and ribs produce a sonographic pattern that we refer to as the “flying swan sign” due to its close resemblance to a swan in flight (Fig. 11–30).
With the ultrasound transducer positioned slightly laterally (position 2, Fig. 11–24), the hyperechoic outlines of the lamina, transverse process, and the rib with their corresponding acoustic shadows are clearly delineated (Fig. 11–31). However, unlike the transverse process of the lumbar vertebra, which are more or less at right angles to the vertebral body, the transverse processes in the thoracic spine are directed posteriorly (Fig. 11–32), and this posterior angulation can be clearly delineated in the transverse sonogram (Fig. 11–31). Once the transverse process, costotransverse articulation, and the rib are identified, one can gently slide or tilt the transducer caudally until the acoustic shadow of the rib is no longer visualized (position 3, Fig. 11–24), and the hyperechoic outline of the lamina and transverse process with their acoustic shadow are seen (Fig. 11–33). Lateral to the transverse process, the hyperechoic pleura and lung are visualized anteriorly, the thick hyperechoic SCTL posteriorly, and the hypoechoic apical part of the TPVS is interposed between the two (Fig. 11–33). If one now gently slides or tilts the ultrasound transducer slightly caudally (position 4, Fig. 11–24), the acoustic shadow of the transverse process disappears, and the hyperechoic articular process (inferior) with its acoustic shadow is seen medially (Fig. 11–34). As in the ultrasound scan at the level of the transverse process (Fig. 11–33), the SCTL, parietal pleura, lung, and the apical part of the paravertebral space are also clearly delineated. However, because the acoustic shadow of the transverse process is no longer present, outlines of the true TPVS can now be visualized (Fig. 11–34). Currently the majority of the published data describing the use of a transverse scan for TPVB have used the ultrasound scan window at the level of the transverse process (position 3, Fig. 11–24), 17,18,20 and there are limited published data describing the use of the transverse ultrasound scan window at the level of the articular process for TPVB. Because there is less bony obstruction through the intertransverse space and at the level of the articular process (position 4, Fig. 11–24), it is our preferred route for imaging and needle insertion during an USG TPVB. However, ultrasound visibility of the paravertebral anatomy is more challenging in the upper thoracic region (Figs. 11–35 to 11–37). This may be related to the increased depth to the paravertebral space and anisotropy, from the pleura reflecting away from the paravertebral space, in the upper thoracic region (Fig. 11–36). Despite some of these limitations, it is possible to perform a transverse scan of the TPVS at all segments of the thoracic spine for TPVB (Figs. 11–35 to 11–44). We have successfully used this approach for both single-injection and multi-injection TPVB at all levels of the thoracic spine.
Sagittal sonoanatomy of the thoracic paravertebral region:
Published data on sagittal sonography of the thoracic paravertebral region in the clinical setting are limited and have been described with the use of a high-frequency linear array transducer. 11,15 During a sagittal scan of the thoracic paravertebral region, the ultrasound transducer is positioned 2 to 3 cm lateral to the midline (paramedian) with its orientation marker directed cranially (Figs. 11–45 to 11–47). On a sagittal sonogram the transverse processes are seen as hyperechoic and rounded structures deep to the paraspinal muscles, and they cast an acoustic shadow anteriorly (Fig. 11–48). In between the acoustic shadows of two adjacent transverse processes, an acoustic window is produced by reflections from the SCTL and intertransverse ligaments, the paravertebral space and its contents, the parietal pleura, and lung tissue (in a posterior-to-anterior direction) (Fig. 11–48).
Ultrasound visibility of the paravertebral structures is relatively poor in a true sagittal scan (Figs. 11–49 and 11–50), and this is true with both high-frequency (Fig. 11–49) and low-frequency (Fig. 11–50) transducers. This may be due to the loss of spatial resolution at the depth at which the paravertebral structures are located. Also anisotropy from the ultrasound beam not being at right angles to the pleura due to its anteromedial reflection close to the vertebral bodies (Fig. 11–46) may play a part. Ultrasound visibility of the paravertebral structures can be improved by gently tilting the ultrasound transducer laterally (ie, outward) during the sagittal scan (paramedian sagittal oblique axis, Figs. 11–51 and 11–52). This maneuver improves imaging by reducing the distance from the skin to pleura (reduced attenuation), and the ultrasound beam is also more at right angles to the pleura (reduced anisotropy (Fig. 11–51). It is difficult to define an optimal angle of lateral tilt for the paramedian sagittal oblique scan, but in clinical practice we recommend that one should gently tilt the transducer outward (laterally) until the parietal pleura is clearly visualized (Fig. 11–52). A pitfall of the lateral tilt maneuver is that one may see the same result if the ultrasound transducer is inadvertently manipulated or tilted too far laterally so that it is now insonating the rib and the posterior intercostal space (Figs. 11–53 and 11–54) instead of the transverse process and the apical part of the paravertebral space. The clinical implication is that one may unknowingly perform a posterior intercostal injection instead of a paravertebral injection, and depending on the approach used the potential for pleural puncture may be greater with the intercostal injection. Also segmental spread of anesthesia is limited with an intercostal injection. Therefore, it is important to differentiate the transverse process (Fig. 11–55) from a rib (Fig. 11–56) in the sagittal sonogram of the thoracic paravertebral region (Fig. 11–57).
We are not aware of any published data validating the sonoanatomy of the thoracic paravertebral region, but it is our experience that there is good correlation between structures that are visualized in a thoracic paravertebral sonogram and that in corresponding anatomical sections, CT, and MRI images of the thoracic paravertebral region (Figs. 11–58 to Fig. 11–60). However, irrespective of the plane of ultrasound imaging, we still have not been able to delineate the intercostal nerve or its branches with currently available ultrasound technology. The intercostal blood vessels are more readily visualized close to the inferior border of the transverse process using Color or Power Doppler ultrasound (Figs. 11–61 and 11–62).
FIGURE 11–15
Transverse ultrasound scan of the thoracic paravertebral region with the patient in the sitting position. Note the position of the ultrasound transducer (linear) relative to the spine.

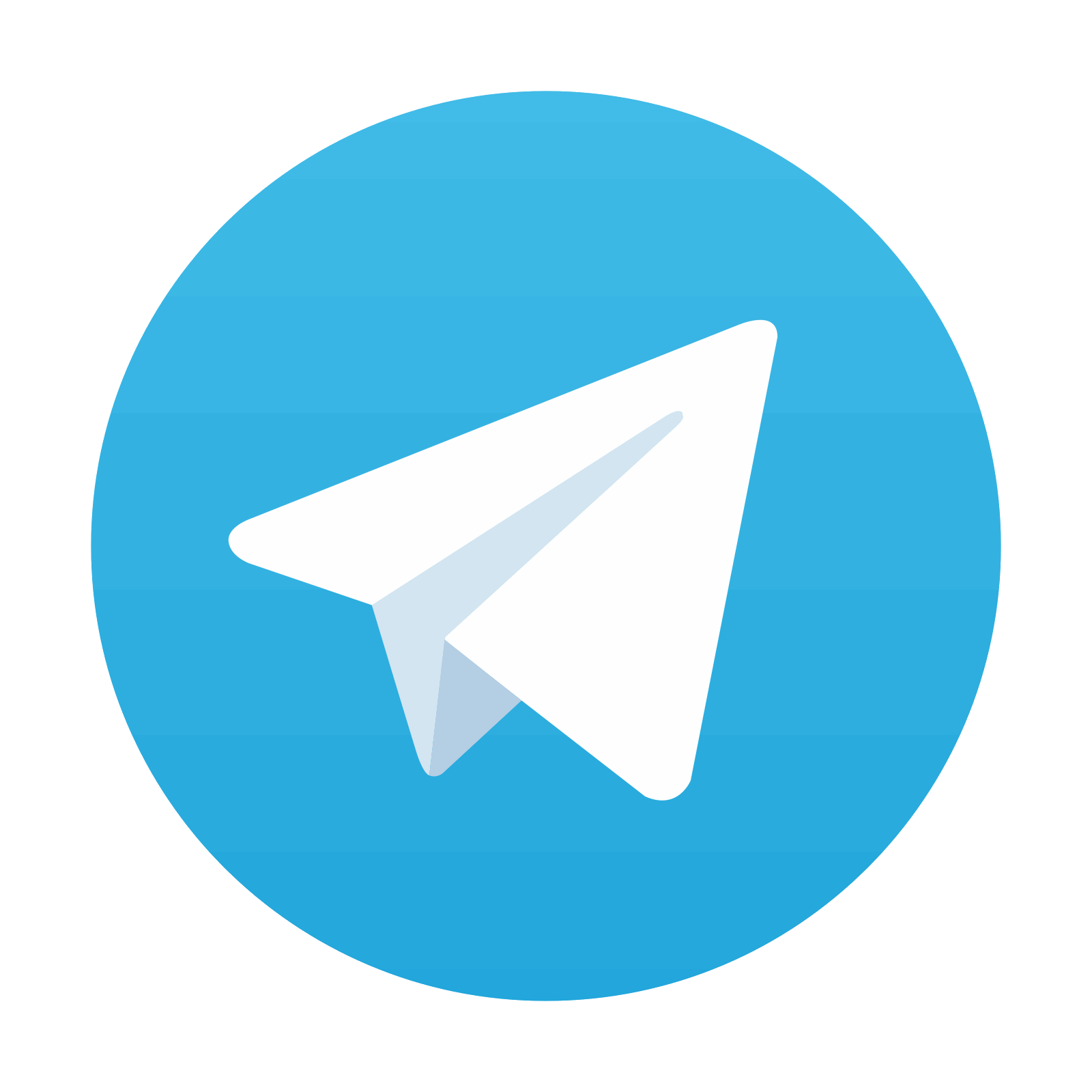
Stay updated, free articles. Join our Telegram channel

Full access? Get Clinical Tree
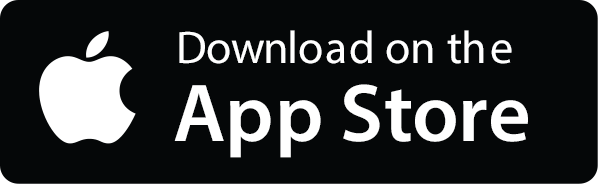
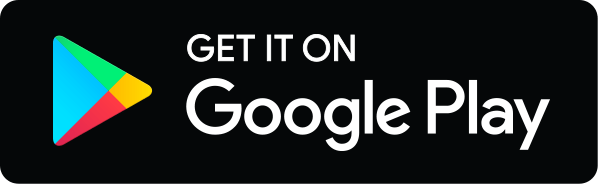
