Introduction
The central nervous system (CNS) is a complicated network of neurons that integrates a myriad of sensory input from both inside and outside the body and effects motor responses. The CNS consists of neurons, oligodendrites, and support cells such as microglia. Abnormal CNS development can result in devastating injury to young children, making the administration of general anesthesia very challenging. In order to successfully care for children undergoing neurosurgical procedures, it is essential to understand the interaction between the disordered CNS and the anesthetic milieu. In this chapter we review the general principles of anesthetic management of infants and neonates undergoing neurosurgical procedures. The purpose of this chapter is not to give recipes for treating infants and neonates for various neurosurgical procedures. Rather, we hope to give the reader an understanding of the variety of anesthetic considerations when caring for babies that are neurosurgical patients.
Development of the Central Nervous System
Many of the neurosurgical conditions seen in infants occur because of abnormal fetal development. Thus a basic understanding of the embryologic development of the nervous system is helpful to comprehend the variety of neurologic conditions that affect young infants and neonates.
Beginning of the Nervous System
During the third week of fetal development, the ectoderm differentiates into the neuroplate. This neuroplate folds upon itself to form the neural groove. There are two neural folds which form along each side of the neural groove. Neurulation begins at the fourth week of development, when the neural folds fuse dorsally to create the neural tube. The spinal ganglia, cranial nerves, and components of the peripheral and autonomic nervous system are derived from neural crest cells which are located adjacent to the neural folds. The neural tube fuses in a central to caudal and central to cranial direction, creating the neural canal. The small holes remaining at each end of the neural tube fuse at days 25 and 27 days concomitantly with the development of the vascular supply to the neural tube. The ventricular system and central canal of the CNS develops from the neural canal, with the walls of the neural tube forming the brain and spinal cord.
Development of the Spinal Cord
The spinal cord develops from the caudal end of the neural tube, with the neural canal becoming the central canal at about ten weeks’ gestation. The cells of the neural tube differentiate into two different layers; the ependymal layer which becomes neurons, astrocytes, and oligodendrocytes, and the marginal layer which becomes the white matter as axons grow into this area from spinal cord neurons. The sulcus limitans is a longitudinal groove which forms on each side of the fetal spinal cord. This groove forms a separation between the dorsal or afferent portion of the spinal cord from the ventral or efferent portion of the spinal cord. The mesenchymal cells surrounding the protospinal cord differentiate into the dura mater, pia mater, and arachnoid mater. These meninges become fluid filled as cerebrospinal fluid (CSF) is produced during the fifth week of development, which forms the subarachnoid space.
As the spinal cord is developing, the cranial portion of the neural tube undergoes rapid growth and turning, with the neural canal forming the cranial ventricular canal. It is believed by many authors that normal development of the ventricular canal system is dependent of the caudal closure of the neural tube, which causes an increase in intraluminal pressure which stimulates brain growth and ventricular enlargement. This is one possible explanation for the finding that infants with open neural tube defects of the spinal cord, such as meningomyeloceles, very often have associated abnormalities of their brain and ventricular system.
Physiology
Intracranial Contents
After infancy the cranial vault should be considered a closed space that is normally occupied by three components: the brain and interstitial fluid comprising 80 percent of the volume; the CSF comprising 10 percent of the volume; and the blood comprising 10 percent of the total volume. The Monro-Kellie hypothesis, which states that the sum of all intracranial components are constant, necessitates that an increase in the volume of one component demands a decrease in volume of one or two of the remaining components in children in whom the sutures and fontanelles are closed. Infants with their unfused sutures and open fontanelles can tolerate slow increases in intracranial volumes [1]. Thus a child with an increase in head circumference should be evaluated for a space-occupying brain lesion or hydrocephalus even if there are no signs of increased intracranial pressure. Rapid increases in the volume of CSF or growth of a space-occupying lesion such as a brain tumor even in a young infant will overwhelm the infant’s ability to increase the size of his cranial volume, leading to brain herniation as a result of acute elevations of intracranial pressure (ICP).
Intracranial Pressure
The normal ICP in children is <15 mmHg and < 6mmHg in infants. The normal ICP for premature infants is not known. In infants with open fontanelles, the ICP may remain normal even in the setting of significant intracranial pathology. Careful physical examination should always include an examination of the fontanelles. Full or bulging fontanelles can be a sign of intracranial disease. Often the first sign of intracranial disease in an infant is a normal steady state ICP with occasional ICP pressure waves. These pressure waves should never be considered normal.
Other infants will manifest an elevation of ICP despite open fontanelles. These elevations of ICP can cause either cerebral ischemia and/or brain herniation leading to secondary brain injury. Elevations of the ICP without concomitant elevations of mean arterial pressure (MAP) will result in a decrease in cerebral perfusion pressure (CPP), which can result in cerebral ischemia.
With a decrease in CPP, cerebral blood flow (CBF) decreases. This results in decreased delivery of metabolic substrates and oxygen. Cell damage then occurs, which leads to cell death and an increase in inflammatory mediators, a cascade causing further increases in extracellular water and exacerbating the elevation in ICP. As CPP falls, neuronal dysfunction and ultimately cell death occur [1].
In addition to decreasing cerebral perfusion pressure, elevations of ICP can lead to brain herniation. This occurs when intracranial contents herniate from one compartment into another, leading to further brain ischemia and injury. Transtentorial herniation occurs when the temporal lobe displaces into the infratentorial space. Infants will show signs of hemiparesis, pupillary dilation, and eventual loss of consciousness. This is a medical emergency that will lead to death if the condition is not expeditiously relieved. The clinical signs of elevated ICP are less reliable in infants and children. Infants may exhibit signs of papilledema, hypertension, and bradycardia, but have normal ICP. Or infants with elevated ICP may show no clinical signs at all [2,3]. Classically, young children with chronically elevated ICP will have headaches, irritability, decreased oral intake, and morning emesis without papilledema [4]. Late signs include alterations in the level of consciousness, with children exhibiting abnormal responses to painful stimuli [2]. Depending on the level of obtundation, general anesthesia or sedation may be needed to evaluate these patients. Imaging studies are most commonly done, such as cranial X-rays, computed tomography (CT), and magnetic resonance imaging (MRI). These studies often reveal a decrease or obliteration of the lateral ventricles, hydrocephalus, midline shift, and/or obliteration of the third and fourth ventricles.
Cerebrospinal Fluid
Cerebrospinal fluid is produced by the choroid plexus at the rate of 0.35 ml per minute in both infants and adults [5,6], resulting in approximately 500 ml produced per day in adults [5]. However, the total volume of CSF in the subarachnoid space in adults is only 100–150 ml, meaning that the CSF is replaced several times during the day. The overall volume of CSF in infants and children is much less than in adults because the subarachnoid space is much smaller. Cerebrospinal fluid production is only marginally affected by elevated ICP.
Arachnoid villi within the subarachnoid space absorb the CSF, which enters the venous system via one-way valves between the subarachnoid space and the sagittal sinus. There is also a limited amount of reabsorption that occurs through the ependymal lining of the ventricles. The rate of reabsorption increases with elevation of ICP. Obstruction of the arachnoid villi or obstruction within the ventricular system can lead to decreased absorption. Pathologic processes such as inflammation, infection, intracranial hemorrhage, congenital malformations, and CSF absorption and ICP elevations [2].
Intracranial Compliance
It is important to estimate the amount of intracranial compliance that an infant or child has before administering a general anesthetic. Intracranial compliance is defined as the ratio of change of ICP over change of intracranial volume. In infants who have greater abilities to compensate for increases in cranial volumes because of their open fontanelles, it is difficult to assess the degree of additional compensation available if needed for general anesthesia. Once the ICP is elevated, it is reasonable to assume that compensatory mechanisms have been overridden. But infants with normal ICP may be at high risk for brain injury with even the small increases in cranial volumes/CBF that may occur with certain anesthetic techniques. Assessment of the fontanelles to determine whether the fontanelle is full in infants suspected of having intracranial pathology is essential before administering a general anesthetic. Although infants have higher compliance than adults because of their sutures and open fontanelles, children have lower compliance than adults. This is thought to be due to a higher ratio of brain water content, less CSF volume, and a higher ratio of brain content to intracranial capacity [1]. Infants with fused cranial sutures are at higher risk than normal infants for developing ICP elevations.
Cerebral Blood Volume and Cerebral Blood Flow
The cranial compartment most easily manipulated by anesthesiologists is the cerebral blood volume (CBV), though this compartment only represents 10 percent of the total volume of the cranial contents. Most of the CBF by volume is contained in the low-pressure, high-capacitance venous system. Initially increases in intracranial volume are met with decreases in CBV. Often, venous blood shifts from intracranial to extracranial, as seen in infants with hydrocephalus who develop distended scalp veins [7].
In a normal adult, the CBF is approximately 55 ml per 100 g of brain tissue per minute, which represents approximately 15 percent of the adult cardiac output [8–10]. The CBF is almost double in children, at approximately 100 ml per 100 g of brain tissue per minute, which represents about 25 percent of cardiac output [11,12]. Infants and young children in general have a larger portion of their cardiac output devoted to cerebral perfusion than do adults. Neonates and premature infants appear to have a lower CBF at about 40 ml per 100 g per minute than any other age group. The reasons for this are unclear. There is also greater variability of CBF in neonates and premature infants depending on the physical state of the infant. Cerebral blood flow is decreased during sleep and feeding states [12,13].
Cerebral blood flow in the nonanesthetized state is usually very closely coupled with cerebral metabolic rate for oxygen (CMRO2), which is known as cerebral autoregulation. In adults, CMRO2 is approximately 3.5–4.5 ml O2 per 100 g per minute; in children it is higher, but in premature infants it is lower [11]. Most general anesthetics will depress the CMRO2 by as much as 50 percent, but in very young premature infants these same general anesthetics can be excitotoxic, leading to an increase in CMRO2 [14]. Inadequate CBF will lead to conditions that result in acidosis, such as hypoxemia, hypercarbia, and ischemia. These conditions increase the hydrogen ion concentration within the cerebral blood vessels, which will cause cerebral dilation and thereby increase CBF and CBV. When there is a loss of coupling between CMRO2 and CBF, cerebral autoregulation is impaired. CBF then becomes pressure passive, meaning it varies with the system MAP.
Cerebrovascular Autoregulation
Effects of Blood Pressure
Cerebrovascular autoregulation refers to the ability of the brain to have a relatively constant CBF within a MAP range. This range is believed to be between 50 and 150 mmHg in older children and adults. In infants the lower limit of autoregulation is unknown, but studies have shown that there is a decrease in CBF when the MAP in infants less than six months of age is less than 40 mmHg. Cerebral autoregulation can be diminished or abolished by a variety of physiologic disturbances, including extreme prematurity, acidosis, sepsis, cerebral edema, tumors, various medications, and vascular anomalies.
Although the absolute lower limits of autoregulation are undefined in premature and term infants, and are likely to vary within that population, most neonatal intensive care units try to maintain the MAPs in mmHg of their patients as equal to or higher than the gestational age in weeks of the infant. It should be assumed that in critically ill infants there is a loss of cerebral autoregulation and systemic blood pressures should be maintained to ensure adequate cerebral perfusion [15].
Neonates are particularly susceptible to hypoxic events when MAP is too low. Further, these patients are particularly sensitive to drugs that depress myocardial function. The end result is that without meticulous management of blood pressure and ventilation to normocapnia, neonates and infants can suffer significant neurologic injury [16]. We have been conditioned to worry about the neurocognitive effects of anesthetic agents by the relatively recent myriad of literature. Anesthesiologists must first ensure that adequate physiologic parameters are maintained in order to achieve the best possible outcomes in neonates and small children.
Effects of Oxygen
Oxygen tension also affects CBF at the extremes, with CBF remaining constant over a wide range of oxygen tensions. In adults, when the partial pressure is less than 50 mmHg, CBF begins to increase to the point that at a PaO2 of 15 mmHg the CBF is four times normal in an effort to maintain O2 delivery [17]. This extreme increase in CBF will result in an increase in CBV, which in turn will result in an increase in ICP if the intracranial compliance is low. Hyperoxia conversely will lead to a decrease in CBF. Adults breathing 100 percent O2 will have a 10 percent decrease in CBF while neonates breathing 100 percent O2 will have up to a 33 percent decrease in CBF [9,10,18]. Hyperoxia has many adverse effects on neonates, especially premature neonates, so it is essential to balance adequate oxygen delivery with potential oxygen toxicity.
Effects of Carbon Dioxide
Carbon dioxide tension and CBF are related in a linear fashion. In adults, a 1 mmHg increase in PaCO2 increases CBF by approximately 2 ml per 100 g per minute [19]. Medically induced hyperventilation will lead to a decrease in CBF and CBV and thus ultimately decrease ICP for a short period of time, and is the basis of treating elevated ICP patients with hyperventilation. Elevations in PaCO2 will increase CBF. There is growing evidence that medically induced hyperventilation is on balance detrimental to most patients, including infants with brain injury, and is generally reserved to acutely treat high spikes of ICP. Cerebral ischemia can be exacerbated in brain-injured children who are treated with moderate hyperventilation for elevations of ICP [20].
Perioperative Management
Preoperative Evaluation
It is important to realize that the perioperative period is extremely stressful to both child and family. Parents have concerns about both the immediate risks of general anesthesia as well as the long-term consequences of their infant undergoing a prolonged general anesthetic for a neurosurgical procedure. These issues should be addressed during the preoperative interview in a reassuring manner. A very careful history and review of systems with an emphasis on the neurologic system is essential to obtain from the parents. In addition, it is very important to obtain a history of any coexisting diseases such as pulmonary and cardiac anomalies, prematurity, or evidence of difficult airway issues. Physical examination should focus on the airway, cardiac, pulmonary, and neurologic systems. A thorough history and review of symptoms with particular emphasis on neurological symptoms should be obtained from the parent. A focused physical examination with particular emphasis to the neurological system should be done.
Emergent neurosurgical procedures in infants pose special challenges. In most cases, the infants should be considered as a “full stomach” and airway precautions should be taken to prevent pulmonary aspiration. There may not be resources available to do a complete preoperative evaluation in neonates suspected of having congenital cardiac disease. A transthoracic echocardiogram along with pre- and postductal oxygen saturation on both room air and 100 percent oxygen can discriminate between cardiac and pulmonary disease.
For elective procedures, infants and neonates are fasted before general anesthesia in order to decrease the risk of pulmonary aspiration. It is important to minimize fasting time to decrease the risk of developing dehydration and hypoglycemia. For routine procedures, fasting times for clear liquids should be no more than two hours and for young infants intraoperative glucose monitoring should occur if the infants are not receiving intravenous glucose solutions intraoperatively.
As part of the preoperative evaluation, the anesthesiologist providing care for the infant should evaluate all neuroimaging studies that have been done on the infant in order to assess the type of lesion, location, the presence of intracranial hypertension, the vascular supply, and the risk of perioperative herniation. Most major neurosurgical procedures in infants are associated with intraoperative blood loss. Accordingly, it is necessary to type and crossmatch blood for intraoperative use. It is also important for the anesthesiologist to confer with the surgeon about special neurophysiologic monitoring that may be done intraoperatively in order to plan the optimal general anesthetic.
Vascular Access and Monitoring
Obtaining adequate intravenous access before incision is essential to conducting a safe general neurosurgical anesthetic. Once the procedure has commenced it is very difficult to place intravenous lines. Two relatively large-bore intravenous lines (larger than 22 gauge) are usually adequate for most procedures. Central venous access can be obtained if peripheral access is difficult. In general, femoral venous access is preferable to internal or external jugular venous access because they are technically easier to place and there is no concern about obstructing cerebral venous drainage. A single lumen central line is preferable to a multilumen central line if rapid transfusion of fluid and blood products is anticipated.
Placement of an intra-arterial catheter for blood sampling and monitoring is also very helpful for procedures in which significant blood loss is anticipated. The most commonly accessed vessel is the radial artery, but dorsalis pedis and posterior tibialis can also be easily accessed. It is important to zero the transducer at the level of the infant’s head in order to get an estimate of the cerebral perfusion pressure (CPP).
Infants undergoing craniotomies, especially in the head-up position, are at increased risk of venous air embolism so precordial Doppler is recommended in addition to standard ASA monitors. During some procedures, electroencephalographic (EEG) and neurophysiologic monitoring are used. Consultation with both the surgeon and neurophysiologist is necessary to coordinate the general anesthetic care.
Keeping the infant warm during neurosurgical procedures can be difficult. Because the head of a neonate is large in comparison with the rest of the body and a larger percentage of cardiac output is directed to the head and upper body, the neonate is at particular risk for significant heat loss during surgery. It is important to use warming lights and forced-air warming blankets, and to keep the operating room temperature high to prevent heat loss. Monitoring core temperature either esophageally or rectally is necessary. Intraoperative hyperthermia should also be scrupulously avoided.
Induction of Anesthesia and Airway Management
The most commonly used inhalational agent for children who are neurologically stable and lack intravenous access is sevoflurane. It lends itself to a rapid and smooth induction without significant myocardial depression and cardiovascular instability. Because inhalational inductions can be accompanied by respiratory depression leading to hypercarbia that could worsen elevated ICP, the anesthesiologist should be prepared to assist in ventilation relatively early during induction. Infants that are cardiovascularly unstable or who have already been accessed intravenously should have an intravenous induction with a rapid-acting hypnotic agent such as etomidate for unstable infants and propofol for stable infants. Although ketamine is a useful medication for infants with cardiovascular instability who are not neurologically compromised, it is not ideal for neurosurgical procedures. It will cause an increase in cerebral metabolism, CBF, and ICP, so it should be used cautiously in this patient population.
For emergency procedures in which the infant is felt to be at risk for pulmonary aspiration, a rapid sequence technique using succinylcholine or a large dose of a nondepolarizing medication such as rocuronium can be used. Care must be taken to ensure there are no contraindications to the use of succinylcholine, such as malignant hyperthermia, myopathies, or denervation diseases.
Elevations of ICP can occur with laryngeal intubation, so it is important to make sure that the anesthetic depth is appropriate before airway manipulation. In infants, a nasal intubation is often more easily secured and less likely to be inadvertently displaced during the surgery, especially when the infant is placed in the prone position.
Maintenance of Anesthesia
Positioning
Positioning with head pins such as the Mayfield clamp is not possible in infants because of the thinness of the infant skull. Padded headholders such as the horseshoe are most commonly used, but are not as stable as head pins. Even in a neutral position, infants are at risk for venous air embolus (VAE) because of the size of their cranium. A precordial Doppler device placed on the right sternal border of the heart will monitor for VAE. A distinctive windmill murmur will be appreciated when there is a VAE, which should prompt the surgeon to flood the field with irrigation fluid, and seal any obvious sites for air entry. The anesthesiologist should also administer a fluid bolus even if there is no evidence of cardiovascular instability. Meticulous care should be taken in positioning young infants to prevent peripheral nerve injuries and skin breakdown during prolonged procedures.
Effect of Anesthesia on the Developing Brain
Most general anesthetics have been implicated in animal models in causing long term neurocognitive deficits. In particular, prolonged exposure to agents that are gamma amino butyric acid (GABA) receptor antagonists and those that are NMDA receptor agonists has caused neuroapoptosis and long-term deficits in monkeys, rats, and mice. Although this particular issue is one of the most important in contemporary pediatric anesthesia, a thorough discussion is beyond the scope of this chapter and will be addressed elsewhere in this text.
Blood and Fluid Management
Neurosurgical cases are difficult to manage in young children, in part because fluid management is critical. Over-hydration is very possible in young children with excellent intravenous access, which can lead to excessive cerebral edema. Procedures on the cranium tend to be accompanied by intraoperative hemorrhage that is very difficult to estimate in young children by examination of the suction canisters and drapes. Frequent measured arterial blood gases and hematocrits are an important method to estimate intravascular fluid volume and red blood cell mass. Antifibrinolytics such as tranexamic acid can be administered to ameliorate blood loss in certain patient populations such as children undergoing major orthopedic and craniofacial procedures [21,22].
It should be assumed that the blood–brain barrier is disrupted during neurosurgical procedures. So the choice of intravenous fluids may affect cerebral perfusion, cerebral edema, water and sodium homeostasis, and serum glucose concentration. It is not known whether osmotic or oncotic pressure gradients are more important in preventing cerebral edema, but most investigators lean toward using crystalloid solutions rather than colloid solutions during neurosurgery. Since the goal is to provide a solution that is hypertonic (or at least not hypotonic) to plasma, lactated Ringer’s solution, which has an osmolality of 273 mOsm L–1 (normal serum osmolality being 285–290 mOsm L–1) is not ideal for neurosurgery. Normal saline, which is slightly hypertonic with an osmolality of 308 mOsm L–1, is a better choice for neurosurgical procedures, even though it can lead to a hyperchloremic acidosis if large volumes are infused [23]. Osmotic and loop diuretics can be used to transiently induce dehydration when surgical exposure is difficult or there is a dangerous elevation of ICP. Care must be taken to avoid systemic hypotension due to peripheral vasodilation caused by diuretics, which can diminish cerebral perfusion pressure. Very young patients can benefit from slow glucose administration during neurosurgical procedures. In patients with altered glucose metabolism, such as those treated with hyperalimentation, who are diabetics, or poorly nourished, the addition of glucose intravenously may also be necessary. However, hyperglycemia during procedures has been associated with increasing cerebral infarct size during cerebral ischemia, so avoiding both hyper- and hypoglycemia is important [24].
Specific Lesions and Situations
Hydrocephalus
Hydrocephalus, affecting about 2 in 1000 live births, is a condition in which there is excessive accumulation of CSF around or within the brain. It can be caused by too much CSF production, too little CSF resorption, or a blockage within the cerebral ventricular system that causes a build-up of fluid. Communicating hydrocephalus occurs when the blockage of CSF flow is downstream of the ventricles. Noncommunicating hydrocephalus or obstructive hydrocephalus occurs when the flow of CSF is blocked along one of the passages connecting the ventricles. During infancy, the most common symptoms of hydrocephalus are a rapid increase in head circumference, irritability, sleepiness, vomiting, and downward deviation of the eyes (sunsetting). It is treated by shunting from the ventricles or spinal cord to the abdominal cavity or atria. There is a one-way valve within the shunt that prevents CSF from flowing back into the ventricles. Some infants with hydrocephalus can be treated neuroendoscopically with the creation of a ventriculostomy on the floor of the third ventricle. Complications of ventricular shunts include failures, infections, and disconnections.
Epilepsy
Surgical treatment for epilepsy is being done more commonly in infants. Lesions associated with epilepsy include focal malformations of cortical development, tumors, Sturge–Weber syndrome, epidermal nevus syndrome, and vascular malformations and infarctions. Surgical treatment of focal lesions has a success rate of infants being seizure-free of approximately 60 percent. Infants operated on at a young age and for infantile spasms had the most improvement of developmental quotients [25].
Myelomeningocele
Myelomeningocele occurs when the neural tube fails to fold normally in the third to fourth week of gestation. It occurs in about 1 in 1000 live births in the United States. The infant is at increased risk for infection, so generally closure of the meningomyelocele occurs on the first day of life. It can be associated with other congenital anomalies so a thorough preoperative evaluation is warranted. About 20 percent of cases at birth are associated with hydrocephalus, but eventually 80 percent of patients with meningomyelocele will require ventricular shunting for hydrocephalus. The lesion must be protected prior to closure with sterile warm, wet dressings. Intubation can be accomplished in the lateral position or with the infant supine on foam cushions that shield the meningomyelocele from any pressure. Postoperatively, infants must be in the prone position.
References
Choanal Atresia and Stenosis
Choanal atresia and stenosis are congenital defects resulting in abnormal narrowing or blockage of the nasal passages(s), typically by excess bone (90 percent) or membranous tissue (10 percent) which does not recanalize during fetal development. Unilateral stenosis/atresia may be asymptomatic, remaining undiagnosed until later in life, or masquerade as chronic sinusitis. However, bilateral stenosis is a serious problem for the obligate nasal breathing neonate that results in respiratory distress marked by severe chest wall retractions during inspiration at rest and relief of the obstruction when the infant cries [1]. In addition, the infant is also unable to breathe and nurse simultaneously. Insertion of an oral airway or endotracheal intubation may be needed to treat severe obstruction until a surgical consult can be done [2,3]. The diagnosis is suspected after failure to pass a feeding nasogastric tube through either nare. Radiographic confirmation is accomplished by a computerized tomography (CT) scan. The incidence of this defect is 1:7000 births. The ratio of male:female as well as bilateral:unilateral is 1:2. Patients with CHARGE syndrome account for 50 percent of the bilateral cases.
Numerous methods of surgical correction have been described using trans-palatal, trans-septal, trans-antral, sublabial trans-nasal, and trans-nasal approaches [4–6]. Traditional surgical correction involves puncturing the occluding tissue followed by dilating the opening and stenting it to prevent closure. The stent is removed after 5–12 weeks, with longer duration resulting in better results [7]. An alternative method to avoid using a stent is to administer oral steroids for five days followed by a month of intranasal steroids [6]. Newer methods of correction utilize a trans-nasal endoscopic approach to remove the membranous or bony tissue with or without the use of a helium:YAG laser. Anesthetic considerations start with evaluation of associated defects, including the possibility of congenital cardiac disease. General orotracheal anesthesia is performed with required safety considerations if a laser is used. Hemodynamic parameters should be closely monitored as the use of cocaine or other vasoconstrictive agents to decrease bleeding and aid visualization may cause hypertension and/or arrhythmias. Operative complications include cerebrospinal fluid (CSF) leak if excess bone is removed, respiratory distress should the stent become occluded, and re-stenosis.
Laryngeal and Tracheal Issues
Tracheal Stenosis
Tracheal stenosis may be congenital, iatrogenic, or the result of a disease process [1]. It may be classified as being general hypoplasia, funnel-shaped, or segmental [2]. Complete tracheal atresia is a very rare entity that is incompatible with life and is mentioned for completeness. Other congenital problems include complete tracheal rings involving one to all rings and a complete cartilaginous sleeve. The abnormal tracheal structure causes problems with airflow and interferes with clearance of secretions, causing stridor, retractions, and wheezing which may be associated with repeat episodes of pneumonia. Treatment of short segments is by surgical excision and re-anastomosis. Longer segments require more extensive procedures or possibly tracheal autograft. Innovative solutions using 3D printing and biologic materials, e.g., stem cells, to replace the defective trachea are starting to be reported.
Iatrogenic causes of tracheal stenosis are almost exclusively caused by prolonged intubation. Mucosal injury may be caused by high transmural pressure of the inflated cuff of an endotracheal tube, which interferes with perfusion of the mucosa. Mucosal damage is compounded by up-and-down movement of the endotracheal tube, leading to scarring and circumferential contraction. The damaged segment can be small or extensive. Small segments can be treated by dilation, while large segments require excision or posterior cartilage graft to keep the segment from re-stricturing.
Tracheomalacia/Bronchomalacia
Tracheomalacia describes the collapse of the airway when tracheal structures are unable to keep the airway patent in response to transmural airway pressures during respiration. Primary tracheomalacia is caused by an abnormal ratio of the cartilaginous to the membranous section of a tracheal ring. During expiration the posterior membranous section collapses into the lumen of trachea causing a variable degree of obstruction. Primary malacia is often seen in premature and newborn infants and usually resolves by two years of age. Severe cases, however, may require prolonged CPAP, intubation, or tracheostomy for treatment.
Secondary tracheal malacia may result from congenital abnormal vasculature, which may compress the trachea at various locations. Other etiologies include compression by an enlarged heart secondary to cardiac defects, bronchopulmonary dysplasia, or a tracheoesophageal fistula. Abnormal development of the major vessels may present as a double aortic arch, pulmonary vascular sling, aberrant right subclavian artery, and right-sided aortic arch [8]. The unifying feature of these conditions is that the trachea is compressed between two vascular structures that cause an increased ratio of the cartilaginous to membranous section of the compressed tracheal rings. Congenital cardiac lesions usually involve the left main stem bronchus, which is normally located between the left pulmonary artery and the left atrium, along with the left pulmonary veins. Cardiac lesions that lead to increased pulmonary pressure and/or left atrial enlargement can encroach on the left main stem bronchus causing malacia. Eighty-six percent of tracheal esophageal fistulas are type C, which consists of proximal esophageal atresia with a distal esophageal fistula to the trachea. The triad of widened cartilages, ballooning of the posterior wall, and collapse of the distal tracheal lumen in one or both bronchi is seen on endoscopy.
The severity of respiratory symptoms associated with tracheomalacia varies. Patients will present with stridor, dysphagia, wheezing, chronic cough, inability to handle secretions, recurrent pneumonias, atelectasis, and cyanotic apneic spells. An inability to wean from mechanical ventilation or the failure of tracheal extubation or tracheostomy decannulation may indicate underlying tracheomalacia. Diagnostic studies include chest radiograph, esophagram, chest CT with dynamic studies, bronchoscopy, and possibly angiography. Treatment may require long-term ventilation and/or surgical correction. Surgical procedures include splinting, stenting, aortopexy, resection, and correction of the underlying problem.
Bronchogenic Cysts
Bronchogenic cysts are the result of abnormal budding of the bronchial tree, which develops independently of normal alveolar formation and growth. They contain ciliated epithelium and pseudostratified columnar epithelium [8]. They may be connected to the bronchial airways, and for that reason spontaneous respiration during anesthesia is preferred. Anticholinergic premedication to decrease secretions and topical local anesthesia are useful adjuncts for sedation. Most often they present in infants as wheezing, cough, or respiratory distress. Airway obstruction may occur if they enlarge. Diagnosis is by radiograph or CT of the chest. Surgical excision is indicated.
Pulmonary Sequestration
Pulmonary sequestration is a collection of abnormal pulmonary tissue that is abnormally connected to the tracheobronchial tree. It may be extralobar or intralobar. The arterial blood supply originates from the systemic circulation from below the diaphragm, but venous drainage may differ. The extralobar type may have its own pleural cavity and systemic venous drainage whereas the intralobar type has no pleural membrane and venous blood drains into the pulmonary circulation. Like other pulmonary problems, it may present with infection, mass effect, and other symptoms. Plain radiographs, CT, MRI, and angiography are used for diagnosis. Treatment is surgical excision.
Congenital Cystic Adenomatoid Malformation
Congenital cystic adenomatoid malformations (CCAMs) are benign cystic lesions that appear during fetal development. They are classified as microcytic, macrocytic, or solid, but differ from bronchopulmonary sequestration by the fact that they do not have a systemic arterial supply. They are found during fetal ultrasonography. The lesions may shrink and disappear or continue to enlarge as the fetus grows. Large lesions may cause fetal distress by causing contralateral lung hypoplasia, cardiovascular collapse, maternal polyhydramnios, and fetal hydrops. For large lesions, fetal surgery, which includes thoracocentesis, thoracoamniotic shunting, or resection utilizing an ex utero intrapartum therapy (EXIT) procedure, may be life-saving. Smaller lesions may wait until after birth to be excised as these lesions may undergo malignant degeneration.
Anesthetic Considerations for Tracheal and Pulmonary Issues
The primary anesthetic consideration is to maintain adequate inspiratory and expiratory air flow through the tracheal branchial tree. Stenotic lesions limit air passage and increase the work of breathing by causing nonlaminar flow. Inhalational induction is prolonged and is best accomplished by using a slow respiratory rate with high concentration of anesthetic gas. Malacia of the tracheobronchial tree leads to collapse of the lumen as transmural pressures change during inspiration and expiration, depending on whether the segment is intrathoracic or extrathoracic. Intrathoracic segments are stented open during inspiration and collapse during expiration. Use of end-expiratory pressure, PEEP or CPAP, keeps the airway patent throughout the respiratory cycle. Thorough intraoperative monitoring and maintenance of deep anesthesia to prevent coughing, laryngospasm, or bronchospasm should be the anesthetic goals for all cases. Mass lesions cause compression of the adjacent lung parenchyma and tracheobronchial structures. Patient positioning may have a dramatic effect on the ability to ventilate the lungs and dictate surgical positioning. Likewise, hypoventilation increases collapse of the distal alveolar segments. Maintaining adequate inflation pressures is necessary to prevent the resultant hypoxia. Finally, for lesions that connect to the tracheobronchial tree, positive pressure ventilation may cause expansion of the lesion. This in turn may increase any mass effect. Spontaneous ventilation should be used in this situation until the connection is severed.
Cystic Lesions
Dermoid cysts are structures lined by epithelium which contain various elements derived from epithelium along embryonic fusion plates [9]. They range from 1 to 4 cm in size, are commonly found on the forehead, lateral eye, or neck, and can be fixed or mobile. Most are subcutaneous although there can be extension intracranially or intraorbitally. Treatment is surgical excision so preoperative CT or MR scan to rule out extension is recommended. A tunneled endoscopic approach starting behind the hairline to avoid facial scarring can be utilized.
Thyroglossal duct cysts occur along the tract by which the thyroid descends during embryonic development from the base of the tongue to the normal thyroid position. They are typically noticed as a midline neck mass following an upper respiratory infection, or become primarily infected [10]. These cysts often move when swallowing and can track though the hyoid bone [3]. Preoperative ultrasound is recommended. Treatment is excision to prevent further infection. Recurrence is more likely if the child is under two years of age or has had two or more infections [11].
Branchial cleft cysts are derived from sequestration of first and second branchial cleft elements, resulting in cysts, sinuses, and tags. The incidence is sporadic, although there are reports of autosomal inheritance with equal male to female frequency, and 10 percent are bilateral [9]. These lesions usually present as soft tissue masses along the border of the sternocleidomastoid muscle. First branchial arch cysts are usually high in the neck. The more common second arch cysts are lower in the neck and often communicate with the tonsillar fossa. The less common third arch cysts are low in the neck and can communicate with the pyriform sinus. Branchial cleft cysts usually present after an upper respiratory infection as a tender mass. After treatment of the cysts with antibiotics they are excised in order to prevent reinfection and the possibility of carcinoma [10].
Vascular Malformations
Hemangioma
Hemangiomas are the most common benign tumors in infants [12]. This abnormal collection of blood vessels is characterized by having increased activity of endothelium and mast cells during the growth phase. They can be superficial, “capillary or strawberry” or deep, “cavernous” and can occur in any organ. They may not be present at birth, but appear within a few weeks and rapidly expand for 6–12 months. It is during this growth phase that they are invasive, causing ulceration, expansion, and may involve a vital organ [12]. After the growth phase finishes, they will slowly involute over a period of years. Hemangiomas may be multicentric; so affected patients should be thoroughly evaluated for other lesions. Large segmental hemangiomas about the face may be part of PHACE syndrome (posterior fossa malformation, hemangiomas, arterial abnormalites, cardiac defects, and eye anomalies). Kasabach–Merritt syndrome is the occurrence of hemangioma, thrombocytopenia, and coagulopathy. Treatment with propranolol may slow the rate of growth. Prednisone is very effective in causing rapid involution. Superficial lesions may be treated by laser excision while surgical excision may be needed for others.
Other Vascular Malformations
Unlike hemangiomas, vascular malformations are characterized as having normal growth of the endothelium and mast cells. They grow proportionately with the rest of the body and do not involute [2]. These vascular malformations can be associated with various syndromes such as Klippel–Trenaunay–Weber, Struge–Weber, Maffucci, and Parkes–Weber. All vascular malformations should be thoroughly evaluated using MR/CT scan, Doppler ultrasonography, and contrast radiography to delineate the type and extent of the lesion.
Venous Malformations
Venous malformations are the most common vascular malformations. They present as soft, compressible, blue-colored structures. They are found predominantly about the head and neck. A high percentage of larger lesions have associated deep cerebral malformations that should be investigated. Treatment with sclerotherapy is highly successful, especially when surgical excision would be disfiguring or impossible.
Arteriovenous Malformations
Arteriovenous malformations (AVMs) are the most serious vascular lesions. They can cause high-output heart failure or may spontaneously rupture. Dental extraction in the presence of a mandibular AVM has been known to lead to massive bleeding. Treatment of an AVM is sclerotherapy, quickly followed by surgical excision to prevent new arterial channels from opening.
Lymphatic Malformations
Lymphatic malformations are the result of primary lymph sacs that do not coalesce with the rest of the lymphatic system. The resultant cystic structures may be small, microcystic, or large, macrocystic, also termed cystic hygroma. Microcystic lymphangioma are invasive and ill-defined, extending through various tissues and organs. Macrocystic tend to be well circumscribed, although both elements can coexist. Lymphatic malformations present early in life and expand as lymph accumulates within the cysts. Cystic hygromas occur in about 1:12 000 live births, and are located in the posterior triangle of the neck, cephalad to the clavicle [3]. The cyst may extend from the skin internally to the mucosa of the trachea. Lesions can be life-threatening if the trachea becomes compressed [11]. If detected in utero by ultrasonography, the airway may be secured intrapartum while the umbilical vessels are still attached, via an EXIT procedure. Surgical excision is necessary for treatment in most cases. A chest radiograph, ultrasonography, and MR/CT scan are needed to delineate the extent of the lesion. Sclerotherapy may be effective, although for macrocystic lesions a new sclerosing agent, OK432, has been very effective.
Anesthetic Considerations for Vascular Malformations
Airway management and blood loss are the two major considerations. As a large percentage of all vascular malformations occur in the head and neck region, problems arise resulting in distortion of facial structures and causing difficulty with mask ventilation, tracheal intubation, and ventilation/respiratory mechanics. Venous and lymphatic malformations can become disfiguring and grow to large size. They may interfere with the fit of the facemask, making inhalational induction of anesthesia difficult or impossible. Alternatively, when located within the neck or thorax, compression and deviation of the airway is common. Fortunately, typically these malformations are compressible, allowing for instrumentation and the passage of an endotracheal tube. However, they can swell and become non-compressible in response to surgical manipulation, sclerotherapy, or infection. Tracheal extubation should be delayed until airway swelling has subsided, which may require several days after surgery. Hemangiomas and arteriovenous malformations may also present in, or around, the airway. Hemangiomas are friable and thus bleed easily. Endotracheal lesions may ooze if traumatized by an endotracheal tube. Arteriovenous malformations may also bleed if manipulated. The bleeding, however, will be brisk, heavy, and potentially life-threatening. Airway management should be directed to avoid manipulating or touching the malformation.
Blood loss during excision of large hemangiomas can be misleading in the small infant or child. Bleeding occurs at a steady slow rate and is controlled by direct pressure. For the small infant near the physiologic hematocrit nadir, the transfusion threshold may be reached quickly. Mucosal paleness or tachycardia may be signs that a repeat hematocrit is needed for determination if red cell transfusion is warranted. Large venous or mixed lesions may result in large blood volume loss, especially since tourniquets cannot be used in head and neck procedures. Large-bore venous access and possibly an arterial line are needed. Arteriovenous malformations always present the possibility of massive blood loss. Preoperative embolization decreases the risk of bleeding, but an arterial line is mandatory for anything but the smallest lesions.
Craniofacial Problems
Structural abnormalities of the head and neck comprise a diverse set of congenital problems that may be grouped in numerous ways. Syndromes resulting from abnormal ossification of bony structures include Crouzon’s (craniofacial dysotosis), Apert (acrocephalosyndactyly), and Treacher Collins (mandibulofacial dysotosis) syndromes. A defining feature of Crouzon’s and Apert syndromes is the premature closure of cranial sutures and that of the skull base, resulting in midface hypoplasia. The small nares and choanal stenosis cause these patients to be nearly obligate mouth breathers with a high incidence of sleep apnea. Those with Treacher Collins syndrome have midface abnormalities caused by nonfusion and hyoplasic development of the maxilla, mandible, and auditory structures. These patients have a high incidence of glossoptosis with associated Robin sequence.
Other abnormalities are the result of lack of development of bone or soft tissue. These include oculoauriculovertebral syndrome (hemifacial microsomia, Goldenhar’s syndrome), hemifacial atrophy (Romberg syndrome), and congenital facial diplegia (Moebius syndrome). In Goldenhar’s syndrome, the first and second brachial arch structures of the ear and mandible are affected to various degrees. Loss of tissue results in distortion of the face and airway, which worsens with unbalanced growth. Romberg syndrome patients are normal at birth, with progressive atrophy and distortion of the facial features with growth. The congenital palsies of the cranial nerves of Moebius syndrome result in difficulties with feeding, speech, and expression. The mouth opening is usually small.
Anesthetic Considerations
Airway management is the major anesthetic concern of these patients as they typically are difficult to bag-mask ventilate, difficult to intubate, or both. Patients with midface hypoplasia have high resistance to nasal breathing and are essentially mouth breathers. Standard airway maneuvers, which close the mouth to get a better mask fit, increase the degree of obstruction. Care should be taken to keep the mouth open to allow for air passage. Early insertion of an oral airway is helpful. Topical anesthesia applied to the oral cavity prior to induction anesthesia will minimize the risk of laryngospasm from the early insertion of the oral airway.
Other patients who have mandibular hypoplasia or associated Robin sequence are at high risk of airway obstruction and difficult tracheal intubation. Alternative methods other than direct laryngoscopy to secure the airway include insertion of a laryngeal mask airway (LMA), fiberoptic instrumentation, and surgical instrumentation. Also see ‘Robin sequence’ below.
Cleft Lip and Palate
Cleft lip and palate are the most common congenital anomalies, occurring in 1 in 500 live births [13]. They may occur singly, together, as part of a syndrome, or the result of Robin sequence. The exact causative genetic factors are unknown but there is a strong familial inheritance. The problems associated with cleft lip/palate include difficulties with breathing, feeding, speaking, hearing, and general psychological well-being. The repair of the cleft lip may be staged or done as a primary repair. A dentomaxillary appliance may be inserted prior to repair to align the discontinuous alveolar ridges of the palate. Correction of the cleft palate should be performed before the child starts speaking. Some surgeons will use the Rose positon, resting the patient’s head in their lap, for the palate repair. Similarly, some will use a balsam of Peru-soaked pack sewn on the palate repair to promote wound healing. This dressing effectively obstructs the oral airway and thus patients must breathe nasally postoperatively.
Robin sequence
Robin sequence is defined by the three findings of micrognathia, glossoptosis, and airway obstruction. It was Pierre Robin who first identified the airway obstruction as being the result of glossoptosis as the posteriorly displaced tongue contacts the pharyngeal wall. Cleft palate occurs in 90 percent of Robin sequence patients as the displaced tongue mechanically interferes with closure of the palatal plates. Both the airway obstruction and the cleft palate are the result of micrognathia. Robin sequence may occur by itself or be associated with any syndrome that includes micrognathia.
These patients may have failure to thrive as the result of chronic hypoxia, increased work of breathing, and poor feeding. Treatment is directed at relieving the airway obstruction. Nonsurgical modalities include using the prone position with or without a nasopharygeal airway. Surgical therapies include tongue–lip adhesion, mandibular distraction, and tracheostomy. Additional alimentation may be provided via a feeding tube or a gastrostomy tube. If the Robin sequence is not associated with a syndrome, the mandible eventually will grow to near normal size, alleviating the airway obstruction. However, if associated with a syndrome, the mandible may remain hypoplastic.
Anesthetic Considerations
Patients with cleft lip or palate usually do not have airway management problems unless they are associated with other facial abnormalities. Airway obstruction may occur during inhalational induction if the tongue falls posteriorly into the cleft palate. Obstruction is relieved by insertion of an oral airway. Tracheal intubation is likewise without problem unless a bilateral cleft exists. The midline prolabial segment is angulated forward and upward, interfering with insertion of the laryngoscope and alignment of the axis of larynx visualization. Oral RAE endotracheal tubes are preferred as they don’t distort the facial symmetry around the upper lip. When properly inserted, the distance from the bend in the tube to the tip is a fixed distance. Flexion of the neck may move the tip into a mainstem bronchus. Secondarily, RAE tubes are not easily suctioned and are easily clogged.
The safe induction of anesthesia and tracheal intubation for Robin sequence patients is always a problem. General anesthesia relaxes the oropharyngeal musculature, augmenting the airway obstruction. Techniques that bypass the obstruction allow safe inhalational anesthesia. These include awake insertion of an LMA or insufflation of anesthesia gas after insertion of a nasal pharyngeal airway/endotracheal tube within the nasopharynx. The second step is to safely secure the airway with an endotracheal tube. Options include intubating through the LMA, use of a glidescope once the patient is anesthetized, or using a fiberoptic bronchoscope for nasal/oral intubation with the patient breathing spontaneously.
Myringotomy and Tympanostomy Tubes
Otitis media, commonly called an “ear infection,” is a very common diagnosis and is the most common indication for pediatric outpatient antimicrobial therapy in the United States [14]. Recurrent otitis media may result in fibrosis and scarring of the middle ear, formation of a cholesteatoma, and conductive hearing loss. The treatment of recurrent otitis media is drainage via a myringotomy, a small incision in the tympanic membrane, followed by the insertion of a small tube to facilitate drainage. The tubes are naturally extruded in 6–12 months, although retained tubes may be surgically removed. The procedure is generally less than ten minutes in duration. General anesthesia is commonly administered using an inhalation agent by mask for induction and maintenance. Intravenous (IV) access is generally not required. The postoperative pain is mild and can be addressed with acetaminophen, ketorolac, or intranasal fentanyl. As always, a careful preoperative history and physical is important to discover comorbid conditions, the most common being cleft palate.
Middle Ear Surgery
Cholesteatoma
A cholesteatoma is squamous epithelium and its accumulated debris within the middle ear and pneumatized temporal bone. It may be characterized as congenital or acquired, with or without an infection. Acquired cholesteatoma is the most common etiology as the result of middle ear disease and is seen in older children. Congenital cholesteatoma is usually diagnosed by a pediatrician and is differentiated from the acquired form by being diagnosed in infancy or early childhood, in patients with no history of prior ruptured eardrums. Treatment is surgical excision either by a tympanoplasty or can be more extensive with a mastoidectomy. A general anesthetic with a secure airway and IV access are required for the surgery.
The patient is positioned supine with the head rotated laterally for exposure of the affected side. Care should be used for patients with limited neck range of motion or those at risk for cervical instability (e.g., patients with trisomy 21). For these patients, consideration for lateral decubitus positioning may be warranted. The facial nerve is usually monitored, necessitating avoidance of long-acting neuromuscular blocking agents. Sevoflorane and/or isoflurane are generally used, but nitrous oxide should be avoided. Tympanometry has shown increased fluctuations in middle ear pressure when nitrous is added to other inhaled anesthetics [15]. Diffusion of nitrous oxide into the middle ear will increase the pressure, causing outward movement of the tympanic membrane. Once the nitrous oxide is turned off, the rapid absorption of gas will create negative pressure, resulting in backward displacement of the tympanic membrane and disrupting the surgical repair. The movement may also be significant enough to cause disarticulation of the ossicles, leading to conductive hearing loss than can persist for several weeks postoperatively. The incidence of postoperative nausea and vomiting (PONV) after tympanoplasty is greater than 50 percent in some studies, although rarely occurs in infants, and the use of nitrous oxide may be contributory. Both dexamethasone and ondansetron have been shown to be efficacious as prophylactic agents for the treatment of PONV [16] in older children. If possible, a deep extubation is desirable to facilitate a smooth wake-up without excessive coughing or head movement.
Cochlear Implants
Cochlear implants are a popular and effective treatment for children with severe to profound sensorineural hearing loss. Unlike hearing aids which simply amplify sounds, the cochlear implants transform acoustic energy into electrical energy and stimulate the remaining inner ear neurons, partially restoring the child’s hearing. The surgical procedure involves attaching an internal processor to the mastoid process and connecting the electrodes to the cochlear neurons. There has been a trend toward earlier placement, with the procedure being performed on children as young as six months of age. The procedure is best completed in tertiary care centers where experienced pediatric surgeons, anesthesiologists, and perioperative care teams can take care of these young children [17].
The procedure requires a general anesthetic with a secure airway for children, though sedation with regional techniques has been used in adults. Use of muscle relaxation for optimal surgical conditions should be discussed with the surgeon as intraoperative facial nerve monitoring may be used. After insertion into the cochlear nerve, the implant is calibrated by two electrically evoked potentials, the evoked stapedius reflex threshold (ESRT) and the evoked compound action potential (ECAP). The ESRT is the loudest stimulus than can be tolerated without pain, while the ECAP is the smallest stimulus that can be perceived as sound. Volatile anesthetics suppress the ESRT in a dose-dependent fashion, resulting in inaccurate calibration, and a stimulus which may be painful when awake. Propofol does not cause this decrement and can be safely used, making it prudent to switch to a total intravenous anesthesia (TIVA) for the calibration portion of the procedure [18]. If volatile anesthetics are used early in the procedure it is important to communicate with the surgeon about the concentration and timing of the inhaled agent in relation to the actual implantation. Alternatively, the device can be calibrated when awake postoperatively, though this is very difficult in an infant. As with tympanoplasty, the PONV rate can be quite high and prophylactic antiemetic agents should be utilized in children older than one year.
Chronic Sinusitis/Rhinosinusitis
Sinus Development in Children
At birth, neonates have pea-sized ethmoid and maxillary sinuses. During the second year of life, the sphenoid sinuses begin to develop, and after age eight the frontal sinuses develop. All reach adult size by adolescence. The paranasal sinuses are very commonly infected in young children, with about 10 percent of upper respiratory infections being complicated by paranasal sinusitis. Diagnosis is usually made in children with a cold lasting longer than 10–14 days, often with a low fever. Sinusitis symptoms are uncommon in infants and should lead to suspicion of immunologic deficiencies, congenital abnormalities, and the possibility of foreign bodies in the nasal cavity. Endoscopic sinus surgery is rarely done in infants. Occasionally adenoidectomies are done in infants to alleviate chronic sinus congestion.
Tongue Tie Ankyloglossia
The term ankyloglossia (AG), commonly called a tongue tie, is Greek for “curved tongue.” It has an incidence as high as 10 percent in some studies. The condition is somewhat controversial as not all clinicians agree on the definition or its clinical significance. Ankyloglossia is essentially a short, tight, thickened, lingual frenulum which may reduce tongue mobility, making speech articulation and breastfeeding difficult. (Figure 21.1) In infants with difficulty breastfeeding, release of ankyloglossia has been shown to improve the ability to latch onto the breast and feed effectively. It may also reduce maternal discomfort with breast feeding. Some clinicians have suggested that ankyloglossia is linked to the development of malocclusion and gingival recession, but the data are inconclusive [19].
Figure 21.1 Ankyloglossia in an adult.
The frenulum naturally recedes between six months and six years of age so observation for some years may be indicated. The timing of the procedure is therefore quite variable and is aimed to relieve symptoms. For speech issues, there are very few data to support prophylactic intervention and limited data to support intervention in those with breastfeeding issues. A simple frenotomy can be carried out very quickly with local anesthetic as the frenulum is often poorly vascularized and innervated. Laser procedures have been described as well. A tighter and more vascular frenulum may require a more extensive frenectomy or frenuloplasty, including possible division of the genioglossus muscle with a Z-plasty of the remaining tissue [20]. In an older child, this may be possible with local anesthesia, but younger children require general anesthesia
There are no literature recommendations to guide specific anesthetic management. Anesthetic technique should be discussed with the surgeon and based on the length of the procedure, the need for mouth opening, and the need to secure the airway. Short procedures may be done with inhalational mask anesthesia, just removing the mask to allow the surgeon brief access. Longer procedures may need to have the airway secured with an oral endotracheal tube or LMA. As always, a detailed history and physical should be completed, with special attention to comorbid conditions as there have been reported associations with an X-linked cleft palate condition.
Iatrogenic Problems
Tracheal Stenosis
Tracheal stenosis may be congenital in etiology, but is more commonly an unfortunate consequence of prolonged intubation in a neonate, even if the endotracheal tube is appropriately sized. The stenosis may be glottic or subglottic, with the latter being much more serious and difficult to intervene upon surgically. Flexible or rigid bronchoscopy is often performed to visualize the site of the lesion and the surrounding anatomy. In combination with CT imaging and bronchoscopy, intubation with the largest endotracheal tube (ETT) that allows an audible leak provides information regarding the diameter of the stenosed portion.
If the stenosis is mild, a simple dilation or laser tracheoplasty may be the only needed intervention. With more severe lesions, invasive reconstructive procedures are indicated. One approach is the anterior cricoid split procedure. This is essentially a cricoid incision that uses an endotracheal tube as a stent. The incision begins just inferior to the thyroid cartilage and traverses the cricoid, continuing into the second tracheal ring. Sutures are then placed through the incision, allowing it to heal over the ETT tube with a slightly larger diameter. The ETT may be left in place for 1–2 weeks while the incision heals.
A more invasive larynogotracheoplasty involves a longer midline incision of the trachea from the inferior portion of the thyroid cartilage to the third or fourth tracheal ring. Following the incision, cartilage grafts are sewn in place to increase the diameter of the lumen. A stent is sometimes needed for mechanical support as the healing takes place. Revisions, excisions of scar tissue, and further procedures are often needed after tracheal surgery.
Vocal Cord Paralysis
Vocal cord paralysis is a common diagnosis in both adults and pediatric patients. While birth trauma, postoperative nerve dysfunction, and cardiac malformations are some potential etiologies, most patients carry a diagnosis of idiopathic vocal cord paralysis. Inspiratory stridor with hoarseness or a weak abnormal cry is frequently the presenting symptom in unilateral paralysis. Bilateral paralysis results in significant airway obstruction, stridor with a normal cry, aspiration, and difficulty with phonation. Bilateral paralysis is frequently comorbid with posterior fossa lesions and Arnold–Chiari malformation as stretching of the vagus nerve over the jugular foramen leaves it open to injury and paralysis. A tracheostomy may be required for severe obstructive symptoms and aspiration until an underlying cause can be diagnosed and surgical correction performed.
When inducing anesthesia, continuous positive pressure should be gently applied, abolishing the stridor, and stenting the airway open for easy ventilation. A direct laryngoscopy often provides very little information other than seeing if the vocal cord is paramedian and immobile. A fiberoptic intubation in the appropriate candidate provides better visualization, and allows a view of the glottis as well. The paralyzed vocal cord will not narrow the airway so a normal-sized ETT can be used.
Postoperative swelling and edema can make a well-compensated infant with a vocal cord lesion more stridulous and increase the work of breathing. Care should be taken to complete an atraumatic intubation with an appropriately sized ETT. The cuff pressure should not be above 20–25 mmHg. If there is concern about airway swelling, consider leaving the infant intubated or administer steroids.
Adenoids and Tonsils
The tonsillectomy, often performed with an adenoidectomy, is one of the most common pediatric procedures in the United States, but is rarely done in children less than one year. However, there is a recent trend to consider adenotonsillectomy in infants as a primary treatment for obstructive sleep apnea [21]. The efficacy of this treatment is unclear, with several studies showing good benefit from adenotonsillectomy in infants but others showing a greater persistence of OSA after adenotonsillectomy and a higher incidence of postoperative complications despite an improved apnea hypopnea index (AHI) [22,23]. Because of the possibility of worsening OSA in the immediate postoperative period, most institutions will admit infants to the intensive care unit postoperatively.
Intraoperative Considerations
The procedure is generally quite fast, typically less than 30 minutes. A variety of surgical techniques are used, including the guillotine and snare, ultrasonic coblation, cold dissection, hot dissection, and the use of electrocautery. In small children, anesthesia is usually induced by mask, using a breathdown technique, after which IV access is established. The airway is secured with an ETT, which may be an oral RAE tube or an armored ETT. The use of an LMA has also been shown to be effective. Tracheal extubation can be accomplished when the patient is awake, either in the supine or lateral head-down position. Alternatively, deep extubation can be utilized, with care given to maintain the patient’s airway and prevent aspiration. Postoperative airway complications include laryngospasm and bleeding, which make mask ventilation and reintubation more difficult. Postoperative airway manipulation can disturb the friable tissues, contributing to postoperative hemorrhage.
The use of electrocautery increases the risk of an airway fire. Fire prevention includes keeping the FiO2 below 0.3, using only short bursts of cautery, and covering the eyes with wet gauze. The use of a special ETT (discussed below) will help reduce the risk of a fire.
Analgesia and PONV
There is a paucity of information about the optimal analgesic plans for infants after tonsillectomy. Analgesia is important to ensure a smooth wake-up without excessive crying and agitation, but narcotics should be used cautiously in infants postoperatively, especially those that have a history of obstructive sleep apnea. Small doses should be used until the infant is awake and able to breathe and maintain a patent airway. A variety of nonopioid techniques can be used in all children. A recent study of 161 children (non-infants) found that a single dose of IV ibuprofen (10 mg kg–1) given preoperatively significantly reduced postoperative fentanyl requirements with no increase in the incidence of postoperative hemorrhage [24]. A 2013 Cochrane review on the effect of nonsteroidal anti-inflammatory drugs (NSAIDs) on postoperative bleeding after pediatric tonsillectomy found a non-significant increase in the risk of bleeding requiring surgical intervention, no significant increase in the risk of bleeding requiring nonsurgical intervention, and less PONV [25]. Regional techniques have been found to be effective and opioid-sparing. A block of the auricular branch of the vagus nerve with 0.2 ml of 0.25 percent bupivacaine was as effective as 2 μg ml–1 of intranasal fentanyl, with no differences in postoperative pain score, rescue analgesics, PONV, or time to discharge [26].
A recent Cochrane review, updated in 2011, found that a single dose of dexamethasone (dose range 0.15–1.0 mg kg–1) not only reduced the PONV rate by approximately one-half, but increased the likelihood of progressing to a solid diet on post-op day one and reduced VAS pain scores without any significant adverse effects [27]. Ondansetron is frequently used in conjunction with dexamethasone for PONV prophylaxis in older children.
Postoperative Complications
The major postoperative concerns after adenoidectomy and tonsillectomy include respiratory issues and hemorrhage. Minor issues include PONV, pain, and poor oral intake. Uvular edema or amputation can occur, but are rare.
Younger children, under three years of age, are more prone to respiratory complications including apnea, breath holding, and supraglottic obstruction. Due to the increased likelihood of needing airway interventions, patients under two years of age are usually not considered candidates for ambulatory tonsillectomies.
The incidence of postoperative hemorrhage is 3–5 percent and is the most common indication for a return to the operating room. The time frame is bimodal, occurring in the first 24 hours or at 7–10 days postoperatively. Disruption of the eschar, either spontaneously or from mechanical trauma (i.e., eating, coughing, etc.) can result in significant bleeding. Volume status should be evaluated preoperatively and fluid resuscitation started. An intraosseous line can be used if there is failure of venous access. The urine output, heart rate, blood pressure, and hydration of mucous membranes can be used to judge the volume status, in addition to lab values. After fluid resuscitation, induction of anesthesia requires a rapid sequence induction (RSI) as swallowed blood constitutes a full stomach. Visualization of the airway may be difficult if bleeding is brisk, and at least two working suction catheters should be available to the anesthesiologist during induction and intubation. Sometimes the only indication of the location of the larynx is the presence of air bubbles to guide ETT placement. Blood transfusion can be given if necessary.
Laser Procedures and Suspension Laryngoscopy
The most common application for lasers in pediatrics involves airway surgery for recurrent papillomatosis. These benign tumors are the result of a human papilloma virus and may occur anywhere on the laryngeal tissues, including the true vocal cords. To facilitate visualization and access to the glottic region, the infant is often placed in suspension laryngoscopy after the induction of general anesthesia. Depending on the size, location, and characteristics of the lesions, they may cause varying degrees of obstruction. Thus, inhalational induction with spontaneous ventilation is the preferred anesthetic technique. As the anesthesiologist slowly takes over the child’s breathing, an IV is placed and airway management addressed.
Visualization of the larynx is accomplished using suspension laryngoscopy. Prior to suspension, propofol (2–3 mg kg–1) should be given as this is quite stimulating. Glycopyrolate and/or atropine should be available to treat bradycardia caused by pressure on the larynx. Once in suspension laryngoscopy, the airway can be managed via several techniques including: (1) conventional ETT intubation, (2) intermittent apnea, (3) jet ventilation, and (4) tubeless spontaneous ventilation. The plan for the airway should be discussed with the surgeon prior to induction of anesthesia. The decision to secure the airway is based on the size and location of the tumor, as well as the surgeon’s need for space while applying the laser to the lesions. Anterior laryngeal lesions are generally not obstructed by the ETT, while posterior lesions will be blocked by the ETT lying directly over the posterior vocal cords and laryngeal structures.
Intermittent apnea is a technique that involves repeated intubations and extubations, which are facilitated by suspension laryngoscopy. During intubation periods, the patient can be hyperventilated and well-oxygenated. The tube can be removed, giving unrestricted access to the glottis. Periods of up to two minutes are generally tolerated quite well. This technique works well for shorter cases where anterior lesions require the absence of an ETT. Due to the apnea, a TIVA is preferable for maintenance of anesthesia.
Jet ventilation is a technique that allows ventilation while maintaining an unrestricted view of the glottis and a quiescent operating field. It requires a special jet ventilator to insufflate the lungs by altering the driving pressure, FiO2, and frequency. A CO2 monitor can be attached to a side port of the suspension laryngoscopy device to monitor the presence of exhaled CO2. Chest rise and SpO2 are used to judge the adequacy of the ventilation. Obese children and those with stiffened chest walls may not show much of a rise in the chest. When in doubt, an arterial blood gas (ABG) can be sent to assess the adequacy of ventilation and other ventilation strategies may need to be considered. In addition to inadequate ventilation, other risks of jet ventilation include gastric insufflation with potential aspiration, pneumothorax, and pneumomediastinum.
Lasers are the second most common cause of operating room fires. The gas mixture and route of delivery are important considerations for laser procedures. Aside from the standard PVC tubes, which are flammable, there are a variety of ETTs available to help reduce the risk of airway fires. These include red rubber tubes that can be wrapped in metallic tape, non-latex tubes, and several ETTs manufactured specifically for laser procedures (see table 3 from [28]). Though routinely used uneventfully in adults, these special ETTs have a large outer diameter and may not be appropriate for infants or children with a partially obstructed airway. It is important to note that the metallic tape-wrapped ETTs are safer than unwrapped tubes, but the cuff remains vulnerable to the laser. Any time lasers are being used, the FiO2 should be less than 0.3, the eyes and face should be covered with wet sponges, and constant communication should be maintained between the surgeon and anesthesiologist. If possible, jet ventilation or tubeless techniques should be used to reduce the risk of fires [28]. Dexamethasone is typically given, 0.5 mg kg–1, to reduce airway swelling as all airway procedures produce varying degrees of controlled injury and inflammation.
References
Introduction
Congenital structural anomalies in the head and neck usually require surgical correction and pose special problems for the anesthesiologist. Management of these infants is best accomplished by an interdisciplinary team of pediatric specialists in plastic surgery, neurosurgery, otolaryngology, ophthalmology, maxillofacial surgery, and pediatric intensive care. Many infants are syndromic and will necessitate multiple procedures. Often there are airway problems and congenital heart anomalies that require careful assessment and anesthetic management.
Cleft Lip and Palate (CL/P)
Cleft lip, with or without cleft palate, is among the most common congenital malformation and presents in a wide spectrum of severity. The incidence is 3.6/1000 in Native Americans, 2/1000 in Asians, 1/1000 in Caucasians, and 0.3/1000 in African Americans. Ninety percent of cleft lips are unilateral and two-thirds are on the left side. Isolated cleft palate (CP) is considered genetically different: It occurs in 0.5/1000 births, more commonly in girls, whereas CL/P occurs more often in boys. Associated anomalies are more frequent in CP (~50 percent) versus CL/P (~10 percent). These infants present with anemia, malnutrition secondary to feeding difficulties, repeated respiratory infections, and impairment in speech.
Classification
Cleft lip is categorized as unilateral or bilateral and designated as incomplete when the skin of the upper lip is not fully separated or complete when the defect involves the nasal floor and alveolus (Figure 22.1).
Figure 22.1 Types of cleft lip: (a) unilateral incomplete; (b) unilateral complete; (c) bilateral complete.
Cleft of the palate occurs in two major forms: soft palate only, which may extend into the posterior hard palate; and complete defect of the soft/hard palate, which is either unilateral or bilateral. The type and extent of palatal clefting is categorized by the Veau classification (Figure 22.2). Submucous CP is a minor form characterized by bifid uvula, thin soft palate due to incomplete muscular formation and a notch in the posterior edge of the hard palate. There is also an occult submucous CP that appears to be intact but can cause hypernasal speech.
Figure 22.2 Veau classification: soft palate only (Veau I); soft/hard palate (Veau II); unilateral complete cleft lip/palate (Veau III); bilateral complete cleft lip/palate (Veau IV).
Surgical Procedures and Timing
Many cleft lip and palate centers use some form of preoperative dentofacial orthopedic manipulation of the maxillary segments for the infant with unilateral and bilateral complete CL/P. A surgical alternative is a preliminary lip adhesion (labial closure) if the cleft is especially wide or if dentofacial orthopedics is not available.
Surgical closure of a cleft lip is usually performed at 4–5 months of age, when the infant is gaining satisfactory weight, and is free of any oral, respiratory, or systemic infection. The most commonly used technique for a unilateral CL is the rotation-advancement method of Millard. Repair of a bilateral CL is often accomplished synchronously with nasal and alveolar closure. Some surgeons stage the correction of the bilateral deformity. Most surgeons repair a CL while standing.
Cleft palate should be repaired at 8–10 months of age, because the incidence of velopharyngeal insufficiency increases after this age. Normal speech becomes increasingly unlikely if palatal repair is delayed beyond one year of age. Closure involves elevating mucoperiosteal flaps from the underlying palatal shelves and approximating these flaps in the midline, including dissection and retro-positioning of the levator muscles. Some centers employ a z-plasty palatal closure (Furlow technique). Most surgeons sit while undertaking repair of the CP.
Usually the infants are discharged on the second postoperative day after CL repair and after CP on postoperative day 2–4.
Associated Conditions
Every infant with CL/P or CP should be assessed for other associated anomalies. There is a long list of syndromes associated with CL/P and CP. Cleft lip/palate centers report that up to 30 percent of affected infants have other anomalies [2]. These congenital anomalies tend to be under-diagnosed at birth and early infancy, especially in those infants with a less severe expression of a genetic disorder. Associated anomalies include malformations of the upper and lower limbs or the vertebral column, the cardiovascular system, and gastrointestinal tract. Bilateral CL/P is a common finding in various trisomies, particularly involving chromosome 13. Nevertheless, most cases of CL/P occur as isolated (sporadic) anomalies, they are not syndromic. Cardiac anomalies occur in approximately 8 percent of CP infants. The most common associated disorder with CP is the Robin sequence, which can be nonsyndromic or syndromic.
Robin Sequence
Robin sequence is a diagnostic term that denotes mandibular micrognathia/retrognathia, glossoptosis (posterior displacement of the tongue), anterior larynx, and respiratory and feeding problems (Figure 22.3). Many infants with Robin sequence have ankyloglossia (tongue tie) which should not be severed until the infant is older and the airway is secure. Usually there is a CP but this is not an obligatory finding for Robin sequence. Most infants are either micro- or retrognathic, but not both. There are over 40 syndromes associated with Robin sequence. Stickler syndrome is the most common cause of Robin sequence (30–40 percent), followed by velocardiofacial syndrome (17 percent) (Table 22.1). In infants with nonsyndromic Robin sequence the mandible may grow to approximately normal size and a nearly normal upper/lower jaw relationship is expected so that the patients “grow out” of the airway obstruction. In infants with a syndromic Robin sequence mandibular growth potential differs depending on the particular syndrome. For example, in Stickler syndrome, mandibular growth is nearly normal. In contrast, in infants with Treacher Collins syndrome or bilateral hemifacial microsomia the mandible will remain hypoplastic and proportionally smaller and the airway problems will persist. (Figure 22.5)
Figure 22.3 (a) Infant with Robin sequence. (b) Intraoral view of U-shaped cleft palate.
Table 22.1 Major syndromes associated with Robin sequence
CHD = congenital heart disease
A few days after birth, the severity of Robin sequence can be clinically graded: eats and breathes well with supine positioning (grade I); breathes well but obstructs when fed by mouth (grade II); cannot breathe or eat without obstruction and desaturation (grade III). Infants in the grade III group present with periods of cyanosis, usually with substernal and intercostal retractions. Feeding through a nasogastric tube might be a short-term solution; some infants need a gastrostomy. Initially the airway may be controlled by placing the infant in a lateral position with the head of the bed slightly raised and using a nasopharyngeal airway or a pacifier to keep the tongue forward. Prone position facilitates drainage of oral secretion and helps posture the tongue forward. Respiratory obstruction may occur combined with central and obstructive sleep apnea which can lead to cor pulmonale. If the infant desaturates despite all attempts of positioning or cannot be fed orally (many grade II and all grade III), operative intervention may include tongue–lip adhesion, mandibular distraction, and, as a last resort, tracheotomy if the tongue–lip adhesion fails (Figure 22.4) [3,4]. Airway management can be complex, with very difficult intubation, so awake techniques may be considered. In skilled hands fiberoptic intubation under general anesthesia may be used. For postoperative extubation infants should be fully awake [5].
Figure 22.4 Left lateral, frontal and right lateral view of former 33-week premature infant with Robin sequence (syndromic) and right predominant hemifacial microsomia. A tracheostomy was placed at age one month because of airway obstruction and apnea along with a gastrostomy for feeding difficulties. Note right micro-ophthalmia, right-sided soft tissue hypoplasia, bilateral mandibular deformity, retrognathia, right macrostomia (transverse oral cleft) and right microtia. He also has cervical vertebral and renal anomalies.
Figure 22.5 Infant with bilateral hemifacial microsomia, predominant on left side, presented with noisy breathing and feeding difficulties requiring gastrostomy. Associated anomalies include bilateral eyelid colobomas, left limbal lipodermoid, facial asymmetry with midfacial hypoplasia, slightly elevated and rotated left nostril, small nasal airways with left-sided choanal atresia, minor bilateral macrostomia, restricted oral opening, micro-retrognathia, ankyloglossia (tongue-tie) glossoptosis, intact palate, bilateral low-set and posteriorly rotated ears with complex pretragal chondro-cutaneous remnants, and cardiac defects. During inhalational induction he did poorly with mask ventilation; two anesthesiologists were required to hold the mask, unable to pass a nasal trumpet but able to insert an oral airway. Direct laryngoscopy and intubation was difficult but possible using a video laryngoscope.
Preoperative Evaluation and Anesthetic Considerations
Careful preoperative assessment, especially of heart, lungs, and airway, is mandatory to reveal associated conditions and abnormalities related to syndromes (Table 22.1). Infants with a large cleft and acute respiratory infection tend to have a higher intra- and postoperative complication rate which should initiate a discussion with the surgeon to postpone the operation [6]. Cleft lip repair involves minimal blood loss. Cleft palate repair has more blood loss but usually does not require blood transfusion in otherwise healthy infants with hematocrit values greater than 30 percent.
In our experience, tracheal intubation is not particularly difficult in most infants with CL/P, unless concomitant defects are present. The incidence of a difficult airway in infants with CL/P ranges between 5 and 10 percent [7–9]. The incidence of difficult laryngoscopy is 7 percent in the age group of 1–6 months old infants and is greatest for infants with bilateral CL/P [8]. Midfacial anomalies, mandibular hypoplasia (retro- or micrognathia, e.g., in Robin sequence, hemifacial microsomia, Treacher Collins syndrome) or restricted cervical movement are indicators of serious airway problems. If micrognathia is present, the incidence of difficult laryngoscopy is approximately 50 percent whereas without micrognathia it is about 4 percent [8]. Intubation difficulty decreases with increasing age in infants with nonsyndromic Robin sequence; however, in syndromic Robin sequence it differs depending on the particular syndrome (Table 22.1). Given the difficulty of recognizing micrognathia and retrognathia in young infants and that the ear and mandible develop from the first and second pharyngeal arches and first branchial cleft, abnormalities of the ear should prompt a closer examination of the mandible for hypoplasia. The presence of isolated microtia is also an indicator for difficult intubation with an incidence of difficult laryngeal view of 42 percent in patients with bilateral microtia, and 2 percent in those with unilateral microtia [10]. A careful review of previous anesthetic records may forewarn of a difficult airway.
Induction of anesthesia is usually performed in a spontaneously breathing infant with a non-irritating inhalational agent and is expected to be uncomplicated in an infant presenting for closure of simple CL/P with a history of airway patency during natural sleep (no snoring or sleep apnea). In the presence of a wide cleft palate the tongue can fall into the cleft and may obstruct the nasal airway. With increased relaxation of the oropharyngeal muscles the tongue will fall further posteriorly and may obstruct the oropharynx completely, which can be prevented by placing an oropharyngeal airway. Spraying the mouth with a topical anesthetic prior to induction will allow placement of an oral airway at light depths of anesthesia. If the anesthesiologist is comfortable ventilating the infant with a mask, a nondepolarizing muscle relaxant can be administered. Intubation of a patient with unilateral right-sided cleft is usually straightforward. Infants with left-sided complete cleft are more difficult because the laryngoscope blade will tend to fall into the cleft as the tongue gets swept to the left side and alters the line of vision. In bilateral CL/P, the premaxilla is angled anteriorly, altering the anesthesiologist’s line of sight onto the entrance of the larynx and hampering the insertion of the laryngoscope blade. To prevent traumatic laryngoscopy and intubation, the wide or bilateral cleft can be packed with moist sterile gauze. Sometimes external laryngeal manipulation of the larynx is useful during direct laryngoscopy if the mandible is hypoplastic. Infants with Robin sequence and associated craniofacial syndromes have a small oral cavity because of a hypoplastic mandible (Table 22.1); the larynx is positioned cephalad and anterior under the base of the tongue, and airway management requires more planning. If the patient has a history of lack of airway patency during sleep, successful ventilation by mask might be very difficult or unlikely. Consequently, alternative techniques for securing the airway need to be considered such as sedated/awake techniques, fiberoptic intubation, intubation through a laryngeal mask airway, and in severe cases tracheostomy [11]. In severe cases an otorhinolaryngologist should be available to help with the airway management and assessment for other causes of obstruction such as anomalies of the epiglottis and laryngomalacia.
For orotracheal intubation a variety of tubes are available. The Ring–Adair–Elwyn (RAE) or the microcuff Kimberly Clark preformed tracheal tube are frequently used and can be fixed on the chin, placed over the midline of the lower lip to facilitate optimal surgical access and visualize lip and palate. In infants with micro- and retrognathia the preformed tubes can be too long, which results in one-sided intubation. The advantage of the microcuff tube is the slimmer cuff which is positioned further distal (no Murphy eye) compared to the RAE tube, which facilitates appropriate midtracheal placement. The tube and the tubing system need to be carefully arranged when the operating room table is turned 90 or 180 degrees away from the anesthesia provider to prevent inadvertent extubation. Care must be taken that bilateral ventilation of the lungs is present during all repositioning maneuvers by the surgical team and after the mouth gag and throat pack is positioned. Insertion of the gag and repositioning of the neck tends to advance the tip of the tube in the trachea, with the risk of one-sided ventilation. The eyes need to be carefully protected before incision. Monitoring should include the standard ASA monitors and ventilation parameters (compliance, ventilation pressures, tidal volume) to recognize compression of the tube caused by surgical maneuvers or obstruction (e.g., secretion).
Maintenance of anesthesia is achieved with low doses of inhalational agents combined with short-acting opioids (e.g., 1–2 μg kg–1 fentanyl for induction as well as for incision and repeated doses during each consecutive surgical hour) or medium-acting opioids (e.g., 30 μg kg–1 morphine for induction, as well as for incision and 30–40 minutes before emergence). Very short-acting opioids, like remifentanyl, are avoided in our practice because of the risk of developing hyperalgesia during the immediate postoperative period. Local infiltration with local anesthetic (e.g., lidocaine 0.5 percent or bupivacaine 0.25 percent with epinephrine 1:200 000) and bilateral infraorbital blocks during CL repair may reduce the amount of opioids and risk of postoperative respiratory depression [12–15]. In neonates, Boesenberg et al. prefer a percutaneous technique instead of a perioral one, with bupivacaine 0.5 percent 0.5–0.75 ml with epinephrine 1:200 000 [16]. Communication with the surgeon and parents is recommended because even small amounts of local anesthetic can cause edema and small hematomas in the cheeks.
Extubation, Acute Airway Obstruction and Postoperative Care
At the end of the procedure infants should wake up in an unagitated state, as hypertension and undue crying may cause bleeding or place excessive tension on the repair. After cleft repair, the minimal to moderate amount of blood in the oropharynx needs to be carefully suctioned. Extubation should be performed after airway reflexes have returned in an infant that is awake, opens the eyes, and presents with regular breathing and good muscle tonus. There may be some respiratory difficulties on emergence, including acute upper airway obstruction as a result of upper airway narrowing due to the surgical reduction of the pharyngeal space, edema, and residual anesthetic effects. All infants with a nonsyndromic or syndromic Robin sequence who presented with a difficult airway management during induction should be observed in the NICU/ICU post CP repair. Rare cases are reported of massive lingual swelling in infants with Robin sequence as well as in otherwise healthy infants follow CP closure. The reasons might be prolonged procedures, head-down position, and prolonged lingual retraction [17,18]. In selected cases surgeons provide a tongue suture for traction which often proves helpful in restoring the infant’s airway. In some institutions after CL repair, surgeons like to use a Logan bow to protect the operative site combined with a saline-soaked gauze over the wound (Figure 22.6). In these cases awake extubation with good airway reflexes is of special importance because mask placement and ventilation is impossible with the bow in place. The saline-soaked gauze is used for the first 24 hours to prevent crusting and the bow is temporary. Arm restraints are used in many centers to prevent trauma to the labial repair. During the postoperative course vigilance and monitoring is required for upper airway obstruction (48 hours), late postoperative edema, subcutaneous emphysema and negative pulmonary pressure edema. Any signs of airway obstruction and stridor require careful attention and treatment with corticosteroid and racemic epinephrine to prevent reintubation. Postoperative bleeding is difficult to detect because the blood is usually swallowed. Postoperative analgesics should be titrated to avoid causing sedation and airway obstruction. Pain is managed with acetaminophen and IV opioids can be titrated in the PACU with conversion to oral opioids as soon as oral intake permits. Feeding is usually initiated as soon as the infant is awake.
Figure 22.6 Five-month-old infant with unilateral left incomplete cleft lip following rotation advancement repair and correction of minor nasal deformity. Xeroform gauze-wrapped tubing inserted in left nostril. Logan bow (left and center picture) holds an iced saline sponge (right picture). The infant was extubated fully awake and recovered in the postanesthesia care unit.
Craniosynostosis
The estimate of prevalence of craniosynostosis ranges from 0.4 to 1 per 1000 live births. [19] It is defined as a premature closure of one or more calvarial sutures with failure of normal growth perpendicular to the suture and compensatory overgrowth parallel to the affected suture. The abnormal cranial shape is determined by the specific sutures involved (Table 22.2). The clinical diagnosis is confirmed by plain skull roentgenogram or CT scan. Uncorrected craniosynostosis can have lifelong effects on appearance and neurocognitive development.
Table 22.2 Nomenclature of craniosynostosis
Classification
Craniosynostosis occurs as either an isolated single fusion (80 percent) or as multiple-sutural fusion (20 percent) that can be syndromic or nonsyndromic. Sagittal craniosynostosis is the most common form (sagittal: 50–60 percent, coronal 20 percent, metopic 10 percent). Increased intracranial pressure can occur in 15–20 percent of infants with single sutural synostosis and in 40–70 percent of those with syndromic craniosynostoses [20]. Surgical intervention is indicated to prevent complications such as cerebral compression, auditory impairment and visual loss [21–24]. The etiology of most cases is sporadic, although there is often a strong genetic component. Genetic mutations that are responsible for the syndromic craniosynostoses include mutations in the fibroblast growth factors (FGFR 1, FGFR2, FGFR3), TWIST, and MSX2 genes.
Progressive postnatal craniosynostosis, a rare presentation, is characterized by a normal cranial shape and normal radiological images at birth. Later in childhood there is slow development of intracranial pressure (ICP), with signs/symptoms such as headaches, vomiting, irritability, papilledema, progressive optic atrophy, seizures, and/or bulging fontanelles [25].
Positional plagiocephaly, also known as deformational plagiocephaly, is extremely common and can be confused with craniosynostosis, particularly lambdoid fusion. There is characteristic unilateral occipital flattening and anterior displacement of the ipsilateral ear. This harmless condition is treated nonsurgically by an orthotic helmet if initiated early enough [26,27].
Craniosynostotic syndromes and implications for preoperative assessment
There are important anesthetic implications in the care of infants with craniosynostoses, which can be associated with numerous syndromes. The most frequent ones are detailed in Table 22.3.
Syndrome | Description | Anesthesia implications |
---|---|---|
Apert | Most common coronal synostosis, possible increased ICP; exorbitism, midface hypoplasia, choanal stenosis, airway anomalies (2 percent); syndactyly of hands and feet, synphalangism, partial cervical spine fusion C5/6 (70 percent); CHD (10 percent); genitourinary anomalies; cognitive impairment. | Obstructive sleep apnea; possible difficult intubation; possible increased ICP; evaluation for CHD. |
Crouzon (craniofacial dysostosis) | Multiple suture synostosis (coronal, sagittal), possible increased ICP resulting from progressive hydrocephalus, intracranial abnormalities (Chiari); exorbitism; midface hypoplasia, choanal atresia, airway obstruction; low-set ears; rare cardiac anomaly; vertebral anomalies C2/3; mental retardation. | Difficult airway management, elective tracheostomy might be indicated; possible increased ICP; ocular protection. |
Muenke | Most common craniosynostosis syndrome; coronal synostosis; midface hypoplasia rare. | Possible mild obstructive sleep apnea. |
Saethre Chotzen | Unilateral or bilateral coronal suture synostosis (brachycephaly, plagiocephaly); possible increased ICP; maxillary hypoplasia; CHD; syndactyly, cervical spine abnormalities, clavicular anomalies. | Possible difficult intubation due to cervical vertebral fusion; possible increased ICP. |
Pfeiffer | Multiple suture synostosis (brachycephaly, turricephaly, most common cause of trilobar cranial deformity (Kleeblattschädel)); possible increased ICP, Chiari malformation; ocular proptosis; midface hypoplasia; CHD; cervical vertebral fusions, mild syndactyly, short and broad thumbs and toes. | Possible difficult airway; obstructive apnea; possible seizures (interaction anticonvulsants with anesthetic drugs); evaluation for CHD. |
CHD = Congenital heart disease.
Airway comorbidity. Children, especially those with syndromic craniosynostosis, can present with a compromised airway. Although the incidence of sleep disorder and obstructive sleep apnea syndrome is high, it is often unrecognized [28,29]. Obstructive sleep apnea symptoms occur in almost half of the children during episodes of upper respiratory tract infections. Major upper airway obstruction is found more frequently in patients with Crouzon, Pfeiffer, and Apert syndromes and less often in patients with Muenke and Saethre-Chotzen syndromes [30]. Causes for severe airway obstruction include mid-facial hypoplasia, choanal atresia, and also lower airway anomalies. Tracheal cartilaginous sleeve is a rare congenital cartilage malformation reported only in children with craniosynostosis syndromes and is characterized by fusion of the tracheal arches that may be isolated to a few tracheal arches, involves the entire trachea, or extends beyond the carina into the bronchi [31]. Infants with syndromic craniosynostosis can present with difficult airway management [11,32] but rarely require tracheostomy, unless there is marked facial dysmorphism [33].
It is important to consider the effect of chronic airway obstruction on the cardiovascular system and the central nervous system. Patients who have a history of longstanding airway obstruction may have chronic hypoventilation and hypoxia that can lead to pulmonary hypertension and subsequently cor pulmonale. The chronic respiratory obstruction forms part of a vicious cycle with increased intracranial pressure and a subsequent decrease in cerebral perfusion pressure [34]. Recurrent episodes of intermittent reduction of cerebral perfusion pressure most likely have a negative effect on neurological and cognitive development in the long term.
Cardiac comorbidity. Craniosynostotic syndromes can occasionally be associated with cardiac anomalies (Table 22.3). Congenital heart disease, both repaired and unrepaired, has been shown to increase the risk for anesthesia and any surgery. Infants with patent foramen ovale or ductus arteriosus are at risk for paradoxical air emboli (coronary or cerebral air embolism) through these defects.
Neurological Comorbidity. Several factors contribute to the risk of developing increased ICP, including decreased intracranial volume and increased number and location of sutures involved [35]. Although it is well known that increased ICP is commonly associated with multiple suture craniosynostosis [30], ICP monitoring has demonstrated that 14–24 percent of infants with single-suture craniosynostosis also have raised ICP [24,36]. Positron emission tomography scans have shown reduced cortical blood flow in the vicinity of sagittal synostosis in 70 percent of cases, which can be corrected by early release [37]. Hydrocephalus is seen sometimes in patients with craniosynostosis. It is particularly associated with Crouzon and Pfeiffer syndrome, and Kleeblattschädel (cloverleaf skull syndrome; see Figure 22.9). Multisuture craniosynostoses, particularly with lambdoid involvement, are associated with a higher incidence of acquired Chiari deformation, require multiple operative procedures, and cause more developmental delay than isolated single-suture synostoses [38]. Evidence of jugular foraminal stenosis or atresia and dilated collateral emissary veins in children with syndromic craniosynostosis is associated with venous outflow obstruction and elevated intracranial venous hypertension [39,40]. Disruption of emissary veins during an operation can produce massive hemorrhage. Enlarged basal emissary foramina which transmit enlarged emissary veins result from stenosis or atresia of the jugular foramen. Bilateral basilar venous atresia is most common in patients with the FGFR3 ala391glu mutation (crouzonoid features with acanthosis nigricans) but also may be found in patients with FGFR2 mutations [39]. Preoperative assessment of patients with syndromic craniosynostosis and enlarged emissary foramina should include skull base vascular imaging of the basilar venous drainage [39]. Significant increased intracranial bleeding is expected during craniectomy.
Ophthalmologic Comorbidities. Ophthalmologic examinations may show papilledema and optic atrophy with chronically raised ICP [41]. Syndromic craniosynostoses with shallow, deformed orbits, and exorbitism increases the risk of corneal abrasion and ocular trauma during an operation.
Cervical vertebra anomalies, particularly in Apert, Pfeiffer, and Crouzon syndromes, limit neck motion (flexion and extension) and can increase the difficulty of intubation and positioning for the operation.
Surgical Procedures and Timing
The type and timing of the operative correction for craniosynostosis varies with age, location, and number of sutures involved, and the experience of the surgical team. Indications for repair of craniosynostosis include: increased ICP, cranial deformity, exorbitism, airway obstruction, and psychosocial reasons.
Endoscopic-assisted strip craniectomy (ESC) is considered for infants less than three months of age, usually for single-suture synostosis and in selected cases for multiple sutural synostosis. Endoscopic-assisted strip craniectomy is a short surgical procedure (approximately 30–45 minutes) associated with low incidence of perioperative blood transfusion (0–11 percent) [42–45], VAE (2–8 percent) [44,45], ICU admission (8 percent) [44], and expense [46]. Most infants are discharged on postoperative day one. Postoperative molding helmet therapy for approximately eight months (Figure 22.7) is an integral part of this treatment modality necessary to counteract the tendency of the cranial vault to revert to premorbid shape after strip craniectomy [43].
Figure 22.7 Endoscopic-assisted strip craniectomy (ESC). Upper left and center picture: Infant in prone position for sagittal ESC on a modified prone head holder in sphinx position. It illustrates the extended head position and the significant distance between the highest point of the surgical field to the right atrium, a risk factor for venous or paradoxical air embolism. Upper right picture: Intraoperative picture of sagittal ESC with two skin incisions perpendicular to the fused sagittal suture: one posterior to the coronal sutures and the other at the junction of the lambdoid sutures. After endoscopic suturectomy, the 1–2 cm wide bone strip of the fused sagittal suture is removed. Lower pictures: Postoperative orthosis after ESC. Infant with sagittal synostosis after sagittal ESC wearing the helmet with openings at the side that allows the growing brain to push the skull out which finally results in the desired round head form.
Patient positioning during neuroendoscopic procedures remains one of the paramount concerns in providing optimal surgical access and exposure of the suture for visualization and resection. Sagittal ESC and lambdoid ESC are usually performed in prone position, often using special headrests (e.g., Doro headrest system, PMI, Freiburg im Breisgau, Germany; Figure 22.7) and metopic and coronal ESC in supine position, commonly using the cerebellar headrest. The endotracheal tube has to be carefully placed in midtracheal position to prevent inadvertent extubation or bronchial mainstem intubation, taking into account the resultant displacement related to the head positioning (Figure 22.7).
Cranial burr holes are made through the midline of each scalp incision. An endoscope is used to visualize the fused suture, identify emissary veins, dural attachments, and assure hemostasis. The fused suture is resected as a 1 cm wide bony strip and removed through the burr hole (Figure 22.7). Bone bleeding is controlled using bone wax and thrombin-soaked absorbable gelatin (Gelfoam, Pharmacia and Upjohn, Kalamazoo, MI).
Spring-assisted cranioplasty involves implanted springs in the synostosed sutures that allow gradual correction of the cranial malformation over time. It is used in infants older than three months of age with single sutural craniosynostosis and in selected infants with multiple sutural synostoses [47,48]. Different surgical groups report varying mean surgical times (range 30–170 minutes for insertion and 20–60 minutes for spring removal) and need for blood transfusion ranging from no blood transfusion [49] to blood loss comparable to open strip craniectomy [48,50]. This technique involves an osteotomy and resection of the prematurely closed suture combined with the insertion of 1–3 stainless steel springs across the newly created cranial gap (Figure 22.8). The expansion of a spring is a slow process and is monitored both clinically and radiologically. The springs are surgically removed after adequate cranial expansion (2–7 months).
Figure 22.8 Spring-assisted cranioplasty: After midline sagittal osteotomy, two omega-shaped springs are placed and cause a separation/distraction between the parietal bones. The two skin flaps created by the lazy-S incision are held aside with sutures.
Open cranial procedures are usually performed in infants at ages 6–12 months. The surgical procedures vary in the extent of cranial elements moved, risk of dural tear, sinus injury, and blood loss (simple strip craniectomy, π cranioplasties, cranial vault remodeling). Anterior calvarial procedures and fronto-orbital advancement involve bilateral craniectomy and sometimes require also posterior cranial vault remodeling, with or without barrel stave osteotomies. These techniques are associated with major blood loss, varying between 0.2 and 4 blood volumes, lengthy operative times (3–8 hours), and hospital stay (4–7 days), and require postoperative monitoring in a pediatric intensive care unit [51–53].
Anesthetic Considerations
Induction, Airway Management, and Monitoring
Despite the usually obvious craniofacial deformity, the infant’s underlying neurodevelopmental status and general health is frequently quite normal. An infant with mental retardation may not cooperate upon separation from the parents or during induction of anesthesia. Premedication should be cautiously considered in the presence of increased ICP, obstructive sleep apnea, and airway difficulties.
During induction, difficult airway management in patients with airway compromise should be expected (Table 22.3). Infants with syndromic craniosynostosis have some degree of midfacial hypoplasia with normal temporomandibular joints and normal-sized mandible (which only appears relatively prognathic), a high arched palate, small nasal passages, and some degree of choanal stenosis that causes primary mouth breathing. Management of the mask airway might be difficult because of the small, flat midface and ocular proptosis causing a problematic fit. Closure of the mouth occludes the oral airway as the tongue fills the smaller oral cavity, while the small nares and choanal stenosis cause resistance to nasal air flow. This problem can be overcome by gently holding the mouth open during induction (instead of closing it) and pressing down with the mask to obtain a good seal. Alternatively an oral airway can be used. In most cases tracheal intubation is not difficult unless there are major vertebral abnormalities and reduced cervical mobility or in the presence of craniofacial syndromes (Table 22.3). Infants with severe airway problems often have obstructive apnea and may require perioperative CPAP or tracheostomy. Abnormalities of the trachea might necessitate using a smaller-sized tube. Meticulous attention to midtracheal tube placement and secure fixation is essential because the infant’s head is constantly moved during these cranial procedures, both rotated and extended. Intraoperative access to the airway is limited and the loss of the airway during the procedure is life-threatening. Most experienced surgical teams do not rely on taping the endotracheal tube to the nose or chin. A nasotracheal tube should be secured with a trans-septal suture, an orotracheal tube with a circum-mandibular wire for cranial vault remodeling procedures.
Ventilation should be performed with O2/air and aimed at normocapnia or mild hypocapnia.
Protection of the eyes must be provided by the anesthesia/surgical team (i.e., lubrication, and tarsorrhaphy sutures) to prevent corneal damage, especially if there is ocular proptosis.
Monitoring includes the standard ASA monitors, several large-bore peripheral intravenous catheters sufficient for rapid transfusion, central line if vascular access is a problem, arterial invasive monitoring if ongoing blood loss is a concern, for hemodynamic monitoring and blood sampling. Laboratory capabilities for rapid assessment of blood gases, electrolytes, metabolic status, and coagulative status, including thromboelastography, should be available. A bladder catheter is necessary to monitor urinary output (0.5 ml kg–1). A variety of different monitors are used for detection of venous air embolism well before cardiovascular collapse occurs, e.g. precordial Doppler ultrasonography (characteristic change of signal, second highest sensitivity), end-tidal carbon dioxide (precipitous decrease in carbon dioxide tension), end-tidal nitrogen monitoring (sudden increase in the nitrogen concentration in the exhaled breath), transesophageal echocardiography (presence of air in the right ventricular outflow tract, highest sensitivity) [54–59]. Insertion of central catheters for withdrawal of air is limited to a 33 percent success rate by the diminutive size of the infant and catheter because the ability to rapid aspirate air decreases with the size of the catheter.
Maintaining normothermia can be a major problem in infants in any lengthy procedure involving exposure of the large surface area of the head. During induction the operating room should be warmed up and warming lights and warming blankets used. Fluid warmers should be used throughout the surgical procedure. Low-flow gas anesthesia or heated humidifiers in the airway circuit minimizes evaporative heat loss from the respiratory system.
Maintenance of Anesthesia
For maintenance of anesthesia a balanced anesthetic technique is usually used that includes inhalational agents, opioids, muscle relaxants, and antiemetics. The depth of anesthesia is titrated to maintain hemodynamics within 20 percent of baseline (heart rate, blood pressure). Opioids are titrated to the level of surgical stimulation. During fronto-orbital advancement (anterior cranial vault remodeling) oculocardiac reflex might be triggered, especially in young infants, by direct ocular pressure or ocular manipulation which most commonly leads to sinus bradycardia but also junctional rhythm, ectopic beats, atrioventricular block, or ventricular tachycardia. If notification of the surgeon to stop the stimulation does not resolve the arrhythmia/bradycardia, atropine (20 μg kg–1) and ephedrine should be considered. Perioperative corticosteroid reduces the postoperative edema of the eyes, which may swell shut during the first postoperative 24–48 hours, and shortens hospitalization without increasing the incidence of postoperative infection [60]. At the end of the procedure the patient should awaken comfortably, be appropriate for neurological assessment, and maintain a natural airway.
Fluid Management, Blood Loss, and Blood Conservation
Close attention to fluid homeostasis and intravascular normovolemia is required to maintain adequate perfusion of tissue beds, kidneys, and particularly the brain in the presence of increased ICP. Maintenance should be substituted with isotonic crystalloid solutions and blood loss replaced 1:1 with blood products and blood derivatives. One study suggested that lactated Ringer’s solution is the preferred crystalloid solution because it is less likely to induce metabolic acidosis than normal saline [61].
The extent of blood loss and the need for invasive monitoring are determined by the type and duration of the procedure, type of cranial malformation, number of sutures involved, and extent of osteotomies and bony movements.
Endoscopic-assisted strip craniectomy is a short procedure, rarely requiring blood transfusion. In healthy infants in our institution we forgo routine invasive arterial blood pressure monitoring in favor of two large-bore peripheral IV catheters, which are suitable for intraoperative venous blood sampling and rapid transfusion of blood products and derivatives. Appropriate IV access is especially important for low body weight infants (<5 kg), syndromic infants, and those with sagittal synostosis for whom there is a risk of sudden, abrupt bleeding from the sinus.
For spring-assisted cranioplasty, different centers report various degrees of bleeding and incidence of blood transfusion. As ESC and spring-assisted cranioplasty procedures are performed in young infants, the anesthesiologist should have always typed and crossed blood products readily available in the operating room.
Open reconstruction procedures have a higher comorbidity due to massive hemorrhage and potential cardiac arrest [62,63]. Blood loss occurs continuously throughout the procedure, but is especially brisk during craniectomy and if there is damage to cerebral venous sinuses. Estimation of ongoing blood loss can be difficult because of the use of large volumes of irrigation fluid and blood loss onto surgical drapes. As major blood loss has to be anticipated, at least one blood volume of blood products as well as a continuous infusion of a vasopressor (e.g. dopamine) should be available in the operating room before incision. Massive blood replacement is complicated by electrolyte and metabolic disturbances, coagulopathy, dilutional thrombocytopenia, and calcium-mediated hypotension [64,65]. High serum potassium levels, arrhythmias, and hyperkalemic cardiac arrest can occur during rapid transfusion, but also when modest amounts of old blood with high potassium concentration is transfused via a central line into the right atrium [66–69]. Administration of blood products is preferably delivered by the peripheral catheters because the central venous access delivers the more concentrated potassium load directly to the right heart through the pulmonary circulation to the left heart, where coronary circulation occurs. Discussion between the anesthesiologist and the blood bank should include (1) reservation of fresh and non-irradiated blood (less than seven days old) for infants less than one year of age undergoing a procedure necessitating rapid transfusion of blood products; or (2) use of washed blood products if those have been irradiated to diminish the possibility of graft-versus-host disease. Irradiation damages the RBC membrane and increases the erythrocyte membrane permeability, potassium leakage, and the potassium concentration in the extracellular fluid [70,71]. Therapy of a life-threatening arrhythmia includes administration of calcium gluconate, sodium bicarbonate, glucose and insulin, hyperventilation, and albuterol.
Massive blood loss requires a prompt coagulation screen and replacement therapy with platelets, fresh frozen plasma, or cryoprecipitate, as required. A coagulopathy should always be anticipated once the blood loss exceeds one blood volume. All intravenous fluid must be administered via a fluid warmer to prevent hypothermia. Maintaining normothermia helps to preserve normal coagulative indices.
Blood conservation techniques to reduce intraoperative blood loss have shown arguable results, have been evaluated in studies with single center design, and are based on small patient numbers. Strategies include: (1) tissue infiltration of a dilute vasoconstrictor solution (epinephrine 1:200 000 or less) to decrease blood loss upon incision through the scalp; (2) surgical technique using a Colorado needle; (3) preoperative administration of recombinant human erythropoietin to increase preoperative hematocrit [72], also used as dual therapy with cell saver [73], or combined with iron substitution [74]; (4) intraoperative antifibrinolytics; and (5) normovolemic hemodilution. Interest in the use of antifibrinolytics was generated by two randomized, double-blind, placebo-controlled studies that demonstrated the effectiveness of the antifibrinolytic agent tranexamic acid (TXA) in reducing blood loss and perioperative transfusion requirements in children with extensive craniofacial procedures [75,76]. Several dosing regimens have been described (loading dose of 15 mg kg–1 TXA followed by an infusion of 10 mg kg–1 h–1 until skin closure [75]; 50 mg kg–1 TXA loading dose followed by an infusion of 5 mg kg–1 h–1 until skin closure [76]), but the lowest effective intraoperative dosing regimen of TXA is uncertain, as well as the effective therapeutic plasma concentration of TXA to inhibit fibrinolysis. A combination of blood conservation techniques and an appropriately chosen surgical technique may best reduce the transfusion rate [77]. Normovolemic hemodilution and induced hypotension may compromise tissue oxygen delivery during rapid blood loss, particularly in small infants, and therefore are not suitable techniques for infants.
Venous Air Embolism
Venous air embolism (VAE) may occur during any operative procedure in which the surgical site is above the level of the heart and non-collapsible veins are exposed to air [54]. With the operative site above the level of the heart, a pressure gradient may develop that favors air entry rather than bleeding if a vein is opened. The highest incidence of VAE during operative correction of craniosynostosis is reported by Faberowski et al. at 83 percent using precordial Doppler; Harris et al. reported an incidence of 66 percent using trans-thoracic echo [55,56]. Although hemodynamically significant VAE is rare [78], preemptive placement of a monitor for early recognition may allow for timely initiation of therapy, thereby decreasing morbidity and mortality rates. For example, a precordial Doppler ultrasound is a noninvasive, inexpensive, very sensitive (nonspecific), safe method and can detect minute VAE (0.05 ml kg–1) [79] by a change in the character and intensity of the emitting sound (erratic, high- pitched swishing roar, “drum like” or “mill wheel” sound with greater air entrainment). The Doppler probe is best positioned on the anterior chest, usually just to the right of the sternum at the fourth intercostal space. In prone position an alternate site on the posterior thorax, between the right scapula and the spine, can be used in infants weighing approximately 6 kg or less [59]. The correct placement can be verified by rapid intravenous injection of 5–10 ml of agitated saline peripherally and the subsequent observation of characteristic Doppler tones. Other hints for VAE include sudden drops in end-tidal CO2, hypotension, and dysrhythmias and/or ischemic changes in the electrocardiogram. The risk of VAE can be minimized by early detection, and maintaining euvolemia to prevent a decrease in central venous pressure caused by bleeding. The development of a pressure gradient between the surgical side and the right atrium increases the potential to entrain air via dural sinuses or bony venous sinusoids. Several therapeutic maneuvers are used simultaneously with the detection of hemodynamically significant air: (1) administration of 100 percent oxygen and fluid (often packed red blood cells); (2) application of bone wax or direct pressure to seal the sites of egress; (3) flooding the surgical field with warm saline; (4) lowering the surgical field using the Trendelenburg position; (5) positive pressure ventilation of 5 cm end-expiratory pressure; and (6) attempt to aspirate air from a right atrial catheter if a catheter is in place. These maneuvers will augment the patient’s blood pressure and prevent further entrainment of intravascular air. Compression of the jugular veins increases ICP, thereby reducing cerebral perfusion, which is a severe limitation of this technique. For catastrophic VAE with cardiovascular collapse, use of inotropic support and, if necessary, cardiopulmonary resuscitation are standard measures. Extracorporeal membrane oxygenation (ECMO) might be used to clear out air in the heart. Open remodeling procedures are associated with large blood losses and frequent hypotensive episodes. Consequently, VAE might be overlooked as a possible cause of intraoperative hypotension if precordial monitoring is not utilized. During ESC a much lower incidence of VAE (2–8 percent) is reported [44,45]. This low incidence of VAE during ESC most likely relates to the low average blood loss corresponding with less decrease in central venous pressure with ESC compared to the significant amount of blood loss approaching 100 percent and more of estimated blood volume during traditional cranial vault remodeling procedures.
Increased Intracranial Pressure
Infants with single suture and more often with syndromic craniosynostosis may have increased ICP. In these cases basic principles of neuroanesthesia to prevent further increase in ICP and prevent decreases in cerebral perfusion pressure should be followed until craniectomy is performed. Accordingly, the anesthesiologist should minimize factors that increase ICP, such as hypercapnia and hypoxia, and factors that increase venous pressure such as the patient’s position and coughing. In the presence of intracranial hypertension and herniation, mild to moderate hyperventilation, appropriate use of mannitol, furosemide, and dexamethasone may be employed to reduce ICP and brain volume. Although cranial vault remodeling increases intracranial volume, infants remain at risk of increased ICP after surgery and require close follow-up.
Postoperative Care
The majority of patients are extubated after the surgical procedure. Postoperative intubation and admission to the intensive care unit or PACU/ward depends on the duration of the operation, hemodynamic stability, preexisting comorbidities (preoperative respiratory compromise, obstructive sleep apnea, difficult intubation), and potential complications during the procedure, including hypothermia, massive fluid resuscitation, electrolyte disturbances, etc. Since the surgical procedure is focused in the cranium, the airway rarely becomes edematous except by mechanical irritation of the vocal cords caused by the tube secondary to frequent head repositioning. In selected cases, if facial morphology permits noninvasive ventilatory support, CPAP or BiPAP may be a postoperative option. The initial management centers upon airway monitoring, sedation, pain management, thermoregulation, and correction of any residual volume deficits, acidosis, or coagulopathy. Isotonic solutions are maintained until fluid shifts are complete. Of particular importance is the risk of hyponatremia. The cause of hyponatremia is likely to be related to antidiuretic syndrome or administration of hypotonic intravenous fluids. Close hemodynamic and neurologic monitoring is provided until the risk of bleeding has passed. Coagulopathy may be consumptive or dilutional in nature and requires specific correction. Continued blood loss via drains needs to be monitored and replaced appropriately and vigilance maintained for additional concealed losses.
Congenital Hand Deformities
Syndactyly is one of the most common congenital hand abnormalities. It may affect fingers and/or toes. Surgical treatment is a reconstructive procedure with the purpose of separating the fingers and reconstructing a webspace to enhance hand function. The term simple syndactyly is used if only the soft tissues are involved and complex syndactyly if the bones of adjacent fingers are fused. Complicated syndactyly describes syndactyly with bone formation between two digital rays. For syndactylies of the first and fourth interdigital webs and syndactylies with fusion of several fingers, surgical treatment is recommended at the age of 4–9 months to provide optimal assurance of normal grip and pinch development [80]. Syndactyly can occur as an isolated anomaly, can be associated with other anomalies of the extremity, or can be part of a syndrome (Figure 22.9). The most common syndromes are: (1) Apert syndrome; (2) Poland syndrome (skeletal abnormalities, unilateral hypoplasia or aplasia of the chest wall muscles, mainly pectoralis major, gastrointestinal, and cardiac malformations); (3) amniotic band sequence (Streeter anomaly, constricting amniotic bands can lead to amputation of all or parts of a limb, one or more digits, distal limb hypoplasia, cleft lip and palate, anencephaly, encephalocele, and other internal organ anomalies); and (4) multiple craniofacial syndromes (Table 22.3).
Figure 22.9 Pfeiffer syndrome (FGFR2 mutation) and a “cloverleaf” cranial deformity (coronal, posterior sagittal, and lambdoid synostosis), orbital hypertelorbitism, proptosis, midfacial hypopoplasia, high arched palate, choanal stenosis, Chiari I malformation, obstructive sleep apnea, scoliosis, and hand abnormalities (radial clinodactyly of both thumbs, bilateral fourth–fifth metacarpal synostosis) as well as simple syndactyly of both feet.
Precautions before anesthesia include assessment for associated head/face, thorax, cardiac, neurological, and respiratory abnormalities. Limb defects may make vascular access difficult. In the presence of facial malformations, the potential for difficult airway management must be anticipated (Table 22.3). An association has been reported between syndactyly and prolonged QT interval with life-threatening arrhythmias and high risk of sudden death [81]. The authors recommend for infants presenting with syndactyly and any type of congenital heart disease a preoperative ECG with careful evaluation of QTc. Timothy syndrome is a rare disorder characterized by the co-occurrence of both syndactyly and long QT syndrome, associated with other cardiac, facial, and neurodevelopmental features [82].
Congenital radial deficiency is a rare anomaly and encompasses a spectrum ranging from partial to complete absence (unilateral and bilateral) of the radius. The hand is found to be radially deviated, unstable, flexed, and pronated on the forearm as a result of varying degrees of hypoplasia or aplasia affecting the soft tissues and the skeletal elements on the radial side of the distal forearm and hand. With congenital absence of the radius, other deformities are associated, e.g., cleft lip and palate, clubfoot, hydrocephalus, absence of fusion of the ribs, aplasia, or collapse of the lung and hemivertebrae, as well as syndromes (e.g., VACTERL: vertebral, anal, cardiac, tracheal, esophageal, renal, limb anomalies) [83].
Congenital thumb hypoplasia is a form of radial ray deficiency and can occur as an isolated deformity or be associated with several syndromes [84]. Associations have been most commonly seen with Holt–Oram syndrome (cardiac–limb syndrome), VACTERL complex, and less commonly with Fanconi anemia (bone marrow failure), Nager syndrome (Treacher Collins mandibulofacial dysostosis type with limb anomalies), and thrombocytopenia–absent radius syndrome. Preoperative assessment of cardiac function and a comprehensive physical evaluation of the other organ systems are required.
Congenital Pigmented Nevus
Congenital pigmented or melanocytic nevi are present at birth in approximately 1 percent of newborns and are categorized by size. They present as a dark-colored often hairy patch of skin, heterogeneous in consistency, covering any size surface area and any part of the body. Giant congenital pigmented nevi are rare lesions (<1:20 000 births) and occur most commonly on the posterior trunk, but may also appear on the head and extremities. These nevi are of special significance because of their association with leptomeningeal melanocytosis (neurocutaneous melanocytosis) and their predisposition for development into malignant melanoma. In the absence of neural melanosis, early excision and repair aided by tissue expanders, rotation flaps, or grafting may reduce the burden of nevus cells and thus the potential for development of melanoma. Surgical treatment often requires several expanders, serial tissue expansions, and reconstructive operations [85–89].
Anesthetic Considerations
If the infant presents for serial procedures, premedication should be considered. The location and size determine the frequency and anesthetic technique. If the face, neck, and head are involved, airway management needs to be discussed with the surgical team. The tissue expander is implanted under general anesthesia. The expanders are usually inflated in the outpatient setting via percutaneous access using local anesthetics at the puncture site until calculated inflation volume is achieved. Some infants might also require anesthetics/sedation for the tissue expansion process. The location of the tissue expanders and their expansion can make positioning of the infant challenging for airway management and surgical exposure. It can be managed by special head rings and positioning devices (e.g., blankets) to build up the body to the level of the head. The most common complications of tissue expansion are infection, deflation, and exposure of the expander [85]. Other indications for tissue expansion include craniofacial anomalies, aplasia cutis congenital, meningomyelocele, and giant abdominal defects [90,91].
Lymphatic Malformation
Lymphatic malformations (LMs), known in the past as “cystic hygromas,” are uncommon congenital malformations that can be categorized as macrocystic, microcystic, or combined forms. Lymphatic malformation also occurs in combination with venous anomalies. Cystic lymphatic anomalies can be diagnosed in utero by ultrasonography. Usually an LM is obvious in the newborn nursery; cervicofacial lesions can affect breathing and swallowing. Cystic LM of the midline posterior cervical region (also called posterior nuchal translucency) is often associated with chromosomal abnormalities, particularly Turner syndrome and trisomy 13, 18, and 21. Posterior nuchal translucency has also been associated with several malformative disorders, e.g., Beckwith–Wiedemann, Brachmann–de Lange, and Noonan syndromes.
The most common location of LM is the cervicofacial region, followed by the extremities and the trunk. LM can be localized to the anterior tongue, presenting as intermittent lingual swelling with dorsal vesicles and intermittent bleeding. More common is microcystic involvement throughout the tongue, with extension in the oral floor, cervical region, and sometimes upper mediastinum displacing pharynx, trachea, and esophagus. Lymphatic malformation of the lingual base and oral floor is the most difficult to manage; usually adjacent structures are involved, including the neck, mandible, face, lips, pharynx, and larynx. This location is associated with major morbidity, such as chronic airway problems, recurrent infections, and functional issues related to feeding, speech, oral hygiene, and malocclusion. Frequently tracheostomy is required during infancy [92]. Lingual LM may interfere with swallowing, requiring placement of a feeding tube or gastrostomy.
The differential diagnosis of a cystic mass in the parotid region, cheek, or neck of a newborn includes cervical teratoma, infantile myofibromatosis, infantile fibrosarcoma, or rhabdosarcoma. Some cervical and periorbital lesions appear to be a combined lymphatico-venous malformation. Periorbital LM is usually unilateral and often associated with intracranial developmental venous anomalies. A characteristic sign of orbital LM is exacerbation of exophthalmos during an upper respiratory tract infection.
In the clinical course, periodic minor variations in size of a lymphatic anomaly are common, but proportionate growth with the infant’s growth is the rule. Typically, LM expands coincident with a viral upper respiratory tract infection or intralesional bleeding. Often there is a history of bruising, recurrent inflammation, and bacterial infection (cellulitis), which can also cause a dramatic increase in size.
Anesthetic Considerations
Antenatal diagnosis of cervicofacial LM permits planning for delivery and immediate postnatal care (Figure 22.10). If prenatal ultrasonography demonstrates a severely narrowed airway, ex utero intrapartum treatment (EXIT) can be life-saving [93–96]. After Cesarean section, the patency of the neonate’s airway is assessed before clamping the umbilical cord. Intubation may be possible, but if laryngeal LM prevents insertion of the endotracheal tube, tracheostomy can be performed [97]. A cervicofacial LM is soft, allowing the neonate to breath spontaneously, whereas a large teratoma in this location is usually a firm, solid mass and more likely causes a problematic airway.
Figure 22.10 Neonate with left cervicofacial lymphatic malformation, born at 34 weeks’ gestational age. Ex utero intrapartum treatment (EXIT procedure) ensured uteroplacental gas exchange and fetal hemodynamic stability while ENT service established and secured the airway. After intubation using a Parson laryngoscope, the neonate was fully delivered and taken to the warming table and ventilated.
A newborn with cervicofacial LM can exhibit rapid enlargement of the tongue or floor of the mouth, leading to respiratory obstruction with stridor, dysphagea, and apnea. A complete examination of the airway is necessary, including fiberoptic nasopharyngoscopy and laryngoscopy to assess for potential involvement of the hypopharynx, supraglottis, and larynx. Acute airway obstruction can occur during induction and spontaneous ventilation should be maintained until the airway is secure. Fiberoptic intubation might be possible; however, a large cervicofacial LM usually necessitates tracheostomy due to involvement of the supraglottic airway and the risk of acute swelling secondary to intralesional hemorrhage and sepsis [92,98].
Treatment options for LM include sclerotherapy for macrocystic lesions, preoperative embolization to control lingual bleeding, CO2 laser and radiofrequency ablation for vesicles, and well-timed staged resection to debulk the mass [99–102]. Resection of a facial LM is difficult because the lesion permeates through the soft tissue. Monitoring of the facial nerve can be helpful and requires withholding of muscle relaxation during that phase of the procedure. Corticosteroid is given intraoperatively to reduce postoperative swelling and tapered over 2–3 postoperative days.
Postoperative complications include: airway obstruction, laryngeal edema, nerve damage, and wound infection or delayed cellulitis. After resection of a LM, a stay in the intensive care unit is required until swelling subsides. An infant undergoing resection of LM in the tongue and oral floor usually has a tracheostomy or requires prolonged postoperative intubation because the tongue has a remarkable capacity to swell and protrude in the immediate postoperative period. A serosanguineous fluid collection may have to be tapped, often repeatedly, by ultrasonographic guidance. Partially resected LM has the potential for regeneration.
Acknowledgments
The author thanks John B. Mulliken, MD, Department of Plastic and Oral Surgery, Boston Children’s Hospital, Harvard Medical School, Boston, MA, USA for discussion, editing, and photographic images; Claes G.K. Lauritzen, MD, PhD, Department of Plastic Surgery, Sahlgrenska University Hospital, Goeteborg, Sweden; Reza Rahbar, DMD, MD, FACS, Department of Otolaryngology & Communication Enhancement; and Mark R. Proctor, MD, Department of Neurosurgery, Boston Children’s Hospital, Harvard Medical School, Boston, MA, USA for providing photographs to illustrate this chapter.
Patient’s Consent
Parents or guardians provided written consent for the use of patients’ images.
References
Gastroschisis/Omphalocele
The neonate with gastroschisis presents with the abdominal viscera herniated and exposed to air after delivery; this is usually an isolated lesion. Omphalocele is associated with other anomalies of cardiac, genetic, urologic, and/or metabolic (Beckwith–Wiedermann syndrome) origin, and the viscera are covered with a sac [1]. Specific considerations are directed at maintaining perfusion of the bowel, fluid resuscitation, and careful staged reduction with maintenance of pulmonary function. Surgical closure of the abdominal contents must be performed with close monitoring of changes in pulmonary compliance in order to prevent an abdominal compartment syndrome (Figure 23.1) [2,3].
Figure 23.1 Schematic of pulmonary compliance relative to increasing intra-abdominal pressure.
Gastroschisis is a full-thickness defect in the anterior abdominal wall, usually located to the right of the umbilicus, leading to herniation of abdominal contents. The defect can be of variable size, but is generally less than 5 cm in diameter [5]. The etiology is uncertain, but various mechanisms have been proposed, including a failure of mesenchymal cells to cover the abdominal wall defect after normal return of the developing gastrointestinal tract to the embryologic abdominal cavity. Other theories include weakness in the abdominal wall leading to rupture and herniation of the rapidly growing gastrointestinal tract, defective involution of the right umbilical vein, and disruption of the right omphalomesenteric artery, leading to ischemia and atrophy or thinning of the anterior abdominal wall [6,7].
The herniated contents usually consist of small and/or large bowel, and may include solid organs such as liver if the defect is large. The gastrointestinal tract is not covered by the peritoneal sac, and is thereby fully exposed to amniotic fluid in utero and to the environment after birth. Blood supply to the abdominal viscera may be compromised, leading to ischemic or infarcted bowel. Often, the bowel may appear dilated, foreshortened and edematous, with areas of atresia from compromised blood flow in utero. The gut is functionally abnormal, even without atretic portions or other associated anomalies. A fibrinous “peel,” thought to come from gastrointestinal contents contacting amniotic fluid, may cover the exposed viscera, and is associated with a worse outcome [5,8].
The incidence of gastroschisis has been estimated to be approximately 1:3000–1:8000 live births, with recent increase in overall incidence worldwide [9,10]. One known risk factor is maternal age less than 20 years [11]. Serious associated congenital anomalies are uncommon, with most abnormalities affecting the gastrointestinal system, such as intestinal atresia [12]. Most cases are sporadic, but there has been an association with prematurity and low birth weight [13]. Complications of gastroschisis include sepsis, bowel infarction and perforation, and necrotizing enterocolitis, with patients also experiencing problems related to prematurity such as chronic lung disease and cardiac abnormalities. Gastrointestinal dysfunction may also lead to prolonged ileus, feeding delay or feeding intolerance and prolonged hospitalization [14]. Gastroschisis originally was associated with 100 percent mortality, but now with a staged closure has a 90 percent survival rate with specialist care. There is an overall good prognosis, although the risk of long-term disability associated with prematurity and gastrointestinal dysfunction exists [15]. A small cohort of over 60 neonates born with gastroschisis followed to 36 months had long-term problems with bowel motility, reflux, short gut syndrome and tube-feed or dependence on total parenteral nutrition [16].
Most cases of gastroschisis are diagnosed with prenatal ultrasound, and the diagnosis is supported with elevated maternal serum alpha-fetoprotein [17,18]. Once diagnosed, arrangements should be made for delivery at a facility capable of resuscitating the neonate with gastroschisis. The goals of resuscitation immediately after birth include airway protection, rapid assessment and support of adequate ventilation and oxygenation, protection of exposed bowel, and minimization of insensible fluid and heat losses [2]. The baby should be actively dried and warmed, with exposed bowel and the lower half of the body covered with a sterile transparent plastic bowel bag as a temporizing measure. The baby should be placed in the right lateral decubitus position to reduce the risk of compromised blood flow to the gastrointestinal tract while further assessment and resuscitation continues. A nasogastric tube for bowel decompression should be placed to reduce the risk of aspiration and regurgitation. Intravenous access should be established for targeted resuscitation with intravenous isotonic fluids and administration of broad-spectrum antibiotics. Catheterization of umbilical vessels is contraindicated in gastroschisis [1].
The high degree of third space losses with gastroschisis should not be underestimated. The neonate should be actively resuscitated with isotonic fluids, with regular assessment of volume status by exam, vital signs, urine output, and laboratory measurements of acid–base status [19]. Invasive monitoring with a peripheral arterial line should be considered. Care should be taken to keep the bowel covered with sterile plastic wrap to reduce the risk of profound hypothermia and dehydration, and the viscera should be physically supported to ensure that no twisting or impingement occurs at the herniation site leading to ischemia, particularly during times of transport [2,5].
Urgent reduction of abdominal contents is necessary due to the risk of ischemia and infection. The size of the abdominal wall defect determines surgical approach. Small defects may be managed with primary closure in the operating room or at bedside in the neonatal intensive care unit. Large defects may be managed in one of several ways, with many practitioners favoring a staged reduction using a preformed spring-loaded silo or a formal silastic silo, which is then suspended [20,21]. Gravity will allow the exposed bowel to gradually return to the abdominal cavity, with the silo concomitantly reduced in size daily. Physiologic neonatal diuresis will also lead to a decrease in bowel edema, enhancing reduction. The abdominal defect is closed in the operating room once the viscera have been completely reduced.
Preoperative preparation includes evaluation of volume status and adequacy of resuscitation, as well as screening for any comorbidities. These patients are at risk for infection, dehydration, hypothermia, acidosis, hypoproteinemia with decreased plasma oncotic pressure, and obstruction with vomiting and abdominal distention. The operating room should be warmed, with forced-air warming blankets, external heat lamps, heat-moisture exchangers and other devices to combat hypothermia [22,23]. Blood products should be cross-matched and available.
Prior to anesthetic induction, the nasogastric tube should be aspirated to drain gastric contents. A rapid-sequence or modified rapid-sequence induction is suggested after adequate preoxygenation. A difficult airway may necessitate advanced airway techniques including video laryngoscopy or fiberoptic intubation. Anesthesia may be maintained with volatile inhaled anesthetic, narcotic techniques, or supplemented with a caudal/epidural [24]. Neuraxial techniques are not contraindicated and can be particularly useful in cases of early extubation. Both ropivacaine 0.2 percent and chloroprocaine 3 percent infusions have been described and used successfully as adjuncts for major abdominal wall repair in the neonate [25]. Nitrous oxide should be avoided due to the propensity for bowel distention. Intravenous dextrose solution administration is recommended for maintenance of serum glucose, and additional intravenous isotonic fluids should be given to maintain euvolemia. A bladder catheter is often placed to monitor urine output. Standard monitors as well as arterial pressure monitoring may be necessary for larger defects. Both core and peripheral temperatures should be monitored. A central venous catheter may be placed for additional access or if total parenteral nutrition will be required postoperatively [23].
Intragastric or bladder pressures may also be monitored during primary closure of a large defect to assess for abdominal compartment syndrome [26]. Herniated viscera reduced into a relatively small abdominal cavity will raise intra-abdominal pressure, compressing the inferior vena cava, decreasing venous return and cardiac output [27]. In severe cases, this may lead to compromised renal and splanchnic circulation, leading to end-organ ischemia with resultant metabolic acidosis, renal failure, bowel perforation, and/or necrotizing enterocolitis. A tight closure may also lead to high wound tension and dehiscence, as well as compromised respiratory function, necessitating higher pulmonary inflation pressures. Peak inspiratory pressure prior to reduction should be noted to assist in evaluation of increased abdominal pressure [3,28]. Ventilation may become problematic with an endotracheal tube with a large leak after a tight reduction.
Omphalocele, or exomphalos, is a midline herniation of the gastrointestinal tract through the umbilical ring into the base of the umbilical cord, and can consist of any amount of intestine as well as liver, spleen, or other abdominal organs. The viscera are housed in a gelatinous-appearing membranous sac consisting of peritoneum, Wharton’s jelly, and amnion. The umbilical vessels themselves insert into the membrane, and the bowel is normal [7,29].
Most cases of omphalocele are sporadic. Unlike gastroschisis, the overall incidence has remained stable at approximately 1:5000 live births [30,31]. The exact mechanisms are unknown, but omphalocele is thought to result from the failure of the midgut to return to the developing abdominal cavity after the tenth week of gestation, with incomplete closure of the anterior abdominal wall at the umbilicus [32]. The size of the defect can be variable, ranging from 2–5 cm (small) in diameter to greater than 10 cm (large), with organ herniation and pulmonary hypoplasia from poorly developed abdominal and thoracic cavities. Greater than 60 percent of cases have associated congenital abnormalities, including cardiac (30–40 percent) defects such as Tetralogy of Fallot, chromosomal disorders (trisomy 13, 18, 21), cloacal or bladder extrophy, Beckwith–Wiedemann syndrome, congenital diaphragmatic hernia, malrotation of the gut, microcephaly, meningocele, and rarely, pentalogy of Cantrell (Table 23.1) [11]. Outcomes are affected by the severity of associated congenital defects, surgical complications, low birth weight, membrane rupture, bowel obstruction, and sepsis [33,34].
Table 23.1 Characteristics of omphalocele and gastroschisis
Omphalocele | Gastroschisis | |
---|---|---|
Incidence | 1:5000; stable | 1:3000 – 1:8000; increasing worldwide |
Position | Midline | Right of umbilicus |
Size of defect | Variable size, small (2–5 cm), large (>10 cm) | Full thickness; variable, usually <5 cm |
Presence of sac | Yes, although sac may rupture | No; bowel completely exposed pre- and postnatally |
Associated defects | Greater than 60 percent cases – cardiac (30 – 40 percent), i.e., Tetralogy of Fallot – chromosomal disorders, i.e., trisomy 13, 18, or 21 – urologic, i.e., bladder extrophy, cloacal defect – metabolic, i.e., Beckwith–Wiedemann – neurologic, i.e., microcephaly, meningocele – congenital diaphragmatic hernia – Pentalogy of Cantrell | Uncommon; usually isolated lesion |
As with gastroschisis, most cases of omphalocele are detected on prenatal ultrasound, and associated with elevated levels of maternal serum alpha-fetoprotein, although values are generally not as high as with gastroschisis. Once omphalocele is suspected, amniocentesis and chorionic villus sampling should be pursued for karyotype analysis to screen for chromosomal abnormalities, along with fetal echocardiogram to evaluate for congenital heart disease [18,35]. There is associated high risk for intrauterine growth restriction, preterm labor and fetal demise [11]. Babies with a large omphalocele defect should be delivered by Cesarean section to decrease the risk of sac rupture [35]. The sac may rupture prior to, during or after delivery, leaving the bowel unprotected.
The goals of resuscitation immediately after birth include airway protection, rapid assessment and support of adequate ventilation and oxygenation, protection of exposed bowel and minimization of insensible fluid and heat losses [2]. Third-space losses, however, are not as profound as with gastroschisis, and there is less immediate urgency for surgical repair unless the sac has ruptured, although the risks of ischemia, bowel obstruction and sepsis are still present. A nasogastric tube for bowel decompression should be placed. The baby may be placed in the left lateral decubitus position to reduce the risk of compression of the inferior vena cava and compromised venous return. Intravenous access should be established for targeted resuscitation with intravenous isotonic fluids, administration of broad-spectrum antibiotics and dextrose solution. Catheterization of umbilical vessels is contraindicated [36]. Infants with Beckwith–Wiedemann syndrome have macroglossia so intubation may be difficult. Intraoperatively, they can develop hypoglycemia so a means of measuring serum glucose should be available.
Reduction of abdominal contents is necessary due to the risk of ischemia and infection. As with gastroschisis, care should be taken to keep the bowel covered with sterile wrap to reduce hypothermia and dehydration, and the viscera should be physically supported to ensure that no twisting or impingement occurs at the herniation site leading to ischemia, particularly during times of transport. Given the known association between omphalocele and other congenital defects, preoperative preparation should include a thorough medical screening, including chest radiograph, echocardiogram, renal ultrasound and laboratory panel [2].
Intraoperative management concerns are similar to those for gastroschisis repair, with the size of the abdominal wall defect determining the surgical approach. Primary closure may be used for small defects. Staged repair using a silo over days to weeks, mesh closure, and tissue expanders have all been described [20,21,37]. Large unruptured, stable defects may be allowed to epithelialize using escharotic topical agents (i.e., silver sulfadiazine), with later repair of the abdominal wall defect. If the liver is herniated, hepatic vein compression or damage to the liver itself during reduction may lead to hemodynamic instability.
Both term and premature neonates are thermogenically active. In order to maintain constant temperature, infants exposed to a cold environment increase their metabolic activity and heat production without shivering. This, however, has high energy and oxygen costs. Heat loss is favored due to the neonatal body habitus of reduced subcutaneous fat and large surface area to body ratio. Neonates have limited shivering and non-shivering thermogenesis (brown fat) and are at high risk for temperature losses in the perioperative period. Survival of premature neonates is directly related to ambient temperature. Perioperative goals of care should include maintenance of a neutral thermal environment. This is facilitated by use of heated transport, warmed solutions, warmed blood products, heated mattress, radiant warmer, and forced-air warmer [23].
Umbilical Hernia
Residual patency of the umbilical ring and the omphalomesenteric duct may lead to other, less severe forms of ventral abdominal wall defects, including umbilical hernia, Meckel’s diverticulum, umbilical polyp, or fistula [38].
All neonates have an umbilical defect for the umbilical vessels and cord. The umbilical ring closes naturally during the first few weeks of life, but in 10–30 percent of patients the ring fails to close. Certain ethnic groups have a higher incidence, with African Americans 6–10 times more likely than Caucasians to present with an umbilical hernia [39]. Those with low birth weight (less than 1200 g) are four times more likely to have umbilical ring defect than those with a birth weight greater than 2500 g. Other risk factors include trisomy 21 and Beckwith–Wiedemann syndrome [40]. Umbilical hernias are covered by skin and peritoneum. Most umbilical hernias will close spontaneously in the first few years of life, depending on the size of the defect and the age of the child at presentation. Most repairs are performed after three years of age. Incarceration is uncommon at less than 5 percent of cases, with a less than 2 percent rate of recurrence [41,42].
Preoperative preparation includes an assessment of any residual comorbidities of prematurity, associated syndromes, and evidence of incarceration or obstruction. Anesthetic techniques include general anesthesia supplemented with truncal (rectus sheath, paraumbilical) blocks [43] or caudal injection of local anesthetic, and regional anesthesia such as spinal or caudal injection of local anesthetic. Umbilical hernia repairs may be scheduled as ambulatory or day surgery cases for older patients that do not require postoperative apnea monitoring [44]. Recent studies have shown superior analgesia from ultrasound-guided rectus sheath blocks compared with peri-incisional infiltration of local anesthetic [45,46].
Inguinal Hernia
Indirect inguinal hernia results in a protrusion of intestine through a congenitally patent processus vaginalis, while a direct hernia is due to a defect in the transversalis fascia. The incidence is 0.8–4 percent of the population, and is approximately 5–10 times more common in boys than girls. It is quite common in premature infants and infants of low birth weight, affecting 10–30 percent of premature infants as opposed to 3–5 percent of term infants, with an incidence of 13 percent in babies born at less than 32 weeks’ gestation, and 30 percent in babies weighing less than 1000 g. It is found more commonly on the right (75 percent) than the left side (25 percent), reflecting the later descent of the right testicle in development. Bilateral hernias occur in 15–20 percent of cases, and risk factors for bilateral hernias include female gender, presentation with a left-sided hernia, prematurity, age less than one year and undescended testicle [47,48].
During development, the gonads descend from the urogenital ridge in the upper abdomen to the inguinal ring at three months’ gestation. The processus vaginalis develops from the peritoneal lining. At 6–7 months’ gestation, the testes descend through the inguinal canal, following the gubernaculum, and come to lie in the scrotum. The processus vaginalis is gradually obliterated, leaving behind the tunica vaginalis, with closure occurring earlier on the left than the right side during development [49]. There is greater likelihood of incomplete obliteration with decreasing age, and higher chance of indirect inguinal hernia formation. In females, the round ligament is analogous to the processus vaginalis, and inguinal hernias occur frequently at the canal of Nuck, anterior to the round ligament.
Hernias may be asymptomatic or minimally symptomatic, with intermittent presentation of a bulge at the internal or external inguinal ring. The bulge can disappear with rest or sleep, and may reappear with Valsalva maneuvers such as crying or straining. The differential diagnosis includes hydrocele, lymphadenopathy, neoplasm, torsion, and retractable testis. Ultrasound may reveal a patent processus vaginalis.
An easily reducible, asymptomatic hernia may be repaired electively. An incarcerated hernia is one that is not reducible, and may be associated with pain, tenderness, abdominal distention, emesis, and anorexia. Attempts should be made to reduce an incarcerated hernia at presentation, which may require the use of pain medication, sedation, or repositioning, with subsequent repair after reduction in 1–2 days to prevent recurrence of incarceration. If the hernia is irreducible or is causing obstruction, it should be repaired urgently due to the risk of strangulation. A strangulated hernia is an incarcerated hernia with compromised blood flow to the bowel or testes, and is a surgical emergency. Presentation includes emesis, pain, fever, leukocytosis, erythema, tachycardia, and history of a prolonged bulge. The patient may require a bowel resection for ischemic or infarcted bowel. Premature infants and those less than six months old are more likely to have incarceration [50,51].
Timing of inguinal hernia repair depends upon the age of the patient and existing comorbidities. The risk of incarceration is balanced with the risk of undergoing surgery, particularly amid evolving concerns about anesthetic neurotoxicity in the young and premature [52]. Factors affecting postoperative apnea and respiratory complications include young postconceptual age, existing lung disease, oxygen requirement, anemia, home apnea monitoring, and administration of sedatives, general anesthetics, and opioids [53]. The risk for postoperative apnea decreases with increasing postconceptual age, and many centers have in place institutional policies requiring postoperative admission for apnea monitoring for those patients whose postconceptual age is less than 52–60 weeks in the former premature infant and less than 45 weeks in the former term infant [44,54]. Hernia repair is often scheduled prior to planned discharge home for the former premature infant, or alternatively, the patient may be discharged home and scheduled to return for elective repair at a later time [55].
Inguinal hernia repair may be performed either open or laparoscopically, and opinion is divided regarding superiority of one approach over the other [56]. Surgical complications include infection, bleeding, infarction, and atrophy of the testis, injury to the vas deferens, bowel perforation, and hernia recurrence (less than 5 percent). Some practitioners will perform laparoscopic evaluation of the contralateral side for patent processus vaginalis and hernia, but whether to do so routinely remains controversial [57]. Fifty percent of children less than two years old will have a patent processus vaginalis on the contralateral side, but it is unclear how many of them will eventually present with hernia, with variable reported rates of 8–20 percent [50,58].
Preoperative preparation includes an assessment of residual comorbidities of prematurity, the nature of the hernia, and the planned surgical approach [53]. Administration of caffeine 10 mg kg–1 IV may be considered for those patients at risk of postoperative apnea [59]. Anesthetic techniques include general anesthesia supplemented with truncal (ilioinguinal, iliohypogastric, transversus abdominis plane) blocks [60,61] or caudal injection of local anesthetic, and regional anesthesia such as spinal or caudal injection of local anesthetic with or without placement of a caudal catheter [62]. The advantage of a spinal anesthetic is its quick onset, and the benefit of avoiding the effects of systemic sedation and postoperative apnea, but drawbacks include limited duration of action and variable reported failure rates [63]. Supplemental sedatives or opioids will eliminate the advantages of awake regional anesthesia on postoperative apnea [44]. Intraoperative restlessness can be soothed with a pacifier dipped in sucrose solution [64]. Caudal anesthesia may be more reliable but has a slower onset [65]. Large, bilateral, and complicated repairs may necessitate general anesthesia. Laparoscopic evaluation of the contralateral side for hernia is not an absolute contraindication for awake regional anesthesia, but can pose specific challenges during insufflation and pneumoperitoneum [63].
Hydrocele
Hydrocele is a fluid collection in the tunica vaginalis around the testicle. A communicating hydrocele is essentially a hernia, with a connection to the abdominal cavity via a patent processus vaginalis, whereas a noncommunicating hydrocele does not maintain such a connection [66].
During development, incomplete obliteration of the processus vaginalis will lead to either hernia or hydrocele. An open processus vaginalis with complete failure of obliteration leads to an inguinal or scrotal hernia. Distal obliteration of the processus vaginalis with a patent proximal portion will lead to inguinal hernia. Narrowing of the proximal processus creates a communicating hydrocele, and partial patency of the processus with obliteration of the proximal portion leads to a noncommunicating hydrocele [47].
Communicating hydroceles fluctuate in size during the day, enlarging especially in the dependent position, and are easily compressible. They are treated like inguinal hernias and generally require surgical repair [67]. Noncommunicating hydroceles do not fluctuate in size daily, but can gradually change in size over the course of weeks and are non-compressible. They are quite common after birth, with most resolving spontaneously by the first two years of life. If they have not resolved in the first 12–24 months of life, noncommunicating hydroceles should undergo surgical repair [50,68].
Like hernias, hydroceles are more common on the right than the left side. Those found at birth do not increase the chance of hernia later, although hydroceles that develop later in life imply the presence of a patent processus vaginalis with a chance of hernia formation. Repair is via an inguinal approach to evaluate for a patent processus [69]. A high ligation is performed, the distal sac is opened and the hydrocele is evacuated but is not completely excised due to potential risk of injury to the testicle.
Preoperative preparation includes screening for any residual comorbidities of prematurity or from the neonatal period, and the type of hydrocele and the surgical approach. Older infants and toddlers may benefit from premedication with an anxiolytic prior to parental separation and induction [70]. Anesthetic techniques include general anesthesia with or without caudal injection of a local anesthetic or ilioinguinal and iliohypogastric nerve blocks [71]. Either inhalational or intravenous induction may be performed. Regional anesthesia options include caudal or spinal anesthesia.
References
Introduction
Abdominal procedures constitute a large percentage of operating room cases in the neonatal and infant population. Therefore, it is vital for anesthesiologists caring for pediatric patients to be knowledgeable regarding general surgical conditions common to this specific patient population, as well as their typical presentation, associated comorbidities and perioperative management strategies. Patients presenting for surgery often have associated syndromes or genetic abnormalities that predispose them to abdominal complications, and the anesthesiologist should be cognizant of the other associated systemic manifestations of the underlying disease.
Foremost among the considerations in evaluating patients presenting for intra-abdominal surgery is the potential for either altered or frankly obstructed gastrointestinal emptying. In children, there are currently no noninvasive methods to reliably discriminate patients with full stomachs from those with relatively intact gastric emptying. Therefore, common practice is to assume that the patient is at high risk for aspiration during induction and emergence, unless actively tolerating oral feeding without complications. Rapid sequence induction should be considered, though with this approach neonates and infants may develop hypoxemia during the time period needed for adequate muscle relaxation due to their higher baseline oxygen consumption (>5 ml kg–1 min–1 vs. 2–3 ml kg–1 min–1 in adults) and decreased apneic functional residual capacity compared with adults [1,2]. Due to these considerations, many practitioners choose to utilize a modified rapid sequence induction with cricoid pressure and low-pressure mask ventilation prior to intubation. Though use of a modified rapid sequence technique can mitigate risk of hypoxia during induction, application of cricoid pressure can potentially obscure airway anatomy and hinder mask ventilation. Despite these precautions, judicious mask ventilation with pressures as low as 10 cmH2O has still been shown to result in gastric insufflation, though applying cricoid pressure can increase this threshold [3–5]. Additionally, use of a nasogastric tube, placed prior to induction, can be used to decompress the stomach. The potential benefit of this maneuver must be weighed against the possibility that the stomach may still not be fully empty and the possibility of additional patient agitation. Though studies in infant and adult cadavers demonstrated effective esophageal occlusion with cricoid pressure of up to 100 cmH2O in the presence of a nasogastric tube, concerns with leaving a nasogastric tube in place during induction include potentially interfering with esophageal sphincter function and serving as a “wick” for regurgitant contents [6,7]. Consequently, if a nasogastric tube is placed for gastric evacuation, whether it should be removed prior to induction is not clear.
In addition to addressing the direct anatomical and surgical concerns of intra-abdominal injury, the pediatric anesthesiologist must be acutely aware of and thoroughly investigate the potential systemic manifestations of abdominal pathology. Patients will often have signs consistent with sepsis and capillary leak syndrome pre-, post-, and intraoperatively secondary to underlying bowel ischemia or perforation. These patients will often require aggressive fluid resuscitation and will occasionally require the use of vasoactive medications when vasoplegia is present. Perioperative management can also be complicated by florid intra-abdominal inflammation and fluid retention, leading to abdominal compartment syndrome. Depending on the degree of abdominal swelling and concerns for postoperative abdominal compartment syndrome, abdominal closure may not be immediately possible or advisable at the end of the procedure. Airway edema secondary to rapid fluid administration, ongoing hemodynamic instability, and unfavorable acid–base status may preclude safe extubation and result in subsequent difficulty with ventilation. These concerns coupled with increased relative evaporative losses in the neonate with an open abdomen can make these cases particularly challenging from a fluid management perspective. Regional anesthetics for postoperative analgesia such as epidural, caudal, and transversus abdominis plane catheters can be considered, but expected duration of postoperative ventilation, future and present coagulation status, and risk of postoperative infection should be considered prior to placement.
Increasingly, many intra-abdominal procedures are being performed laparoscopically, and understanding the physiological changes that occur with abdominal insufflation is important. Carbon dioxide is the preferred gas for laparoscopy due to the fact that it is not readily flammable, clears quickly from the peritoneum, and does not expand within closed spaces. Carbon dioxide is readily absorbed across the peritoneum and necessitates an increase in minute ventilation to maintain normocapnia. The insufflated abdomen can lead to cephalad displacement of the diaphragmatic, to compression of dependent lung areas and to subsequent V/Q mismatch and hypoxemia. Higher airway driving pressures may be needed to compensate for reduced pulmonary and thoracic compliance with laparoscopy [8]. Hemodynamically, higher insufflation pressures can lead to reduction in cardiac output [9,10]. If insufflation pressures exceed venous pressure in the setting of open abdominal venous channels, clinically significant carbon dioxide embolism can occur [11]. Intracranial pressure can be increased by a combination of increased abdominal pressure, hypercarbia, and head-down positioning, and therefore the risks and benefits of laparoscopy should be carefully considered in patients with elevated intracranial pressure or an existing ventriculoperitoneal shunt [12,13].
Specific Conditions
Gastrointestinal Duplications
Gastrointestinal duplications are rare congenital lesions that occur in approximately 1 in 4500 live births [14]. Duplications have been described at all points along the gastrointestinal tract as proximally as the mouth and as distal as the anus. The etiology of gastrointestinal duplications is unclear, but they are thought to occur between four and eight weeks during embryonic development. Existing theories to explain their occurrence include errors in recanalization of the primitive intestine, failure of regression of embryonic diverticuli, and errors of notochord splitting [15,16]. Gastrointestinal duplications can either occur as an isolated occurrence or involve multiple locations. Duplications generally do not communicate with adjacent gastrointestinal structures, though this is not universally the case. Similar to the case of many congenital malformations, there are often associated systemic abnormalities that should be identified and evaluated [17,18].
With the advent of and improvements in prenatal imaging, most gastrointestinal duplications are detected in utero and have in only rare instances required fetal intervention. Most pediatric surgeons advocate resection of the duplication, though for asymptomatic patients the optimal timing of surgery remains unclear, and presently there are no concrete data to aid in guiding practice [14]. Patients who develop sequelae of duplications usually do so in the context of secretions that accumulate in noncommunicating and closed space duplications, leading to pain and compression of adjacent structures. Such developments are of particular concern when the compression of adjacent vasculature leads to bowel ischemia. The duplication can also serve as a lead point for the bowel to telescope around surrounding intestinal segments, leading to intussusception. Ulcerations resulting in bleeding can occur in the duplication itself secondary to ectopic gastric tissue or in adjacent tissue [19].
Anesthetic considerations for gastrointestinal duplications include localization of the duplication, identification of any associated abnormalities, and the clinical manifestations of the duplication. Potential for airway obstruction may require the use of rigid bronchoscopy, and bowel obstruction will mandate full stomach precautions.
Pyloric Stenosis
Pyloric stenosis is a relatively common condition requiring surgical intervention in infants and occurring in approximately 2–5 out of 1000 live births [20]. The etiology of pyloric stenosis is not entirely clear, though likely multifactorial. The condition occurs four times more commonly in males than in females and has a higher prevalence in first-born children [21]. Unlike many other gastrointestinal abnormalities, patients with pyloric stenosis are otherwise generally healthy, though a familial genetic predisposition is observed.
The pathological finding in pyloric stenosis is hypertrophy of the muscularis layer of the pyloric sphincter, leading to gastric outlet obstruction. Patients typically present with immediate, nonbilious, nonbloody, postprandial projectile vomiting at the age of 3–12 weeks [22] that often increases with intensity over time. The severity of dehydration and electrolyte abnormalities generally correlates with the degree and duration of symptoms prior to presentation [23]. In general, patients will present with a chloride-sensitive, hypochloremic, hypokalemic, metabolic alkalosis with some degree of hyponatremia secondary to volume depletion and paradoxical aciduria. However, if there is longstanding volume loss without correction, patients can present with metabolic acidosis as a result of both lactic acidosis and renal dysfunction.
Pyloric stenosis can often be diagnosed by history alone, but supporting physical exam findings include an olive-shaped mass in the right upper quadrant. Diagnosis is typically confirmed with an abdominal ultrasound, though barium studies have also been utilized [24].
Pyloric stenosis is not a surgical emergency, though definitive treatment is surgical pyloromyotomy, which can often be performed laparoscopically. Prior to surgical intervention, acid–base status and electrolyte abnormalities should be corrected. Patients with persistent or untreated metabolic alkalosis are at increased risk of postoperative apnea [25]. Metabolic alkalosis is often corrected after hospital admission and preoperatively with saline administration that corrects volume status, replenishes total-body sodium and chloride, and promotes excretion of urine bicarbonate. Secondary to dehydration, patients may present with renal insufficiency, and potassium repletion should be initiated only with the establishment of urine output. Serum chloride concentration can be followed to gauge the adequacy of rehydration and correction of the underlying metabolic alkalosis. Studies by Goh et al. and Shanbhogue et al. demonstrated that a serum chloride concentration greater than 106 meq L–1 predicts correction of alkalosis in the majority of patients with pyloric stenosis [26,27].
Due to functional gastric outlet obstruction, patients with pyloric stenosis are at high risk for aspiration during induction. The stomach should be emptied with a large-bore orogastric tube, sometimes with several passes, prior to induction. This does not necessarily guarantee an empty stomach. The airway should be secured by means of a (modified or standard) rapid sequence induction or awake intubation. Anesthesia can be maintained with nondepolarizing neuromuscular blockade and volatile anesthetics, though Davis et al. showed decreased postoperative respiratory events with the use of a remifentanil-based anesthetic [28]. For longer cases, a dextrose infusion should be started. Surgeons may ask that an orogastric tube be used to insufflate the stomach to rule-out luminal perforation following repair. Adequate analgesia can generally be obtained with rectal or intravenous acetaminophen combined with local anesthetic via caudal block or local infiltration by the surgeon. Sparing use of intermediate and long-acting opioids is advocated. The patient should be awake and vigorous at the end of the case prior to extubation. In addition to remaining metabolic alkalosis, hypothermia, hypoglycemia, residual neuromuscular blockade, and previous opioid administration can all further increase the risk of postoperative apnea, and every attempt should be made to avoid these conditions [21,29].
Necrotizing Enterocolitis
Necrotizing enterocolitis (NEC) is characterized by ischemic necrosis of the intestinal mucosa, leading to gut translocation of enteric organisms and to potential local and systemic complications. Necrotizing enterocolitis occurs in approximately 1 out of 1000 live births, is inversely related to birth weight and rarely occurs spontaneously in full-term neonates unless associated with predisposing conditions, such as congenital heart disease, sepsis, or hypotension, that lead to compromise of intestinal blood flow [30,31]. Mortality from NEC is 15–30 percent, and survivors often suffer from long-term sequelae such as short-gut syndrome, parenteral nutrition dependence, and growth and neurodevelopment deficits [30,32].
Necrotizing enterocolitis classically develops in the second to third week of life after the initiation of feeding, though it can develop in patients prior to initiation of feeding and has been rarely observed in patients who have tolerated feeding for a significant length of time. NEC often initially presents with constitutional symptoms such as lethargy, temperature instability, hypotension, and apnea in conjunction with or followed by gastrointestinal symptoms such as feeding intolerance, emesis, abdominal distention, or bloody stool. As the disease progresses, worsening abdominal distention, increased abdominal tympany, palpable bowel loops, and periumbilical abdominal wall erythema and ecchymosis can develop. Imaging studies can help to confirm diagnosis, although NEC is a clinical diagnosis and radiographic findings are not always appreciated. Findings that are highly suspicious for NEC include pneumatosis intestinalis, pneumoperitoneum, and evidence of air within the portal system. Patients may concurrently experience deterioration of other organ systems by avenues such as hematologic abnormalities (disseminated intravascular coagulation, thrombocytopenia, coagulopathy), metabolic acidosis, respiratory failure, and septic shock.
Initial management includes supportive measures such as bowel rest, nasogastric tube decompression, broad-spectrum antibiotics, and hemodynamic and respiratory support if necessary. Medical management is continued with serial monitoring of abdominal exam and radiographic studies. If there is high suspicion or frank evidence of intestinal perforation, surgical intervention is indicated and includes laparotomy with resection of affected bowel segments and/or bedside peritoneal drainage. Between 20 and 40 percent of NEC cases require surgical intervention [31,33], with Guthrie et al. showing a mortality rate of 23 percent for surgical NEC patients compared with 4 percent for medically managed NEC patients [33].
Patients who present requiring surgical treatment will often have multi-organ dysfunction that will complicate perioperative management. Existing hematologic abnormalities may require ongoing treatment with appropriate blood component therapy. Hypotension secondary to intravascular volume depletion and septic vasoplegia is common in the presence of intra-abdominal fluid sequestration and distributive shock. Consequently, the patient may require additional or initiation of vasoactive infusions and continued fluid administration to maintain blood pressure. A primarily narcotic-based anesthetic may be better tolerated from a hemodynamic perspective than one that utilizes higher concentrations of volatile anesthetics. In addition, delivery of volatile agents may be limited if an ICU ventilator is utilized for transport and better control and monitoring of respiratory parameters. If the airway is not already secured, consideration should be given to a rapid sequence induction or to an awake intubation. Venous access should take into account the likely need for administration of large amounts of volume and vasopressor therapy. Obtaining arterial access for hemodynamic monitoring and blood sampling is optimal but should not unduly delay definitive surgical treatment in a clinically deteriorating patient.
Meconium Ileus
Meconium ileus (MI) is characterized by bowel obstruction, generally at the level of the terminal ileum and ileocecal valve secondary to accumulation and failure of passage of thick, desiccated meconium. Patients with cystic fibrosis (CF) in particular have a propensity to develop MI due to secretion of highly viscous meconium that adheres to the intestinal lumen. Twenty percent of CF patients who develop MI represent 80–90 percent of the total MI cases [34,35]. Meconium ileus is categorized as simple or complex. Simple MI presents clinically with abdominal distention, failure to pass meconium and, in some cases, emesis. Roughly 50 percent of cases of MI are classified as complex. Meconium ileus is classified as complex if there is associated gastrointestinal pathology such as perforation, peritonitis, atresia, or volvulus. Volvulus and other ischemic insults that occur in utero may manifest postnatally as bowel atresia. Simple MI is treated with nasogastric decompression, correction of any fluid or electrolyte abnormalities, and administration of serial hyperosmolar enemas [36]. Repeat enema administration is common but increases the risk of perforation and other complications with each additional attempt [37,38]. Simple MI that is refractory to more conservative medical management requires surgical intervention.
Preparation for the operating room should identify and initiate correction of electrolyte abnormalities and volume depletion as a result of potential dehydration, hyperosmolar enema therapy, or fluid shifts associated with bowel injury or obstruction. In CF patients, the lung pathology is generally not a concern until after several years of life [39], unless the clinical presentation is associated with previous aspiration or secondary lung injury due to sepsis or systemic inflammatory response syndrome. Stomach contents should be decompressed with an orogastric tube prior to induction to minimize the risk of aspiration during anesthetic induction. Rapid sequence induction or awake intubation with local anesthetic should be employed to secure the airway. Anesthetic can be maintained with neuromuscular blockade and, depending on hemodynamic status, with volatile versus an opioid-based anesthetic. Vasoactive infusions should be readily available to treat potential hypotension.
Malrotation
Malrotation results from in utero arrest of normal rotation of the embryonic gut, leading to narrowing of the mesenteric base and to a predisposition to mesenteric twisting and consequent volvulus. With malrotation, there can also be abnormal attachments, known as Ladd’s bands, that form in the process of attempting to affix the bowel in the peritoneal cavity and that can lead to duodenal obstruction. Symptomatic malrotation occurs in approximately 1 in 5000 live births [40], and 50–70 percent of cases are associated with other congenital abnormalities. Gastrointestinal anomalies are most common, though cardiac, orthopedic, and central nervous system anomalies also frequently occur [41,42]. Intestinal atresia can be seen in cases in which malrotation occurs in utero during bowel development. In children with malrotation requiring surgery, up to 65 percent present during the first month of life [42].
Patients with malrotation can clinically present with either volvulus or nonischemic intestinal obstruction due to Ladd’s bands or atretic bowel. Patients with volvulus present with bilious emesis, symptoms of bowel obstruction, and potentially an acute abdomen. Grossly bloody stool can also be seen and usually indicates a more severe presentation with significant bowel ischemia. Diagnosis of malrotation in a clinically stable patient can be made radiographically with plain film or fluoroscopic upper gastrointestinal series with small-bowel follow-through or an abdominal ultrasound to determine the relative orientation of mesenteric vessels [43].
A patient with suspected or confirmed volvulus requires prompt surgical treatment to salvage affected bowel segments. Because volvulus is a surgical emergency, every effort should be made to safely proceed as quickly as possible to surgery with the goal of saving any viable bowel. The airway should be secured using full-stomach precautions. Arterial access can be obtained as needed, though doing so should not delay surgery and relief of ischemic bowel. As with many other ischemic abdominal pathologies, patients may present with a combination of intravascular depletion due to intra-abdominal inflammation and swelling along with vasodilation from sepsis. Treatment for hypotension may therefore require both fluid and blood component therapy in addition to vasoactive infusions. In cases in which abdominal compartment syndrome is present, concern should be exercised upon surgical decompression of the peritoneal cavity. With the relief of elevated abdominal pressures, subsequent systemic washout of lactate and products of cell ischemia, such as potassium, can result in rapid clinical deterioration.
Intestinal Atresia
Intestinal atresia is the complete congenital obstruction of the lumen of a hollow viscus. Intestinal atresia can occur at any point of the intestinal tract, with the small intestine being the most common site of pathology. Of the cases of small-bowel atresia, the majority occur within the jejunum and ileum. The atresia can also be present in the large bowel, though these cases constitute only 7–10 percent of all cases of intestinal atresia [44].
Intestinal atresia is classified into types I–IV using the following definitions:
Type I: Intact muscularis and serosa, with the lumen obstructed by an intact diaphragm or membrane.
Type II: Obvious gap in bowel with the proximal and distal segments connected by a fibrous band.
Type III:
a. Obvious gap in bowel with no connection between proximal and distal segments.
b. Proximal small-bowel atresia, absence of mid-small bowel that would normally be supplied by the superior mesenteric artery and a large gap in small-bowel mesentery.
Type IV: Multiple II or IIIA atresias.
In many cases, intestinal atresia can be diagnosed prenatally and is often accompanied by polyhydraminios. Patients with intestinal atresia present with abdominal distention, emesis, and failure to pass meconium. With bowel obstruction, there can be increased enterohepatic circulation of bilirubin and resultant jaundice. Patients with partial obstruction or stenosis will often present later than those with complete intestinal atresia. Diagnosis should exclude other intra-abdominal pathologies, and additional radiographic studies can help to confirm the diagnosis, particularly plain film or fluoroscopy with and without contrast imaging.
Patients with duodenal atresia should be screened for other associated abnormalities, with particular attention to the heart, kidneys, spine, and hepatobiliary system. There are associated chromosomal abnormalities in approximately 30 percent of cases of duodenal atresia, most often trisomy 21 [45]. Though the majority of patients with jejunal and ileal atresia are otherwise healthy, it can be associated with coexisting disease such as CF, gastroschisis, and, less commonly, omphalocele [44]. Patients with CF are predisposed to develop in utero bowel ischemia due to volvulus or perforation; the resultant necrotic bowel is resorbed, leaving behind an atretic segment of gut. Patients with colonic atresia are generally healthy, though they can also be affected with pathology such as gastroschisis and Hirschsprung’s disease (HD) [44].
Initial management of intestinal atresia involves discontinuation of feeding, decompression with a nasogastric tube, and correction of fluid and electrolyte abnormalities. Patients with duodenal atresia should be evaluated for possible comorbidities, while those with jejunal or ileal atresia should be tested for CF.
Emergent surgery for intestinal atresia is generally not indicated, and clinical stability should be established and further evaluation of potential associated abnormalities should be completed prior to bringing the patient to the operating room. Patients with intestinal atresia by definition have a full stomach, and appropriate measures should be taken during anesthetic induction to minimize risk of aspiration. Adequate peripheral intravenous access should be obtained and an arterial line placed if needed in the setting of associated cardiac abnormalities.
Hirschsprung’s Disease and Anorectal Malformations
Hirschsprung’s disease results from failure of neural crest cells to migrate during intestinal development, leading to a segment of aganglionic colon and, in approximately 7–10 percent of cases, to complete involvement of the colon, resulting in depressed gut motility [46,47]. In rare cases of HD, the entire colon and a portion of the small bowel may also be affected. Males are disproportionately affected, with a male:female gender ratio of 3–4:1 cases [48]. Multiple specific genetic mutations are associated with HD. Furthermore, HD is often associated with chromosomal abnormalities and monogenic syndromes [49].
The majority of cases of HD are diagnosed in the neonatal period when affected individuals develop symptoms of distal intestinal obstruction such as vomiting, abdominal distention, and the failure to pass meconium and stool within the first 48 hours of life. Older patients typically present with symptoms of chronic constipation, failure to thrive, and abdominal distention. Hirschsprung’s disease patients can also present acutely with enterocolitis, toxic megacolon, and associated sepsis, fever, and abdominal distention [50]. Rarely, volvulus can also occur in patients with HD due to twisting of feces or meconium-filled, enlarged bowel segments.
Patients with enterocolitis may require immediate surgical intervention, though it is preferable to stabilize the patient and obtain a definitive diagnosis of HD prior to surgery and then proceed with a single-stage definitive surgery rather than with a diverting colostomy and the need for a later pull-through procedure. Stable patients with suspected HD should undergo an appropriate diagnostic workup consisting of a rectal suction biopsy, though abdominal radiographs, contrast enemas, and anorectal manometry have all been used to provide supportive diagnostic information. Definitive treatment for HD involves surgical resection of affected bowel segments with an anal sphincter-preserving re-anastomosis of normal ganglionic bowel. Even after definitive surgical treatment for HD, patients can present later with enterocolitis [39,49].
Patients with HD presenting for surgery should be carefully evaluated for comorbid disease and syndromes that could potentially impact anesthetic management. Anesthetic induction should take into account the degree of abdominal obstruction. Patients with HD enterocolitis may require aggressive fluid resuscitation, vasoconstrictive drug infusions such as dopamine, and arterial access for monitoring of hemodynamics and metabolic status. Pull-through procedures may require lithotomy positioning, which can limit placement of intravenous catheters to the upper extremities and in rare cases has been associated with complications such as lower extremity compartment syndrome.
A similar surgical condition that the pediatric anesthesiologist may need to treat is anorectal malformations. Anorectal anomalies manifest with a wide spectrum of severity and have an incidence rate of 1 in 2500–4000 live births [51,52]. These anomalies arise from failure of normal development of the cloaca into the urogenital and rectal structures [53]. Consequently, the correction of these congenital defects will often require a multidisciplinary surgical approach.
Presurgical planning is centered on definitively defining the anatomy and identifying associated defects that may require more immediate treatment. Pre-procedure studies should include evaluation of the heart, kidney, urinary collection system, spine, and remainder of the GI tract [54]. Surgical approach will be dependent on the nature of the anomaly and on whether it is amenable to repair via a simple anoplasty, pull-through, or temporizing staged colostomy. Given the often elective nature of these procedures, anesthetic concerns similar to those entertained in HD should be considered. After corrective surgery, postoperative course often requires serial dilations that may also require the services of an anesthesiologist.
Intussuseption
Intussuseption is the invagination of one segment of intestine into another, leading to compromised lymphatic and venous drainage. Eighty-five percent of patients with intussuseption are under three years of age [55] and are generally otherwise healthy. Intussuseption is the most common cause of intestinal obstruction in the pediatric population. The site of intussuseption is often in close proximity to the ileocecal junction, though bowel segments in both the large and small intestines can be affected. If left untreated, intussuseption results in progressive bowel edema, ischemia, and possible perforation. Though the majority of cases of intussuseption have no clear etiology, commonly associated predispositions include viral illness, viral or bacterial enteritis, and identifiable “lead points” for intussuseption such as Meckel’s diverticulum, lymphoma, duplication cysts, inflamed Peyer’s patches with concurrent Henoch–Schonlein purpura, and vascular malformations [39].
Patients with intussuseption present with sudden intermittent cramps, severe paraoxysmal colicky abdominal pain, and progressive lethargy often out of proportion to abdominal exam. The classic presentation of pain, a palpable sausage-shaped mass, and currant jelly stool is observed only in a minority of patients [56]. Abdominal plain film can be suggestive of intussuseption, but abdominal ultrasound is generally the preferred modality of diagnosis [56], though computed tomography (CT) can be helpful if prior workup has been unrevealing.
Surgical intervention is indicated for patients with evidence of perforation. Otherwise, patients should be stabilized with intravenous fluids and decompression with a nasogastric tube and reduction attempted with hydrostatic or pneumatic enema. Nonoperative reduction has a success rate of 80–95 percent and can be repeated, but does carry a 1 percent risk of perforation [57] and a recurrence rate of 5–7 percent [58]. Surgical intervention is necessary if nonoperative intervention is not successful or results in perforation.
Anesthesiologists may be asked to provide anesthesia or sedation during nonoperative or surgical reduction. Patient with intussuseption should be considered to have full stomachs, and appropriate precautions should be taken during anesthetic induction. Similar to considerations for other intra-abdominal surgical emergencies, intravenous access, hemodynamic monitoring, and need for vasoactive medications and fluid resuscitation will be contingent on the severity of clinical presentation.
Biliary Atresia
Infants born with biliary atresia (BA) are most often treated with a hepatoportoenterostomy or Kasai procedure that allows for bile drainage from small bile ducts into the small intestine. There are three common types of BA: type 1 – atresia restricted to the common bile duct; type 2 – atresia of the common hepatic duct; type 3 – atresia of the right and left hepatic duct. If the Kasai procedure is performed in the first two months of life, greater than 80 percent of infants will achieve some bile drainage, although a majority of these eventually will require a liver transplant.
A hepatoportoenterostomy or Kasai portoenterostomy is a surgical treatment performed on infants with biliary atresia to allow for bile drainage. In these infants, the bile is not able to drain normally from the small bile ducts within the liver into the larger bile ducts that connect to the gall bladder and small intestine.
The surgery involves exposing the porta hepatis (the area of the liver from which bile should drain) and attaching part of the small intestine to the exposed liver surface. The rationale for this approach is that minute residual bile duct remnants may be present in the fibrous tissue of the porta hepatis and thus provide direct connection with the intrahepatic ductule system to allow bile drainage [1].
Prognosis
If performed before 60 days of age, 80 percent of children achieve some bile drainage.
Prognosis is progressively worse the later surgery is done.
Postoperatively, cholangitis and malabsorption are common.
Many children with biliary atresia will require liver transplantation despite the attempted surgical repair.
In type I, atresia is limited to the common bile duct, and the gallbladder and hepatic ducts are patent (i.e., “distal” BA). In type II, atresia affects the hepatic duct, but the proximal intrahepatic ducts are patent (i.e., “proximal” BA). Type II is subgrouped in type IIa, where a patent gallbladder and patent common bile duct are present (sometimes with a cyst in the hilum, i.e., “cystic BA”), and in type IIb, where the gallbladder as well as the cystic duct and common bile duct are also obliterated. In type III, there is discontinuity of not only the right and left intrahepatic hepatic ducts, but also of the entire extrahepatic biliary tree (i.e., “complete” BA). The French classification is similar, but the designation of the above types IIa and IIb as types 2 and 3 results in a total of four types [3].
Most often, BA is complete (Japanese/Anglo-Saxon type III, 73 percent) or subcomplete (type IIb, 18 percent), with “cystic” BA and “distal” BA being infrequent (types IIa and I, 6 percent and 3 percent, respectively) [4].
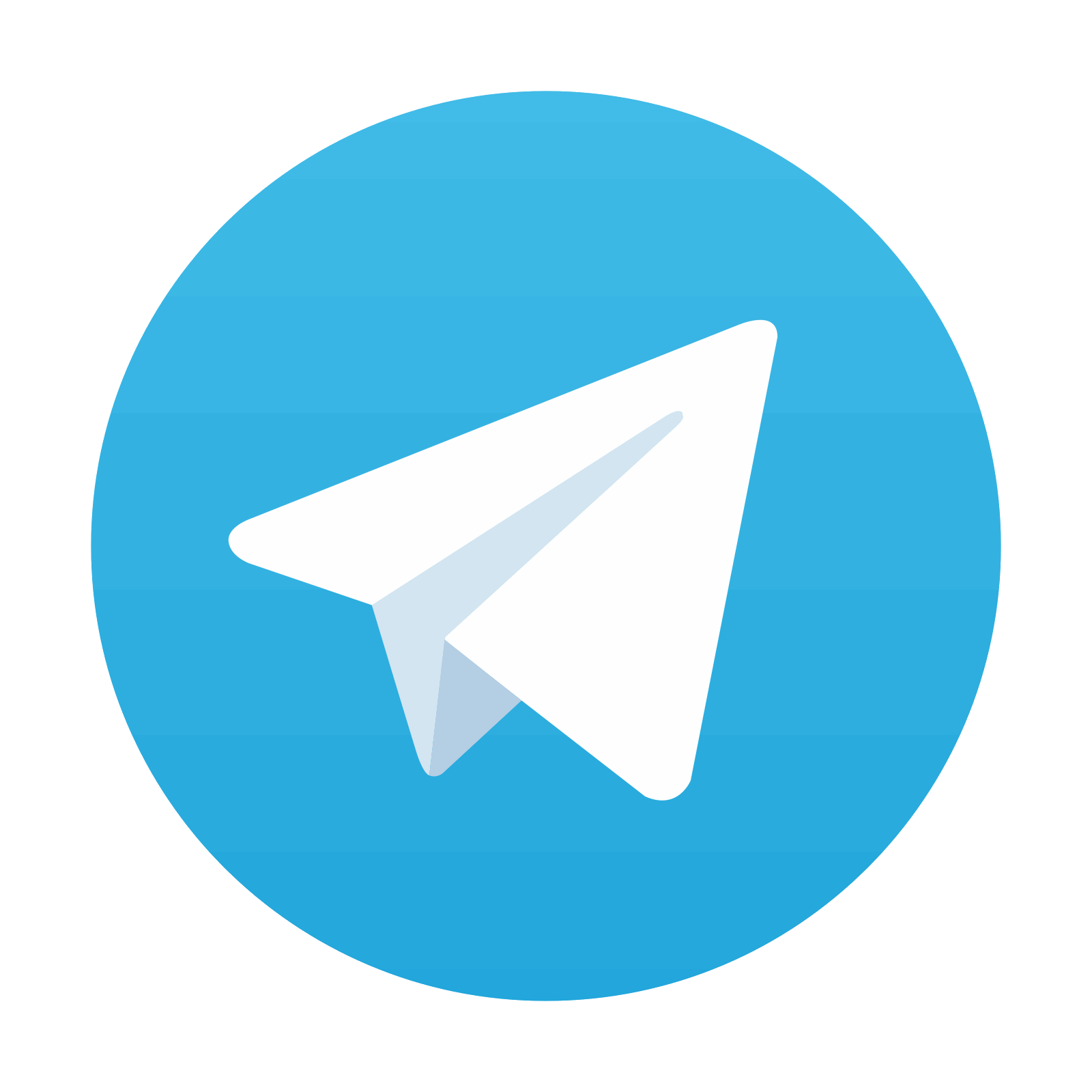
Stay updated, free articles. Join our Telegram channel

Full access? Get Clinical Tree
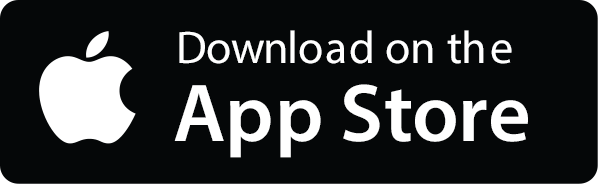
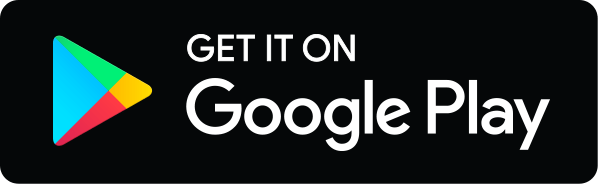
