Since the lungs have a tendency to recoil inwards, inflation requires an increase in transpulmonary pressure (TPP), generated by the respiratory muscles. As TPP increases, lung volume naturally increases. At relatively low lung volumes, the lungs are highly distensible and for a given change in TPP, this results in a relatively large increase in lung volume. However, at high volumes, lungs reach the limit of their distensibility; here there is little change in lung volume for an equivalent change in TPP. Approaching functional residual capacity (FRC), lung compliance is greatest and has a value of approximately 200 ml/cmH2O.
What factors affect compliance?
Compliance is reduced by pulmonary oedema; pulmonary fibrosis; extremes of lung volume; restrictive diseases of the chest wall; pregnancy and supine posture. It is increased by old age and emphysema.
This is the resistance of the respiratory tract to gas flow during the respiratory cycle. During laminar gas flow:

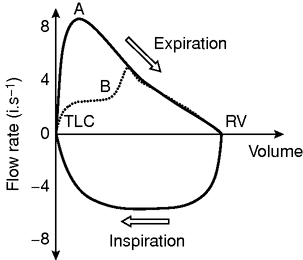
Diagram of normal flow-volume loop.
Factors affecting resistance:
1. Diameter of airways: reduction in diameter increases airway resistance
Bronchoconstriction, e.g. asthma, COPD, stimulation of parasympathetic nervous system
Reduced elastic recoil, e.g. emphysema
Airway inflammation, e.g. asthma, bronchitis
Excess mucus, e.g. cystic fibrosis, bronchitis
Upper airway obstruction, e.g. foreign body, tracheal stenosis
2. Type of gas flow: during laminar flow there is less resistance than during turbulent flow
Low density gases, i.e. helium, are more likely to produce laminar flow
3. Lung volume: at high lung volumes the airways are pulled opened therefore reducing resistance.
4. Anaesthesia: general anaesthesia increases resistance by decreasing FRC.
Flow is plotted against volume to produce a diagrammatic representation of spirometric effort. It is a continuous loop of expiration (starting at total lung capacity, TLC) and inspiration (starting at residual volume, RV). The maximal flow rate during expiration is around 8–10 l/s, and during inspiration is 4–6 l/s.
Obstructive lung disease
Peak expiratory flow rate (PEFR) is reduced. There is a scooped out appearance of the expiratory limb and a reduction in flow rates throughout expiration. RV is increased owing to gas trapping.
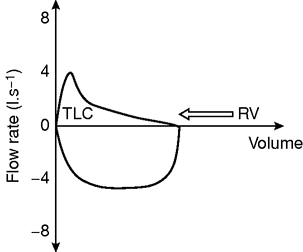
Diagram of flow-volume loop in obstructive lung disease.
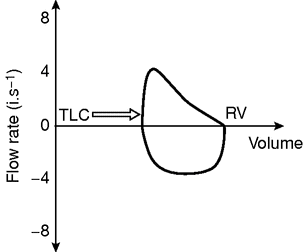
Diagram of flow-volume loop in restrictive lung disease.
The PEFR and total volume expired are reduced. TLC is reduced, shifting the curve to the right.
Further reading
Physiology and biochemistry: control of blood pressure
1. Shock can be classified into four different modes; hypovolaemic, cardiogenic, neurogenic and distributive. With regards to this classification, which of the following statements are correct?
a) Tachypnoea is a relatively early sign in hypovolaemic shock
b) Tachycardia can be the primary pathology
c) Capillary refill time is usually low in all forms of shock
d) Central venous pressure is usually elevated in cardiogenic shock
e) Skin temperature is not normally changed in obstructive shock
2. With respect to the renal and central nervous control of blood pressure and perfusion, which of the following statements are correct?
a) Hypertension typically results in an increased vagal output causing a reduction in systemic vascular resistance
b) The adrenal medulla is innervated by postganglionic sympathetic fibres
c) The resting autonomic tone is inherently sympathetic
d) Renal blood flow is highly variable, and is controlled by the calibre of the afferent and efferent arterioles
e) Increase in sympathetic outflow increases renal blood flow
Answers: 1. TTFTF; 2. TFTFF
The control of blood pressure (BP) and blood flow are two distinctly different phenomena, and are central components to the physiology underpinning good anaesthetic practice. This control is via a complex communication between many organ systems.
Figure 7.4 shows the complex communication between the different receptors and effectors, and their connection with the central nervous system. Tachycardia and tachypnoea are both relatively early signs of hypovolaemic shock. However, in the fit, young patient, hypotension may be a late sign of hypovolaemia. Both bradycardia and tachycardia may be a cause of hypotension. As the heart rate (HR) drops, BP will also be reduced. With respect to tachycardia, an extremely high HR will reduce the cardiac filling time in diastole, and hence impact negatively on stroke volume. In most situations, modest tachycardia is a beneficial compensatory mechanism to maintain perfusion pressure.
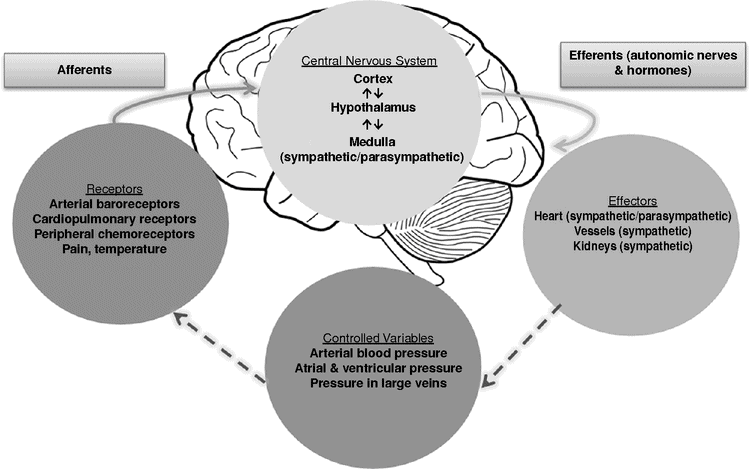
Control of blood pressure and flow.
Table 7.1 shows changes of variables commonly affected by the different types of shock. An understanding of these variables will arm the clinician with the ability to administer the correct treatment in each clinical scenario. The importance of this skill, for example, would be the avoidance of vasopressors in the patient with cardiogenic shock.
Hypovolaemic | Obstructive | Cardiogenic | Distributive | |
---|---|---|---|---|
Blood pressure | ↓ | ↓ | ↓ | ↓ |
Central venous pressure (preload) | ↓ | ↑ | ↑ | ↓ |
Capillary refill time | ↑ | ↑ | ↑ | ↓ |
Skin temperature | ↓ | ↓ | ↓ | ↑ |
Central control of BP is modulated via the autonomic nervous system. The resting tone is inherently sympathetic, and this is evident during a myocardial infarction when all autonomic communication is arrested. In this scenario the HR falls.
The kidney is also involved in maintaining pressure and flow. The afferent and efferent arterioles constrict and dilate in response to changing BP, resulting in a relatively constant renal blood flow. Renal blood flow is reduced by sympathetic nerve activity as part of the arterial baroreceptor response to decreased BP. The macula densa within the kidney is involved in the tubulo-glomerular feedback system. More adenosine is released if the renal perfusion pressure rises. Nitric oxide is a vasodilator and can be produced when the renal perfusion pressure falls.
Therefore, it is important to consider each of the causes of hypotension in turn and systematically determine which is the most likely, thus allowing you to choose the correct treatment.
Further reading
Physiology and biochemistry: factors affecting ICP
Following a fall down some stairs, a 56-year-old man is promptly admitted to ICU with an isolated head injury. He had a Glasgow Coma Score (GCS) of 5 prior to intubation and has evidence of cerebral contusions on his CT brain scan.
Immediate management should include maintenance of the following, with the exception of which ONE?
a) Normocapnia
b) Normoglycaemia
c) Normotension
d) Hypothermia
e) Normoxia
Answer: d)
Head injury is a common presentation to emergency departments, with a significant mortality and morbidity. Eighty-five per cent of patients suffering a severe head injury will be either dead or disabled at 1 year post-injury. It can be classified in a number of ways depending on the mechanism and type of injury, or based on the patient’s GCS. The GCS is useful in helping to predict outcome following a significant head injury, with the motor component being particularly useful. Simply speaking, traumatic brain injury (TBI) is graded as mild (GCS 14–15), moderate (GCS 9–13) and severe (GCS ≤ 8).
Intracranial pressure (ICP) depends on the volume of the intracranial contents, which normally comprises 1.4 kg brain, 50–70 ml blood and 50–120 ml cerebrospinal fluid. A normal ICP is 7–17 mmHg (1–2 kPa). An increase in volume of any one of the intracranial components will be counterbalanced by a decrease in the others to a point, after which ICP will exponentially increase, reducing cerebral perfusion and therefore oxygenation (cerebral perfusion pressure (CPP) = mean arterial pressure (MAP) – ICP). Under normal conditions autoregulation maintains a constant blood flow between a MAP 50 mmHg and 150 mmHg. However, in traumatized or ischaemic brains, cerebral blood flow (CBF) may become blood pressure dependent.
Hypercapnia causes vasodilatation, increasing CBF and therefore increasing the intracranial contents and ICP. Hypocapnia (pCO2 < 3.3 kPa) will not cause any further reduction in CBF and offers no advantage. In fact it is detrimental, as it shifts the oxygen dissociation curve to the left, impairing cerebral oxygen delivery. Arterial pO2 has a minimal effect until it falls below 6.7 kPa at which point CBF increases significantly.
This patient has sustained a severe TBI and is at risk of cerebral oedema. He should ideally be managed in a specialist neurosurgical unit with ICP monitoring. He should be discussed with a neurosurgeon, and hopefully arrangement for transfer can be made. While this is happening, it is important to target the prevention of secondary brain injury by addressing the key principles of ongoing management of the head-injured patient on the ICU and maintaining normotension, normoxia, normocapnia, normothermia and normoglycaemia.
There is no evidence that induced hypothermia is beneficial in the immediate management of head injury, but it may be necessary as part of the management of persistently raised ICP.
Please refer to the management algorithm for patients with severe traumatic brain injury in Chapter 31.
Further reading
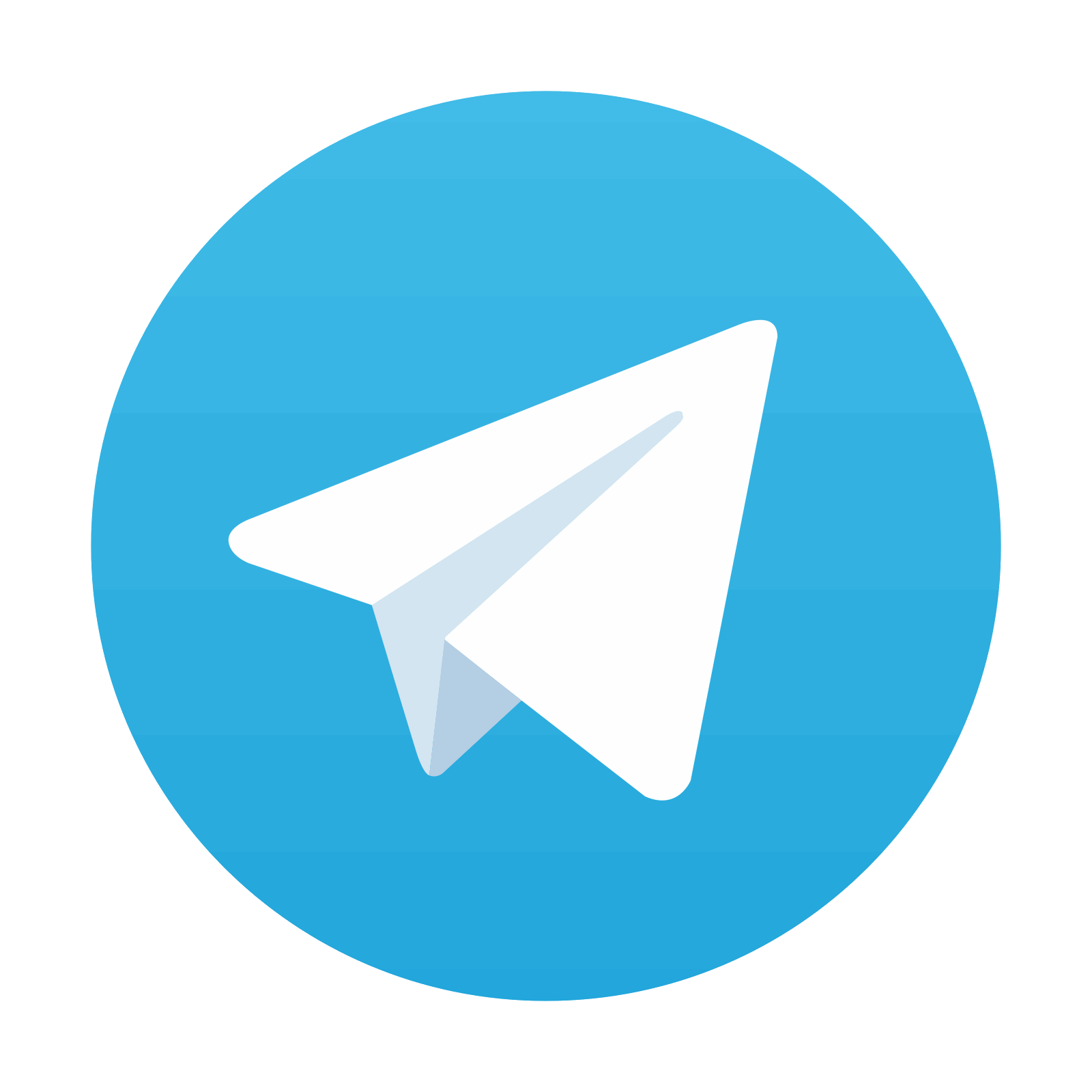
Stay updated, free articles. Join our Telegram channel

Full access? Get Clinical Tree
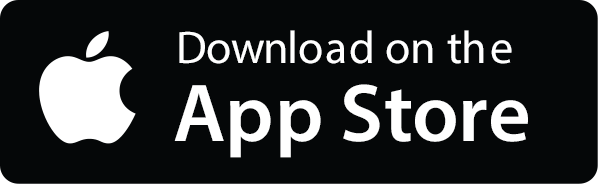
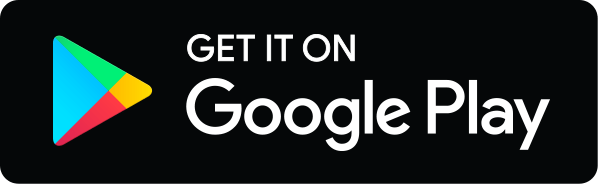