Trauma resuscitation is a complex and dynamic process that requires a high-performing team to optimize patient outcomes. More than 30 years ago, Advanced Trauma Life Support was developed to formalize and standardize trauma care; however, the sequential nature of the algorithm that is used can lead to ineffective prioritization. An improved understanding of shock mandates an updated approach to trauma resuscitation. This article proposes a resequenced approach that (1) addresses immediate threats to life and (2) targets strategies for the diagnosis and management of shock causes. This updated approach emphasizes evidence-based resuscitation principles that align with physiologic priorities.
Key points
- •
Apply evidence-based clinical measures to identify shocked patients with trauma, such as Shock Index (SI) greater than or equal to 1.0, prehospital systolic blood pressure (sBP) less than 90 mm Hg, or sustained sBP less than 110 mm Hg.
- •
Critical hypoxemia or dynamic airway in patients with trauma represents the rare circumstance for immediate definitive airway management on emergency department arrival.
- •
Among most patients with trauma in shock, a resequenced approach to trauma care is preferable, emphasizing physiologic optimization before intubation.
- •
A reduction of 25% to 50% of the usual rapid sequence intubation induction agent dose is recommended in patients with SI greater than or equal to 1.0 or other evidence of shock.
Case 1.1: advanced Trauma Life Support approach
A 63-year-old female pedestrian is struck by a car. Her Glasgow Coma Scale (GCS) score in the field is 13. In the emergency department (ED), evaluation proceeds according to the Advanced Trauma Life Support (ATLS) protocol. Her vitals are as follows: respiratory rate (RR) 26 breaths/min, O 2 saturation 90% on 100% oxygen, heart rate (HR) 105 beats/min (bpm), blood pressure (BP) 103/80 mm Hg, and temperature 36.0°C. Her airway is assessed to be patent, and air entry is decreased in the right chest. A focused assessment with sonography in trauma (FAST) examination is positive for free fluid in the right upper quadrant. Her pelvis is mechanically stable. Her peripheral neurologic examination is normal. She has no past medical history, takes no medications, and has no drug allergies.
Following the A-B-C-D-E (airway-breathing-circulation-disability-exposure) heuristic, the team identifies her management priorities as (1) need for intubation based on projected clinical course, (2) chest tube placement, (3) blood product administration, (4) axial imaging (panscan). According to the ATLS shock classification, the patient is in class II shock (estimated blood loss, 750–1500 mL). Two units of uncrossmatched packed red blood cells (PRBCs) are requested and 1 L of crystalloid is given as an initial bolus.
A rapid sequence intubation (RSI) with 120 mg (2 mg/kg) of ketamine and 120 mg (2 mg/kg) succinylcholine is performed without difficulty. Five minutes after intubation, the patient becomes profoundly hypotensive, with a BP of 53/30 mm Hg. Suspecting a tension pneumothorax, the team quickly decompresses the right chest with a needle thoracostomy in the second intercostal space, midclavicular line, and an additional 1 L of crystalloid is given before blood products. A massive transfusion protocol (MTP) is activated, and tranexamic acid (TXA) is administered. Following chest tube placement, the patient’s BP improves to 75/40 mm Hg; however, she remains hypotensive for an additional 30 minutes until 3 units of PRBCs are administered. Her hemodynamics stabilize, and she is transported to the computed tomography (CT) suite, where her systolic BP once again decreases to less than 70 mm Hg, requiring further blood products. She is found to have a small subdural hematoma, a right-sided pneumothorax, and a grade 3 liver laceration.
Case 1.1: advanced Trauma Life Support approach
A 63-year-old female pedestrian is struck by a car. Her Glasgow Coma Scale (GCS) score in the field is 13. In the emergency department (ED), evaluation proceeds according to the Advanced Trauma Life Support (ATLS) protocol. Her vitals are as follows: respiratory rate (RR) 26 breaths/min, O 2 saturation 90% on 100% oxygen, heart rate (HR) 105 beats/min (bpm), blood pressure (BP) 103/80 mm Hg, and temperature 36.0°C. Her airway is assessed to be patent, and air entry is decreased in the right chest. A focused assessment with sonography in trauma (FAST) examination is positive for free fluid in the right upper quadrant. Her pelvis is mechanically stable. Her peripheral neurologic examination is normal. She has no past medical history, takes no medications, and has no drug allergies.
Following the A-B-C-D-E (airway-breathing-circulation-disability-exposure) heuristic, the team identifies her management priorities as (1) need for intubation based on projected clinical course, (2) chest tube placement, (3) blood product administration, (4) axial imaging (panscan). According to the ATLS shock classification, the patient is in class II shock (estimated blood loss, 750–1500 mL). Two units of uncrossmatched packed red blood cells (PRBCs) are requested and 1 L of crystalloid is given as an initial bolus.
A rapid sequence intubation (RSI) with 120 mg (2 mg/kg) of ketamine and 120 mg (2 mg/kg) succinylcholine is performed without difficulty. Five minutes after intubation, the patient becomes profoundly hypotensive, with a BP of 53/30 mm Hg. Suspecting a tension pneumothorax, the team quickly decompresses the right chest with a needle thoracostomy in the second intercostal space, midclavicular line, and an additional 1 L of crystalloid is given before blood products. A massive transfusion protocol (MTP) is activated, and tranexamic acid (TXA) is administered. Following chest tube placement, the patient’s BP improves to 75/40 mm Hg; however, she remains hypotensive for an additional 30 minutes until 3 units of PRBCs are administered. Her hemodynamics stabilize, and she is transported to the computed tomography (CT) suite, where her systolic BP once again decreases to less than 70 mm Hg, requiring further blood products. She is found to have a small subdural hematoma, a right-sided pneumothorax, and a grade 3 liver laceration.
Introduction
Trauma resuscitation is a complex and dynamic process that is best managed by experienced, highly trained teams. Until the development of ATLS, patients received heterogeneous care by physicians lacking formal trauma resuscitation training. The development of ATLS brought a much needed standardized approach to the care of critically injured patients. It facilitated a common language among care providers, highlighting the importance of a team approach and introduced the A-B-C-D-E sequence to trauma care.
Using this simplified algorithm, teams are directed to assess injuries during a primary trauma survey in a predictable and sequential manner (ie, address A before moving on to B, and so on). The premise is to move in a stepwise and ordered fashion, particularly when multiple injuries may exist, in order to, for example, not miss the subtle tension pneumothorax by fixating on the obvious limb amputation. This paradigm is based primarily on experience rather than carefully conducted trials.
The sequence of priorities in ATLS until recently aligned similarly with the Advanced Cardiac Life Support (ACLS) approach of airway-breathing-circulation (A-B-C). Updated guidelines, based on new evidence, introduced a radical reordering of ACLS priorities to C-A-B in an effort to emphasize chest compressions. High-quality chest compressions are linked to improved survival in medical cardiac arrest, and interruptions to cardiopulmonary resuscitation for any reason is associated with increased mortality.
In major trauma, the value of strict and rigid adherence to the A-B-C-D-E sequence is also questionable. Specifically, the ATLS approach overemphasizes the need to immediately secure a definitive airway and undervalues the importance of shock identification and preintubation resuscitation. The primacy of the airway often comes at the expense of more critical interventions related to supporting circulation and preventing hemodynamic collapse.
This article outlines an evidence-based update for the initial resuscitation of critically injured patients on arrival in the ED. It presents an algorithm ( Fig. 1 ) that focuses first on immediate threats to life followed by the identification and targeted management of shock and occult shock causes. In this resequenced approach, intubation should occur after the resuscitation has been initiated, unless critical hypoxia or a dynamic airway injury is present. Where evidence is lacking, a preferred approach is explicitly provided, based on our experience.
Case 1.2: resequenced approach
The case presented at the beginning of this article highlights the potential issues that can arise by strictly adhering to the A-B-C-D-E sequence outlined by ATLS. Specifically, the patient is intubated before the management of both obstructive and hemorrhagic shock, prompting a dangerous but predictable episode of postintubation hypotension. Using a resequenced approach, this article presents a management plan to mitigate these risks by way of targeted interventions to manage a shock state before RSI.
In trauma resuscitation resequenced, the team addresses key management priorities, as outlined in Fig. 1 . The patient requires intubation urgently but not immediately, given she is self-ventilating with an oxygen saturation of 90% and is protecting her airway. With a positive FAST and Shock Index (SI) greater than 1.0, the team leader chooses to focus on hemodynamic optimization before RSI, specifically a presumed right-sided tension pneumothorax and intra-abdominal injury. The SI, defined as the HR divided by systolic BP, is an important clue to the presence of shock and occult shock, and is sensitive for predicting critical bleeding, the need for blood products, and incidence of postintubation hypotension. The team leader fosters a shared mental model (SMM) with the team members by verbally outlining management priorities in the following sequence: (1) blood product administration and MTP activation, (2) right chest decompression with finger thoracostomy, and (3) RSI using a reduced dose of ketamine. The team performs regular airway and neurologic evaluations to monitor for clinical deterioration that may warrant more rapid airway intervention. Following finger thoracostomy, the patient’s oxygen saturation improves to 95%. Two units of PRBCs are administered, HR decreases to 85 bpm, and the BP remains 105/50 mm Hg. An uncomplicated RSI is performed using a reduced dose of ketamine (0.5 mg/kg). There is no postintubation hypotension. The patient is given postintubation analgesia and sedation, and remains hemodynamically appropriate during transport to, from, and during CT imaging. In case 1.1, the clinicians doggedly followed the ATLS algorithm. As a result, the patient experienced prolonged hypotension with a risk of postintubation cardiac arrest, which increased her risk for a poor neurologic outcome related to prolonged hypoperfusion in the setting of a significant head injury. In contrast, the team in case 1.2 optimized the patient’s hemodynamic status before intubation, resulting in more consistent hemodynamics following intubation.
The resequenced approach to trauma resuscitation: a shared mental model
According to SMM theory, team performance is optimized when team members have a common understanding of the team and task requirements without the need for explicit discussion. The algorithmic features of ATLS may help to foster an SMM among team members. A resequenced plan for trauma resuscitation requires that all team members understand how and when priorities should deviate from the A-B-C-D-E approach. Preferably, regular team training exercises and/or prebriefings before patient arrival should be used to establish a workable SMM of team-based and task-based processes, particularly when it differs from the standard ATLS approach. The importance of resequencing SMMs cannot be overstated, because the resequenced approach to trauma resuscitation needs to be operationalized with careful consideration to each team member’s understanding of process and order of care.
Where does airway fit within trauma resuscitation?
As outlined in Fig. 1 , airway evaluation is necessary immediately on patient arrival as part of the primary survey. Whether definitive airway management must always occur before interventions related to breathing or circulation is questionable, particularly when physiologic considerations instead support the prioritization of obstructive or hemorrhagic shock causes. In our experience, most patients with trauma do not immediately require a definitive airway (eg, within the initial 10 minutes of arrival). Instead, the airway can be managed temporarily with supportive maneuvers while an airway plan is devised and physiologic risks for postintubation hypotension are addressed.
Intubation causes an increase in intrathoracic pressure, resulting in a decrease in right atrial pressure. The reversal in intrathoracic physiology following intubation (compared with spontaneous respirations) has a significant impact on the shock states that are common among patients with trauma. In hemorrhagic shock, an already low venous return can decrease further with positive pressure ventilation. Although not studied exclusively in trauma, preintubation hypotension is a significant risk factor for postintubation cardiac arrest, highlighting the importance of adequate volume resuscitation before intubation. In obstructive shock states (eg, tension pneumothorax, cardiac tamponade), decreased venous return and may also be worsened by positive pressure ventilation. Focused interventions to relieve obstructive causes should be considered before intubation in order to optimize hemodynamics and establish safer peri-intubation and postintubation conditions.
There are several situations in which definitive airway management must be the immediate priority of the trauma team:
- 1.
Critical hypoxemia, despite high-flow oxygen and supportive airway maneuvers
- 2.
Dynamic airway: blunt, penetrating, or burn injuries to the head, neck, or oropharynx that may compromise oxygenation, ventilation, or airway management, and that are suspected to worsen on a very short time course
Even when airway is the immediate priority, concurrent management of shock states can occur to a greater or lesser extent, depending on team size and structure. A single physician working with a respiratory therapist and nurse may elect to perform a needle decompression and request blood products in line with intubation efforts, whereas a larger team may be able to address multiple priorities concurrent with airway management.
In summary, a small minority of patients with trauma (those with critical hypoxia or dynamic airway injuries) require definitive airway management immediately on arrival in the ED. Instead, most patients benefit from aggressive resuscitation before intubation. Interventions include those that stay hemorrhage, optimize perfusion, and relieve obstructive shock causes. Once these interventions are initiated or completed, then definitive airway management can proceed.
Identification of shock and occult shock in patients with trauma
Shock is a clinical state defined by circulatory failure resulting in insufficient cellular oxygen use, and manifests with clinical and hemodynamic derangements. Occult shock can exist if these obvious clinical disturbances are subtle or absent despite underlying hypoperfusion. In an effort to identify occult shock, several strategies have been proposed using biomarkers (lactate, base deficit [BD]), vital signs (BP, HR), or SI, each of which have specific benefits and limitations.
Early shock identification is critical in order to prevent clinical deterioration and refractory shock states. Furthermore, airway management that precedes resuscitation can worsen hemodynamics. Several studies show that an SI greater than or equal to 0.9 and preintubation systolic BP (sBP) less than 90 mm Hg are independent predictors for postintubation cardiac arrest. Hypotension, hypoxemia, and pH/acidosis (the HOP killers) are physiologic disturbances that can result in poor outcomes among critically ill ED patients undergoing emergency airway management. Although not unique to patients with trauma, they should be considered and corrected in line with, and ideally before, active airway management for patients in whom shock or occult shock is suspected. Delays in the identification and management of shock and occult shock among patients with trauma is associated with poor outcomes, such as multiorgan failure, increased intensive care unit (ICU) length of stay, and ultimately death. The imperative to identify patients with shock is clear, particularly because early damage control surgery results in substantial benefits. The early identification of occult shock is equally important because under-recognition is associated with worse patient outcomes.
Challenges exist in the early identification of shock in patients with trauma. Variable pathophysiologic and compensatory mechanisms produce a range of hemodynamic presentations that complicate assessment. Traditionally, ED physicians have applied the ATLS shock classification to predict the amount of blood loss, a system comprising 4 shock classes using vital signs and mental status. The widespread application of this system occurred without formal study or validation and recently the accuracy of these classifications has been called into question. ATLS underestimates the prevalence of bradycardia in hemorrhagic shock and fails to consider the complex pathophysiology related to blood loss and tissue injury. A study of a German trauma registry showed that only 9.3% of patients with trauma can be properly categorized according to ATLS shock classification. In an audit of the United Kingdom Trauma Registry from 1989 to 2007, patients progressing to stage 4 shock (>2 L of blood loss) increased HR from 82 to 95 bpm, while showing no significant changes in GCS, respiratory rate, or sBP. The ATLS shock categories are inaccurate in geriatric patients with trauma, because of decreased physiologic reserve, comorbid disease, restricted vascular elasticity, and concurrent use of cardiovascular medications. The traditional shock categories also do not account for other causes of shock (obstructive, neurogenic) or mixed shock causes.
Over the past decade, clinicians and researchers have sought simple and more accurate methods for identifying and quantifying shock in patients with trauma. In general, these techniques are best studied in hemorrhagic shock, with the exception of bedside ultrasonography, which can be used to identify hemorrhagic and some forms of obstructive shock. During the initial resuscitation period, indices for shock identification can be organized into 2 broad categories:
- 1.
Clinical indicators: obtained exclusively at the bedside
- 2.
Laboratory indicators: additional laboratory testing required
The role of each indicator as a potential alternative to the traditional ATLS approach is discussed here, highlighting their utility during the prehospital phase and initial resuscitation period (time spent in the ED before transport to imaging or definitive care). There is no perfect indicator to reliably and accurately identify shock in all cases; each has benefits and pitfalls, with applicability related to local resources and clinician preference. Our approach to using each indicator is summarized in Table 1 .
Indicator | How It Is Applied to Practice |
---|---|
Clinical Indicators | |
Vital signs |
|
SI |
|
Ultrasonography | eFAST examination for all patients with trauma Pericardial fluid on eFAST should prompt cautious approach to intubation, preferably in operating room with surgeon prepped for intervention eFAST before intubation to rule out clinically significant pneumothorax Serial eFAST examinations if persistent hemodynamic compromise without initial signs of hemorrhage Pneumothorax visualized on ultrasonography and hemodynamic instability warrants immediate chest decompression Rare application of IVC examination |
Laboratory Indicators | |
BD | Patients with a BD >6 require aggressive resuscitation and risk hypotension during/after intubation BD >6 requires significant diagnostic effort to identify source of hemorrhage and/or shock cause Integrate in decision making for those at risk of crumping after departure from trauma bay Preferential reliance in patients with suspected shock and concomitant alcohol intoxication |
Lactate | Complements BD and often used interchangeably Levels >4.0 warrant increased suspicion for occult shock, in combination with other indicators |
Viscoelastic assays (ROTEM or TEG) | Acknowledge limited evidence for use Reasonable predictor of MTP Integrate abnormal findings into decision for targeted blood product administration (eg, evidence of hyperfibrinolysis, consider second dose of TXA) |
Clinical indicators
Vital Signs
Despite their limitations, vital signs are still a central element of the trauma primary survey. Vital signs are obtained immediately on patient arrival and repeated frequently; trends in vital signs can often be more informative than a single, static measurement.
Hypotension is defined by ATLS as sBP less than or equal to 90 mm Hg, but this cutoff is debatable. A sustained sBP less than or equal to 90 mm Hg is associated with a 60% mortality and BD values of greater than 20, suggesting that this cutoff may capture only the sickest of patients. Several studies have proposed that an sBP of less than 105 or less than 110 mm Hg may be more appropriate estimates of hypoperfusion. In one study, patients with trauma with an sBP of 90 to 109 mm Hg had a significantly increased risk of death (5% vs 1%) compared with those with an sBP greater than 109 mm Hg. The investigators concluded that an sBP of 90 to 109 mm Hg warrants aggressive resuscitation and surgical evaluation. Even a single, isolated decrease in sBP to less than 105 mm Hg is associated with a 12-fold increase in the need for immediate therapeutic intervention.
The importance of prehospital hypotension that normalizes without intervention should not be underestimated. Multiple studies have shown an association between isolated prehospital hypotension and an increased risk for serious injury, surgical intervention, and mortality. Repeat episodes of hypotension in the ED confer an even greater risk of serious morbidity and death.
The predictive value of vital signs among geriatric patients remains uncertain. In one study, 20% of patients more than 65 years old had normal vital signs (defined as sBP >90 mm Hg and HR <120 bpm) despite laboratory measures suggesting hypoperfusion. These data align with other studies to support the need to redefine normal in the geriatric population. In a separate study, HR did not predict massive transfusion among geriatric patients. In contrast, sBP, pulse pressure, and diastolic BP all proved to be strongly predictive of more than 5 units of PRBCs within 24 hours of arrival to hospital.
In addition, physical examination findings can provide additional evidence of shock. Poorly perfused extremities or loss of central pulses are important findings in profound shock; however, their reliability and accuracy have not been well studied. BP as assessed by the presence or absence of peripheral or central pulses tends to underestimate the degree of hypotension. Used together, our experience is that combining an assessment of trends in vital signs, adequacy of peripheral perfusion, and physical examination findings can yield a more accurate estimate of the presence and degree of shock during the primary survey, compared with BP or HR alone.
Shock Index
The SI, defined as HR/sBP, is a simple and reliable method for estimating degree of shock and predicting clinical outcomes in a variety of settings, including trauma resuscitation. The SI is useful in trauma because of its simplicity, strong inter-rater reliability, and accuracy as a predictor of blood loss. Although there is no precise cutoff for an abnormal SI, a value of greater than or equal to 1.0 is associated with significant increases in transfusion requirements, injury severity, and mortality. Estimating an SI of greater than or equal to 1.0 is also straightforward and requires no formal calculation (HR > sBP), which is important during periods of high cognitive and task load.
Notably, the SI is predictably unreliable in the following circumstances :
- 1.
Altered physiologic compensation (eg, geriatrics)
- 2.
Presence of underlying medical conditions (eg, undertreated hypertension)
- 3.
Medication-related disturbances (eg, β-blockers)
Importantly, geriatric patients with trauma often have 1 or more of these circumstances. Although the best available evidence strongly supports the utility of SI in trauma as a predictor of clinical outcomes, the results are less definitive among geriatric patients. In one study of more than 200,000 patients with trauma, SI was the strongest predictor of mortality among patients 65 years of age and older. In contrast, a smaller study of 1987 patients did not find that SI was an independent predictor of mortality. This difference highlights the complexity of geriatric physiology following critical injuries and the challenges that face clinicians in detecting shock among elderly patients.
The delta SI (d-SI) has been proposed as a way to overcome the underlying hypertension and impaired tachycardic responses that are prevalent among geriatric patients with trauma. The d-SI is defined as the change in SI from the prehospital setting to arrival in the ED. A difference of greater than 0.1 is considered significant, and suggests the presence of shock or occult shock. A recent retrospective study found that mortalities were significantly higher among those with d-SI exceeded 0.1 (16.6% vs 9.5%). Although these data are preliminary, they may prove useful once confirmed in prospective studies.
In our practice, the authors use an SI of greater than 1 or d-SI of greater than 0.1 in conjunction with the presence of isolated hypotensive episodes to identify patients at risk for worse outcome.
Ultrasonography
The FAST is a standard part of the evaluation of patients with trauma. The presence of abdominal free fluid directs clinicians to consider intra-abdominal organ injury as a source of hemorrhage, and in the presence of hypotension supports emergent operative intervention. In contrast, a negative FAST study despite hypotension should generate a search for extra-abdominal causes of hemorrhage and other shock causes. Pericardial fluid is also rapidly identified during the FAST examination, and, when present, prompts consideration for tamponade physiology as a cause for shock. Notably, the evidence is limited for improved patient outcomes with the integration of a FAST examination. Several investigators have questioned the role of the FAST examination in hemodynamically stable patients. Despite these limitations, given the minimal impact on team efficiency and acquisition of potentially useful data, the authors follow the published guidelines applying a FAST examination for patients with all blunt mechanisms and most patients with penetrating mechanisms of trauma.
The extended FAST (eFAST) includes an evaluation of thoracic structures for pneumothorax and hemothorax. There is growing evidence that eFAST is a superior diagnostic modality to supine chest radiograph (CXR) for detecting pneumothorax. Compared with supine CXR, emergency physician (EP)–performed lung ultrasonography shows superior sensitivity (86%–98% vs 28%–75%) and equal specificity (98%–100% vs 100%). Whether this translates to improved patient-oriented outcomes remains to be seen. These studies support our current diagnostic approach in the trauma bay with eFAST integration during the primary survey, followed by CT when stability permits.
The utility and accuracy of bedside ultrasonography to assess the inferior vena cava (IVC) has not been systematically studied. In critical care, the utility may only exist at extremes of measurements, but this remains a matter of much debate. In our experience, when ultrasonography shows findings suggestive of shock, assessing the IVC to estimate size, collapse, and respiratory variation rarely adds to our clinical impression.
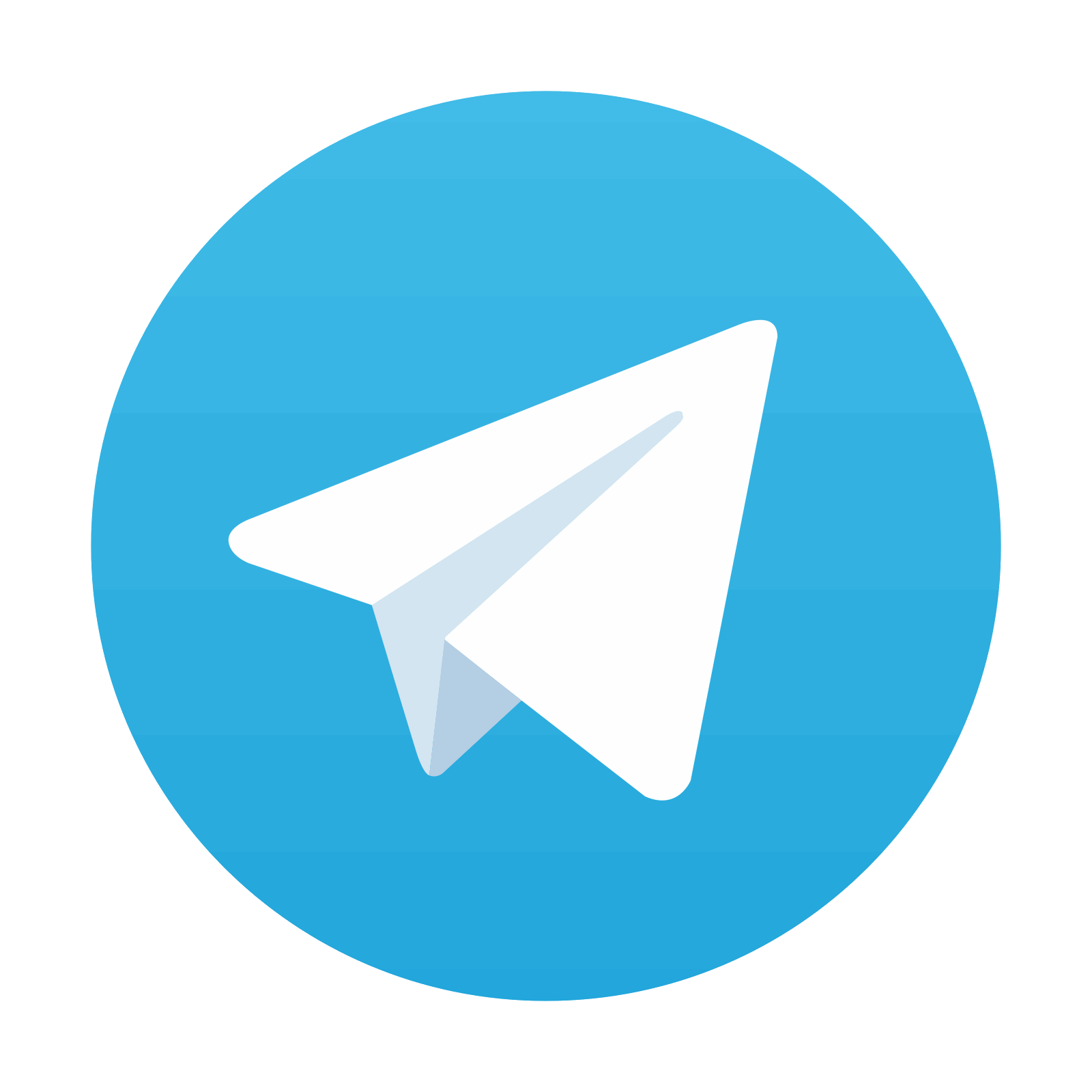
Stay updated, free articles. Join our Telegram channel

Full access? Get Clinical Tree
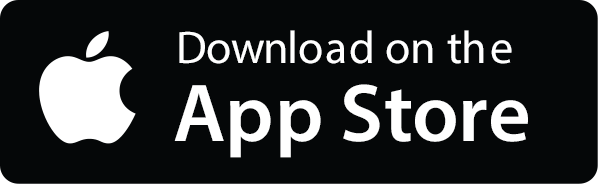
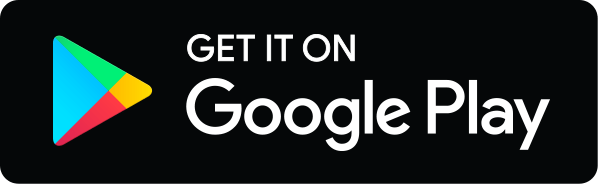