Respiratory, Renal, and Cardiovascular Postoperative Complications
Alessia Pedoto
David Amar
Key Points
1. Respiratory complications occur in 12% to 26% patients and account for the majority of morbidity and mortality following thoracic surgery. Postoperative predicted DLCO (ppDLCO) may be used to identify high-risk patients preoperatively. Prolonged air leak is the most common respiratory complication following thoracic surgery.
2. Renal complications following thoracic procedures are uncommon and usually occur in the setting of sepsis. Use of NSAIDs, dehydration, and preexisting renal disease are all predisposing factors. Pharmacologic therapy is generally not effective, and prevention continues to be the desired strategy.
3. Supraventricular arrhythmias are common after lung resection and are typically transient, but increase morbidity and hospital length of stay. Diltiazem appears to be effective in preventing postoperative atrial fibrillation.
Clinical Vignette
A 72-year-old man with long-standing smoking history underwent a thoracotomy and right pneumonectomy for nonsmall cell lung cancer and was admitted to the surgical ICU postoperatively. He has diet controlled diabetes mellitus type II and hypertension. Preoperative medications include lovastatin and lisinopril.
Pneumonectomy is one of the surgical curative options for nonsmall cell lung cancer (NSCLC).1,2 It is usually considered for extensive tumors or for tumors located in specific anatomic areas, often as part of a multimodal approach combined with perioperative chemo3 and radiation treatment.4
Despite strict selection criteria, improved surgical and anesthetic techniques, and enhanced postoperative care, patients like the one described in the clinical vignette still suffer significant postoperative complications following lung resection surgery. There is considerable variability in the reported mortality rates after pneumonectomy (5%-6%) which depend on the case volume of the hospital, the age of the patient, the side of surgery4–7 and the use of induction chemotherapy.3 Old age, poor nutritional status, current smoking, and coronary artery disease8 are all well-known risks factors associated with an increased morbidity and mortality after lung resection. Respiratory complications are especially prevalent and a major contributor to morbidity in this patient population, who often exhibits preexisting pulmonary disease. The presence of COPD may increase the risk of developing bronchopleural fistulas and acute respiratory failure.8 Additionally, predicted postoperative DLCO (ppDLCO) is the strongest predictor of increased operative mortality and respiratory morbidity, independently from the presence of COPD9,10 (see Chapter 9). Unfortunately not all centers perform routine DLCO measurements, more so in the presence of normal spirometric measurements.
As a result of all the above, great effort should be tailored in preventing postoperative complications, since they are associated with an increase in ICU admission rates, hospital length of stay and mortality rates.8 This chapter focuses on important respiratory, renal, and cardiovascular complications following thoracic surgery.
POD1: The patient’s (from Clinical Vignette) oxygenation worsened and he was placed on 100% nonrebreather mask. His condition continued to deteriorate and was reintubated on the morning of POD2. His CXR showed diffuse pulmonary infiltrates on the left lung and normal postoperative changes in the right hemithorax.
RESPIRATORY COMPLICATIONS
Respiratory complications are common after pneumonectomy (especially right sided), and several risk factors have been suggested. They account for the majority of morbidity and mortality, despite an overall improvement in surgical and postoperative strategies.
Table 23-1 summarizes early complications after pneumonectomy, while Table 23-2 summarizes the common radiographic findings in these patients. Early respiratory complications are discussed here.
Table 23–1. Incidence of Common Early Complications after Pneumonectomy1,3,5,6,8,69,76
Table 23–2. Radiographic Characteristics of Respiratory Complications112
POSTPNEUMONECTOMY PULMONARY EDEMA
Postpneumonectomy pulmonary edema (PPPE) is characterized by a sudden onset of noncardiogenic pulmonary edema, which is fatal in more than 60% patients if not recognized. It occurs in 2% to 4% pneumonectomy cases, especially right-sided ones. The etiology is unknown and its clinical features are undistinguishable from the adult respiratory distress syndrome (ARDS).11 A high index of suspicion is necessary for the diagnosis and cardiac failure, pneumonia, sepsis as well as bronchopleural fistula all need to be excluded. Pulmonary infiltrates on chest x-ray and respiratory distress on clinical exam are common diagnostic features.11,12
The onset of PPPE is usually within the first two postoperative days and can present with oliguria in patients with normal preoperative renal function.11 Hypovolemia is usually present due to fluid restriction and possible sequestration of water into a “leaky” lung. Oliguria and pulmonary infiltrates on chest x-ray preceded the onset of dyspnea by an average of 23 hours in a small study.13 Other signs included tachycardia, lung rales, and hypoxemia, and sometimes the presence of subcutaneous emphysema on the operative side.12 Fever (>38°C) and leukocytosis can also be present. Hypoxemia is usually unresponsive to oxygen therapy or diuretics (including “renal dose” dopamine), and often requires endotracheal intubation within the first three days after surgery.13 The treatment is largely supportive and includes diuretics, oxygen, mechanical ventilation, and fluid restriction.
The pathophysiology of PPPE is unclear, with several proposed mechanisms. Volume overload remains a very controversial explanation. Some of the landmark literature originated from animal studies, with variable results on the development of edema. Not all the fluid overloaded animals developed PPPE, and the timing between the clinical manifestation and the diagnosis made at the autopsy remains unclear.12 In a series of 5 case reports,13 PPPE developed in patients who were both eu- and hypovolemic, suggesting more complicated mechanisms.
An increase in the alveolo-capillary membrane permeability has been demonstrated by Waller et al14 who measured the amount of radioisotopic tracer accumulation in lung tissue in patients who underwent pneumonectomy. The changes occurred within 5 hours of surgery. Proposed etiologic factors were neutrophil activation and an increase in pulmonary vascular resistance.14
Impaired lymphatic drainage has been suggested as another potential factor contributing to PPPE. Using a dog model, Little et al15 showed that disruption of the lymphatic drainage was associated with PPPE. However, several other animal and human studies have failed to demonstrate a link between the two. Furthermore, the lung has a significant lymphatic drainage reserve, and further compensation can occur via pleural drainage.11 It is therefore unlikely that lymphatic drainage impairment plays a major role in the development of PPPE.
One-lung ventilation (OLV) with high tidal volumes and hyperoxia has been linked to the damage of the alveolo-capillary membrane. Animal studies have shown that the production of reactive oxygen species associated with high peak airway pressure may cause injury of the alveolo-capillary membrane with an increase in pro-inflammatory cytokines, which may contribute to PPPE.11 In humans, the use of large tidal volume (VT) ventilation with increased peak inspiratory pressure (PIP >40 cm H2O) can be associated with an increase in pulmonary vascular resistance and subsequent pulmonary hypertension.11,16 An important lesson is learned form the ARDS data network on lung injury and high tidal volume.17 A protective lung strategy includes tidal volumes of 3 to 4 cc/kg with OLV, peak inspiratory pressure less than 35 cm H2O and plateau pressures less than 25 cm H2O, with the potential need to accept permissive hypercapnia.
The pneumonectomy empty space can lead to mediastinal shift and hyperinflation of the remaining lung with consequent severe pulmonary hypertension, leading to respiratory distress and death.11 Stabilization of the mediastinum in the midline using intermittent clamping of a water sealed chest tube or use of a balanced drainage system helps prevent this complication12 (see Chapter 22).
A certain degree of right ventricular overload occurs after pneumonectomy, even in the absence of preoperative cardiac disease.11 Patients who develop acute lung injury postoperatively exhibit an increase in mean pulmonary arterial pressures and right ventricular dilatation, and this is usually associated with a poor prognosis.18 Although right ventricular dysfunction is unlikely to represent the main etiologic factor, it may contribute unfavorably to the course of PPPE.
Treatment for PPPE is mainly supportive, consisting of mechanical ventilation, fluid restriction and the use of diuretics. Steroid administration intraoperatively in the attempt to prevent PPPE remains controversial, with the majority of the trials done in the ICU setting in patients with ARDS. Cerfolio et al19 designed a safety study on the role of methylprednisolone administered prior to clamping the pulmonary artery in patients undergoing pneumonectomy, and looked at the rate of complications. The 37 subjects who were treated with corticosteroids had a reduced incidence of PPPE/ARDS when compared to historical controls. However, the study was small, not randomized, and the definition of PPPE was very broad, with no mention of the degree of hypoxia and the amount of infiltrates on chest radiography. There was also no follow up on the potential long-term effects of steroids, making potential clinical recommendations difficult.
Given the grave prognosis and the lack of targeted interventions, it is imperative that the thoracic anesthesiologist be familiar with this complication and that he or she make every effort to minimize its occurrence. Suggested perioperative strategies include minimization of intraoperative volume replacement, the use vasopressors to treat intraoperative hypotension (as opposed to fluid boluses), the use of protective lung ventilation strategies, the administration of supplemental oxygen therapy in the postoperative period to reduce pulmonary vascular resistance, and the use of balanced drainage systems after pneumonectomy.
PNEUMONIA
Postoperative pneumonia still represents a major cause of morbidity and mortality after lung resection. The incidence is variable (2%-40%), depending on the population studied, the extent of surgery, and the type and timing of perioperative antibiotic prophylaxis.20 Clinical diagnosis may be difficult, since hypoxia, fever, or an abnormal chest x-ray may be common findings in the postoperative period. Hypoventilation due to pain, as well as the inability to cough and clear secretions, is commonly associated with atelectasis and eventually postoperative pneumonia. Several independent risk factors have been proposed, such as COPD, FEV1 <70%, age >75, induction chemotherapy, type of surgical resection (lobectomy and bilobectomy versus pneumonectomy), intraoperative bronchial colonization and male gender.20,21 High tidal volumes and increased fluid administration are also contributing factors for pneumonia, as well as other postoperative respiratory complications such as ventilator induced lung injury and pulmonary embolism.22 Postoperative pneumonia is associated with higher rates of re-intubation and noninvasive ventilation modalities, prolonged length of stay in the hospital and in the ICU and overall higher mortality rates (19%). It commonly occurs during the first postoperative week, with a peak on postoperative day four.20,23 Lung resection is usually defined as a “clean–contaminated” procedure, due to the opening of the trachea and bronchi and migration of tracheobronchial contaminants, especially with bacterial strands that are resistant to common antibiotic prophylaxis.20 Several studies20,23 suggest that the most common causative micro-organisms are Haemophilus influenzae (41.7%), Streptococcus pneumoniae (25%), Enterobacteriaceae (8.7%), and Pseudomonas (25%) species. Despite recommended antibiotic prophylaxis, the incidence of postoperative pneumonia is still high, and no defined guidelines are available for noncardiac thoracic surgery.23 First and second generation cephalosporins are commonly used in the United States to prevent wound infection, empyema, and pneumonia. While they are extremely efficacious against the former, controversial results exist for the latter.23 In most of the cases the etiology for postoperative pneumonia favors gram negative microorganisms, which are susceptible to a broader coverage. Schussler et al24 studied 455 patients who underwent major lung resection and noted a decrease in postoperative pneumonia after changing the antibiotic regimen from a second generation cephalosporin (cephamandole) to high-dose amoxicillin-clavulanate. The study was reflective of a clinical practice change, and it was neither prospective nor randomized, making the conclusions difficult to apply in the United States, where first generation cephalosporins still remain the recommended antibiotic of choice, with vancomycin or clindamycin as an alternative for β-lactamase allergic patients.25 Current recommendations consist of a single dose antibiotic regimen at an appropriate dosage per body weight, with repeated administration intraoperatively if the wound is not closed after two half lives of the drug.25 Temperature control, supplemental oxygen, and avoidance of hyperglycemia are all additional maneuvers suggested to decrease the incidence of postoperative infections;25 however, their impact on postoperative pneumonia remains unclear.
BRONCHOPLEURAL FISTULA
Bronchopleural fistula (BPF) is defined as a communication between the bronchial lumen and the pleural space, and it can be confirmed via bronchoscopy, thoracotomy, or both.26 It occurs most commonly after right pneumonectomy, probably due to the length of the remaining bronchial stump (longer on the right side), especially if not covered with a flap.1,26 Its incidence is estimated to be from 0% to 9%, leading to a mortality rate of 16% to 23%. Local and systemic risks factors have been identified.26 Local risk factors include bronchial invasion by the tumor, length of the stump, integrity of blood supply, preoperative radiation, stump closure technique (manual suturing vs stapling) and extent of the resection. Extensive lymph node dissection has also been suggested as a cause for BPF formation.27 Systemic factors include patient age older than 70, male gender, diabetes, poor nutritional status, preoperative chemotherapy and underlying lung disease, COPD with low FEV1 and DLCO, and the presence of empyema.26 Common symptoms include cough, which may be productive and worsening when laying on the ipsilateral side of the fistula, or signs of infection if empyema is present. Conventional treatment consists of surgical repair with thoracoplasty and chest wall fenestration to allow drainage of the infected cavity and antibiotic irrigation.28 However, this procedure is associated with high morbidity and mortality rates, especially in the elderly and frail, with many of these patients being unable to have their thoracic fenestration closed. Minimally invasive bronchoscopic approaches have been investigated in an attempt to avoid a repeat thoracotomy.29 The studies published on this topic are mainly a summary of case reports, where the number of patients analyzed is still too small to allow any recommendations. Proposed procedures involve tracheo-endobronchial stent placement (mainly with metallic stents), fibrin glue occlusion, and scar tissue forming agents at the site of the fistula (Nd:YAG laser and sclerosing material). Fibrin glue use has been associated with 20% mortality, 35.6% rate of progression to surgical repair and 15.6% rate of chronic empyema. Better results seem to be achieved with synthetic glue, with a 67% resolution rate for the fistula and a survival rate of 83%. However, only 10% of the empyema seems to resolve with this technique.29 In the majority of cases, serial CT scans or bronchoscopic follow up are required, with repeated treatment being frequently necessary. Overall, prevention still remains the best treatment for BPF, focusing on modifying the potential risk factors when possible. Please refer to Chapter 18 for an in-depth discussion of the anesthetic management of patients undergoing bronchopleural fistula repair.
ACUTE LUNG INJURY
Acute lung injury (ALI) without an obvious etiology has been described after major lung resection in 1% to 3% cases.5,10 The incidence of ALI has significantly decreased in recent years, mainly due to an improvement in postoperative management and analgesic techniques. As a result, recent efforts have been directed toward understanding the pathophysiology and prevention of this serious complication.
According to the guidelines of the American-European Consensus Conference on ARDS, acute lung injury is defined as an acute onset of hypoxia with an abnormal PaO2/FiO2 ratio (usually <300) and radiographic infiltrates that are characteristic of pulmonary edema.30 ALI can occur either in the early (day 0-3) or late (day 3-10) postoperative period.31 The former is usually associated with PPPE, while the latter is associated with postoperative pneumonia or aspiration.
The strongest predictors for post-thoracotomy ALI seem to be related to patient characteristics (severe pulmonary disease, alcohol consumption) and perioperative medical care (extended resection, ventilator-induced lung injury and fluid overload).10,32 Recent focus has been directed toward one-lung ventilation strategies, with the goal of avoiding alveolar hyperinflation, alveolar stretching, and enhanced release of proinflammatory mediators. A retrospective study on 146 patients who underwent pneumonectomy showed that high tidal volumes and peak airway pressures during one-lung ventilation were associated with an increased incidence of postoperative ALI/ARDS.33 These findings were true both for healthy patients and those with decreased preoperative pulmonary compliance.34 Several animal models have demonstrated an increased systemic inflammatory response in the lung when high tidal volumes and plateau airway pressures were used, leading to increased mortality.35
Overall, once post pneumonectomy ALI occurs, hospital length of stay is prolonged and in-hospital mortality increased.10,33 Treatment is mainly supportive, focusing on mechanical ventilation with low tidal volumes (4-6 cc/kg predicted body weight), plateau airway pressures less than 30 cm H2O, respiratory rates titrated to maintain pH between 7.3 and 7.45, and an appropriate FiO2 and PEEP to achieve adequate oxygenation (O2 saturations of 88%-95%).35 As a consequence of the low minute ventilation, moderate hypercapnia may occur. This is usually well tolerated unless metabolic acidosis is also present, which may require an increase in respiratory rate and the use bicarbonate infusion. Recruitment maneuvers should be done intermittently and held for 30 seconds, as they may cause significant concomitant hypotension, limiting peak airway pressures to 35 cm H2O. Prone positioning may be considered as a short-term rescue treatment in the ICU setting in case of persistent hypoxia despite high FiO2 (>60%) and plateau pressures (>30 cm H2O). In selected patients, oxygenation may improve. However, multiple studies have shown no effect on mortality, which still remains elevated.35 The physiologic mechanisms by which the prone position may improve oxygenation are still unclear. Alveolar recruitment, redistribution of ventilation toward areas that have better perfusion, and elimination of cardiac compression by the lungs are suggested hypotheses.35 Among the risks of prone positioning are dislodgment and occlusion of the endotracheal tube and pressure ulcers.35
Several randomized trials have shown a reduction in the duration of mechanical ventilation in patients who have been able to tolerate trials of spontaneous ventilation on a daily basis.35 Patients who breathed unassisted for 30 to 120 minutes a day were able to be extubated earlier than those on pressure support or assist control ventilation. Ventilation with a T-piece, continuous positive airway pressure or 7 cm H2O of pressure support may be used if patients meet certain criteria, such as PEEP less than 8 cm H2O and FiO2 less than 50%, hemodynamic stability and the ability to initiate respiratory efforts.35 Moreover, trials of spontaneous ventilation paired with light sedation or wakeup periods are associated with earlier extubation rates, shorter ICU and hospital stay and decreased mortality rates.36
Several pharmacological interventions have been studied in addition to the protective lung ventilation strategies discussed above. However, the results are not too promising. Nitric oxide, prostaglandins and prostacyclins, surfactants, lisophylline, ketoconazole, and immuno-nutrition with fish oil have all been used successfully in animal models but have not shown the same positive results in clinical studies.37 Novel strategies under investigation target the stimulation of proteins in the alveolar epithelium to enhance edema clearance, the proliferation of type 2 pneumocytes to repair damaged alveoli, and the use of anticytokine antibodies to target inflammatory mediators.37
The role of corticosteroids in ALI/ARDS patients is still controversial in terms of morbidity and mortality, especially when high doses are used (30 mg/kg/d of methylprednisolone or equivalent).38 A meta-analysis of 5 cohort and 4 randomized controlled studies (a total of 648 subjects) favored the use of low dose methylprednisolone or equivalent (0.5-2.5 mg/kg/d) in the early stage of the disease, leading to a decreased mortality rate and improved morbidity.39 Similar results were found when more than a 7-day course was investigated, even though the sample size analyzed was small.38 Corticosteroid treatment needs to be initiated prior to the onset of the end-stage fibrosis, usually occurring within two weeks of diagnosis, and weaning should be gradual to prevent rebound inflammation. In the early phase, the disease is characterized by an intense inflammatory response, both generalized and local, with an increase in cytokines and chemokines, alveolar membrane disruption, and fibrogenesis.38 An abnormal pathway in the proinflammatory response involving glucocorticoid receptors has been identified both in the systemic and pulmonary circulation, explaining a potential rationale for corticosteroid treatment.38 A high index of suspicion for side effects is needed when steroids are used for a prolonged time, since nosocomial infections in the absence of fever and prolonged neuromuscular weakness may be quite common in the ICU setting.38
PROLONGED MECHANICAL VENTILATION AND THE NEED FOR TRACHEOSTOMY
Tracheostomy is usually performed in patients requiring prolonged mechanical ventilation or presenting with upper airway obstruction.40,41 Despite being a procedure that is widely performed in ICU patients, the indications, timing and choice of technique remain controversial.42 It seems that a consensus is reached only for mechanical ventilation longer than 3 weeks, as reported in a recent survey sent to several ICUs in France,40 even though another French study suggested 7 days as optimal.42 Some studies suggest an improved survival when tracheostomy is performed early, but the results are still controversial partly depending on patient risk factors such as age, neuromuscular status and COPD.42
Several advantages and disadvantages of tracheostomy were reported by the physicians responding to the aforementioned survey, the main advantage being easier weaning from the ventilator, better patient comfort and tracheal toileting, and the ability to take oral nutrition.
Among the complications of tracheostomy, tracheal injury and stomal infection were most commonly listed. See Table 23–3 for a summary of the indications, contraindications, and complications of tracheostomy. Tracheostomy can be performed surgically in the operating room, or percutaneously at the bedside in appropriate candidates. Percutaneous tracheostomy offers additional advantages, such as the fact that it is done at the bedside, eliminating the need for transport, which can be labor intensive and risky, especially if the patient is unstable. By eliminating the need for operating room time and personnel, the overall costs of the procedure are significantly decreased.43 In a survey of Dutch ICUs, the lack of operating room availability was listed in 9.1% cases as a reason for delaying tracheostomy, only preceded by the absence of a surgeon (11.4%).44,45 This caused a delay of 2 to 3 days from the time the decision of performing a tracheostomy was made. The procedure is relatively contraindicated in patients with adverse anatomical conditions such as short, fat neck, or obesity an enlarged thyroid gland, an inability to extend the neck, including either documented or suspected cervical spine fracture, previous cervical spine surgery or tracheostomy, coagulopathy, and anticoagulation therapy. Ben Nun et al46 reported a series of 157 patients who underwent percutaneous tracheostomy at the bedside, 58 of which had 1 or more relative contraindications. The incidence of short- and long-term complications for both percutaneous and surgical tracheostomy patients was similar, provided the procedure was performed by experienced personnel. The authors concluded that the only true contraindication for the percutaneous approach was the pediatric population because of the limited experience, agreeing with the majority of the experts in the field. A retrospective study conducted in Brazil showed similar results in terms of complications, when the procedure was performed by surgical residents supervised by a thoracic surgeon, and the patient population had no contraindications for the percutaneous approach.47
Table 23–3. Indications, Contraindications, and Complications of Tracheostomy41,43,44
POD 2: Intravenous loop diuretics were administered on POD 1 with a modest response but his creatinine rose to 3.1 mg/dL. The diuretics were discontinued later.
RENAL COMPLICATIONS
Acute renal failure (ARF) after lung resection is an uncommon complication and usually occurs as a result of infection or sepsis.48 Despite its low incidence (0.4%-1.0%),5,48,49 it is associated with a 60% to 90% mortality rate.45,48,50 Although the etiology is unclear, fluid restriction, sepsis, nephrotoxic agents, cardiogenic shock and tumor embolization have all been suggested as predisposing factors.51 Kheterpal et al49 in an observational study conducted on 75,952 patients undergoing noncardiac and nonvascular surgery found that age, male gender, diabetes mellitus, either on oral medications or insulin, acute heart failure, ascites, hypertension, and renal insufficiency were all associated with an increased risk of postoperative renal injury. Interestingly enough, low urinary output was not associated with ARF. This is in contrast with common clinical practice where a “good” urinary output is indicative of preserved renal function and a guide for fluid management. In a small study, Golledge et al52 found that ARF significantly increased morbidity and mortality after thoracic surgery. Hospital length of stay was 50% longer and mortality 19% higher. They proposed similar risk factors to those described above. Systemic hypotension, which can occur with the use of epidural analgesia, was also suggested as another cause for ARF, especially if local anesthetics were used at high concentration or volume. In healthy subjects, thoracic epidural analgesia interferes with the renin-angiotensin-vasopressin system. Sharrock et al53 in a retrospective study of 150 patients undergoing total hip replacement showed that hypotensive epidural anesthesia did not increase the incidence of postoperative ARF even in patients with preoperative renal disease. In that study, mean arterial pressure was kept at about 40 to 55 mm Hg for an average of 95 minutes, and low dose epinephrine infusion was used to maintain cardiac output. Patients with renal disease were rehydrated during surgery more liberally than the controls, and maybe this contributed to prevent long-term renal disease. As reassuring as these results may be, it must be kept in mind that this model does not completely apply to the thoracic population. Thoracic epidural analgesia is commonly used intraoperatively, but with lower concentrations of local anesthetic and with the goal of keeping the hemodynamics as close as possible to baseline. Rehydration is not used liberally, and average blood loss is usually less than 500 mL.
Renal failure is classified as oliguric (<400cc/d) or nonoliguric (>600 cc/d), the latter being easier to treat and associated with a better prognosis.48,54 Other than supportive care, volume expansion and hemodialysis are the suggested treatments. However, volume expansion is poorly tolerated in the thoracic population, especially after pneumonectomy, due to the potential risk of pulmonary edema, and the use of diuretics can potentially cause harm, thus is not routinely recommended. Maintenance of tissue oxygenation, treatment of sepsis, nutritional support and some form of dialysis and filtration (continuous arteriovenous hemofiltration in case of volume overload, continuous arteriovenous hemodiafiltration for hyper-kalemia, acidosis, or progressive azotemia) have also been suggested.48
Nonsteroidal anti-inflammatory drugs (NSAIDs) are usually used as an adjunct to intravenous narcotics or epidural analgesia in the postoperative period, and may potentially cause ARF due to their nonspecific inhibition of cyclooxygenase enzymes.55 By decreasing prostaglandin production, NSAIDs decrease pain and inflammation, but may also cause hypertension, gastrointestinal hemorrhage and renal dysfunction. The analysis of the literature is inconclusive on the role of these medications on perioperative renal function. In patients without renal disease, NSAIDs may reduce creatinine clearance, as well as potassium and sodium elimination on postoperative day 1. However, no effect on urinary volume or need for hemodialysis has been described.55–57 Preexisting renal disease as well as dehydration (or fluid restriction) may play a role in the development of renal insufficiency related to NSAID use, especially in patients with chronic renal failure, where residual function is prostaglandin dependent. When NSAIDs and aminoglycosides are administered together, there is an additive increased risk of developing ARF, even in the presence of normal preoperative renal function.57 Cyclooxygenase (COX) inhibitors, especially COX2, represent a potentially safer alternative to non specific NSAIDs, especially in the presence of preexisting renal dysfunction. However, a review of the literature has produced inconsistent results to be able to provide recommendations.57 Moreover, these drugs are available only in the oral form in the United States, making their use difficult in the immediate postoperative period.
Several medications are still used for renal protection, however the data in the literature is extremely controversial in terms of whether they improve outcome (summary in Table 23–4).
Table 23–4. Possible Pharmacologic Treatment of Acute Renal Failure
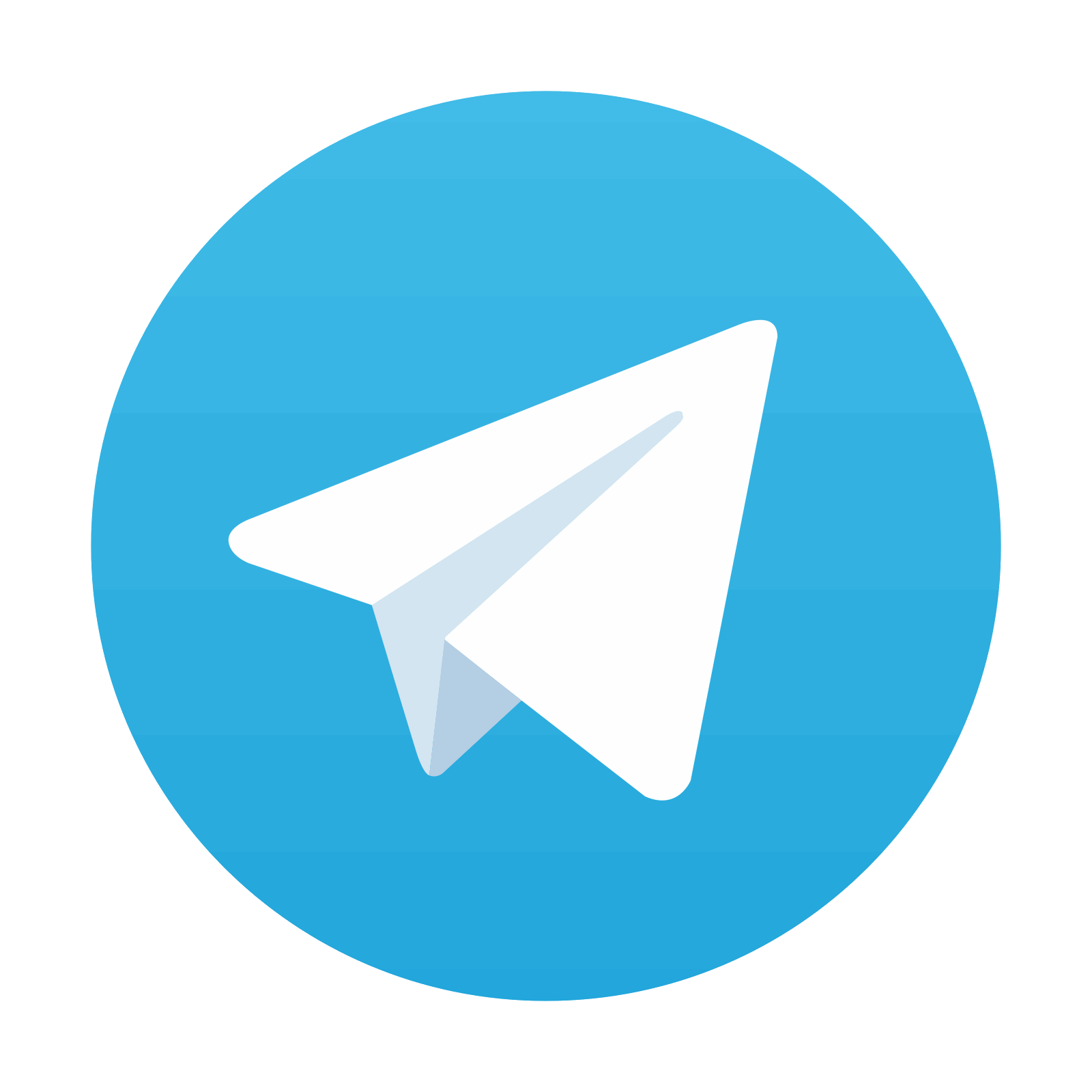
Stay updated, free articles. Join our Telegram channel

Full access? Get Clinical Tree
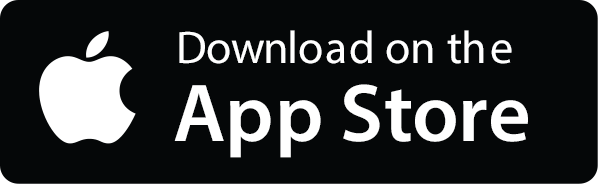
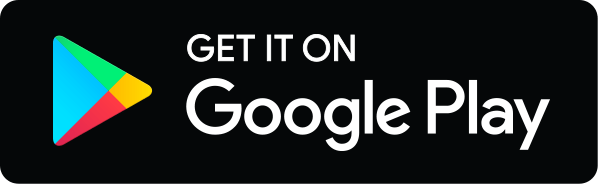