Mr. S is a 52-year-old entrepreneur in the waste management industry. He weighs 119 kg, is 175 cm tall (BMI of 38.9 kg·m−2), and is being investigated for “dizzy spells.” His medical problem list includes obesity, untreated hypertension, and possible obstructive sleep apnea (OSA) (based on his wife’s observation that “sometimes he just stops breathing” at night). A previous attempt at a magnetic resonance imaging (MRI) scan was unsuccessful because Mr. S, startled by the onset of the loud noises made by the MRI machine, panicked and tried to get out of the MRI scanner. Upon further questioning, he admits to extreme claustrophobia, possibly the result of a protracted period of time spent in a car trunk as a child.
On this occasion, the MRI team decides that Mr. S might be more cooperative with pharmacologic assistance and to this end has given him 5 mg of IV midazolam (Versed®). Unknown to the clinical team, just before arriving at the MRI suite, Mr. S had also taken 6 mg of his wife’s lorazepam (Ativan®) to help reduce his considerable anxiety. For the scan, a pulse oximeter and nasal capnograph are used to monitor respiration. Oxygen is administered by nasal prongs at 3 L·min−1.
About 10 minutes into the MRI scan, the pulse oximeter alarm activates, drawing attention to an oxygen saturation reading of 81%. The pulse oximeter waveform quality appears to be good. However, no waveform can be obtained from the capnograph. Since Mr. S is deep inside the MRI machine, it is difficult to visually assess his respiratory status. You are urgently summoned to the MRI suite by the radiology team to help manage this patient.
A basic understanding of how MRI works is important for both medical management and the safety of both patients and medical personnel in the MRI suite. In simple terms, MRI systems use high-strength magnetic fields and radio waves to generate images based on interactions between the generated magnetic fields and hydrogen molecules in the tissues being imaged. More specifically, static magnetic fields generated by an MRI scanner interact with small fields generated by atomic nuclei. Some nuclei develop a magnetic dipole moment when subject to this field, deflected at a slight angle to the static magnetic field. These nuclei also “wobble” about the direction of the magnetic field much like a spinning “top” at what is known as the precession frequency, which is proportional to the magnetic field strength. When a variable magnetic field is applied at the precessional frequency, more nuclei move from a lower to higher energy state. When the variable field is removed, they relax, emitting energy at the same precessional frequency which is then detected by a receiving coil in the scanner. Medical grade MRI scanners are typically tuned to interact with hydrogen and water, while the receiving coils detect energy in the radiofrequency range (RF). Thus images are generated by small spatial gradient (variable) field perturbations that localize individual tissues which then emit RF signals detected by the receiving coil and reconstructed with a computer in 3D space.
Modern MRI scanners typically use static field strengths from 0.5 to 3 tesla (T) with many research machines now producing 4 T. Most diagnostic scanners operate at 1.5 T. For perspective, 1 T = 10,000 gauss (G), and the earth’s magnetic field is approximately 0.5 G. A cylindrical bore at the center of the scanner is surrounded by a superconducting solenoid housed in liquid helium. Field strength is strongest within bore of the scanner and expressed in tesla. Fringe fields extend outward from the scanner with shape dictated in part by the magnet design and by shielding, typically expressed in gauss (Figure 60–1).1,2
FIGURE 60–1.
Unshielded and passively shielded field strength contours from a 1.5 T MRI extending radially and axially from magnet bore. (Reproduced with permission from Bushberg J, Seibert J, Leidholdt E, Boone J. The Essential Physics of Medical Imaging. 2nd ed. Philadelphia, PA: Lippincott Williams & Wilkins; 2002.)

Intraoperative magnetic resonance imaging (iMRI) systems are a more recent tool for both diagnostic and therapeutic interventions. They can be used for preoperative functional brain mapping of eloquent brain areas as well as for real-time brain mapping for invasive neurosurgical procedures. Examples include monitoring progressive changes in the lesion and surrounding tissue, or updating brain mapping by accounting for brain shift after opening up the dura.3
The MRI suite is organized into four concentric “zones” corresponding to increasing level of hazard (Figure 60–2). Zone I includes all remaining zones within, and is the area where patients and health care provides must gain access to the MRI suite. It is generally publicly accessible. Zone II is a controlled area restricting access to Zone III. Patients are interviewed and checked in here and under the supervision of MRI personnel. Zone II must have a ferrous metal detector as well as a 1000 G handheld magnet to screen equipment and patients entering the MRI suite. Zone III requires complete screening from Zone II of all personnel and patients as any contraindicated equipment and devices may interact with the MRI static and time-varying magnetic fields causing serious injury or death. Zone IV is contained within Zone III, and is the room containing the MRI magnet itself. Zone IV is heavily restricted and under full supervision by MRI suite personnel.4 Entrances to all zones should be appropriately labeled and controlled, and it is in Zones III and IV where the anesthesia practitioner must consider the safety restrictions of the MRI magnetic fields when formulating an anesthetic plan.
FIGURE 60–2.
Diagram illustrating the four zones of the MRI suite. All patients and staff must enter through Zone I and undergo appropriate screening in Zone II before entering Zones III and IV. Certain MRI conditional equipment may enter Zone IV but must be outside the appropriate 5 or 300 G contour as designated by magnetic screening or the manufacturer. A predetermined location in Zone III must be readily available to move the patient in the event of emergency such as cardiopulmonary collapse.

The physics of MRI dictates identification of unique patient and equipment considerations when managing cases in the MRI suite. Not only can the strong magnetic fields directly affect patient physiology such as vestibular function, hearing, and interaction with ferromagnetic prostheses, they also create numerous safety concerns with how and what equipment is used. Electronic interference leading to equipment malfunction, metallic projectiles from strong magnetic fields, and limited patient access are a few examples and represent occupational health and safety concerns that must be identified and mitigated. As long as these hazards are understood and appreciated, the anesthesia practitioner can safely provide anesthesia even within Zone IV of the MRI suite while image requisition is taking place.
MRI scanners employ a static magnetic field within Zone I that may always be on between cases. Within the 30 G field line, any ferromagnetic objects may be drawn toward the center of the bore as well as experience a torque in order to line up with the magnetic field. It should be common practice to fully screen patients and staff before passing through the 5 G contour (marked on the floor of Zone I) of the static field.1 These fields may interact directly with patients and health care workers as well as with surrounding objects and equipment. Occupational health surveys of factory workers in a 1.0 and 1.5 T MRI magnet manufacturing facility reported transient symptoms of headache (27%), vertigo (22%), and metallic taste (11%). Median magnetic field exposure time for these symptoms was as short as 20 minutes.5
Displacement, twisting, or migration of ferromagnetic aneurysm clips with the magnet field in the MRI suite6 has resulted in fatal outcomes in prior case reports in the literature. Specific metal alloys containing alpha phase iron such as in stainless steel have been implicated in reacting with strong magnetic fields. A recent literature review discussed the evolution of aneurysm clips toward MRI compatibility; however, current recommendations assume contraindication if there is any doubt about compatibility.7
The dangers of these strong magnetic fields in the MRI suite cannot be understated. In July of 2001, a 6-year-old child undergoing MRI suffered a skull fracture and intracranial hemorrhage from a projectile oxygen tank that had errantly been brought into the room. The child had received more sedation than necessary and became bradypneic. The anesthesiologist noted there was no flow through the wall oxygen supply, and while technologists sought a solution, a nurse handed the anesthesiologist an oxygen tank. They brought this tank into the MRI suite, and it was drawn with great force toward the magnet bore, hitting the child and causing the skull fracture. He passed away 2 days later from this injury.8,9 There are multiple case reports of such accidents or near misses during the last two decades.6,8,10–13
Screening of equipment for ferromagnetic materials may be performed onsite with handheld rare earth magnets of 1000 G or greater. They can be used to detect even weakly ferromagnetic equipment by assessing if there is any perceptible force interaction between the rare earth magnet and equipment being tested. However, equipment with no apparent ferromagnetic material after magnetic screening may still pose a threat. Zimmer et al.11 describe a case report of a young boy under general anesthesia who underwent abdominal MRI. A portable sevoflurane vaporizer was brought into the MRI suite and subsequently was attracted to the magnet. Two individuals were able to deflect the trajectory of the vaporizer so that it struck the MRI gantry and not the child within the magnet bore. Although an emergency quench of the magnet was considered (described below), the vaporizer was ultimately removed with the help of three individuals. A strong handheld magnet showed no attraction to the vaporizer. However, when investigated further it did contain small amounts of ferromagnetic material within the temperature compensation module. Similar to the principle stated above regarding aneurysm clips, any equipment with unknown MRI compatibility should be considered dangerous until its safety has been definitively confirmed.
Low-amplitude magnetic fields that vary throughout the MRI imaging sequence can interact with equipment and the patient. These fields can induce current in coiled wire, such as with ECG leads, creating current and heat strong enough to cause tissue burns.1 These fields have also been shown to stimulate peripheral nerve and muscle cells.1 Humans, mice, and zebra fish have all demonstrated behaviors implicating peripheral vestibular stimulation while subject to strong static and changing magnetic fields. One such theory involves a Lorentz force interacting with natural ionic currents flowing within the inner ear endolymph to vestibular hair cells sufficient to displace semicircular canal cupula and induce vertigo and horizontal nystagmus in humans and mice.14 Another theory implicates electromagnetic induction, where currents can be induced within the body by changing magnetic fields directly stimulating neural tissue.14 Even transient visual disturbances described as flashes of light called phosphenes have been reported in research grade (4–7 T) scanners.14 At present there is no evidence to suggest these sensory effects are harmful to the patient. However, there are case reports of acute vertigo experiences by anesthesia practitioners even in 3 T MRI scanners.15
Noise generated with switching of gradient fields is usually above 85 dB within the MRI suite. Exposure to these noise levels can contribute to hearing loss for both staff and patients (awake or under general anesthesia) and ear protection is necessary.1 Additionally, the noise can be a distraction from monitor alarms and during emergency situations, inhibiting communication between staff.
Power dissipation from the strong radio transmitter can interact with patients’ tissues leading to increases in temperature and have the potential to burn the patient. Although the RF power of the MRI is monitored, other environmental factors can contribute to temperature changes, such as ambient temperature, airflow, humidity, and clothing.1 Additionally, any conductive metal material can rapidly heat causing burns on the patient. Case reports implicate induced thermal burns from circular metal implants, tattoos, deep brain stimulators, halo pins, ECG leads, coiled wiring, and transdermal patches. Studies have shown temperatures as high as 63°C can be achieved. These burns can be dramatic, and have, for example, resulted in full thickness burns leading to amputation in a child.10
In extreme situations that require an emergency magnetic field shutdown, a process called a quench can be performed. The super conductor that maintains the static magnetic field is housed in approximately 1000 L of liquid helium near absolute zero. During a quench, this helium is rapidly vaporized and expands, rapidly disabling the magnet with subsequent dissipation of the magnetic field. All MRI suites should be designed to rapidly vent the helium vapor; however, if there is a failure or blockage of the vented vapors then a hypoxic mixture can result within the magnet bore. Oxygen sensors and backup venting strategies should also be in place.1 Extra oxygen face masks or nasal prongs compatible with the suite should be available to connect to MRI safe wall oxygen or tanks outside Zone IV in the event the patient or other personnel are subjected to this hypoxic environment. It should be noted that a magnet quench can very easily cause damage to the magnet coil and it can take on the order of weeks to months before the MRI is ready for imaging. As such, the decision to quench is not taken lightly but is necessary when patient safety is at risk.
While there are few case reports on MRI quenches, one incident led to a quench when a physician carried an oxygen cylinder into Zone IV to treat hypoxemia in an overly sedated patient.12 Another involved a failed quench when a replacement oxygen H-cylinder was brought into Zone IV without adequate screening or signage.13 In both cases, the cylinders became projectiles, causing damage to the MRI machine and in the latter case, the patient suffered severe facial fractures. These cases highlight the importance of having MRI-compatible supplemental oxygen strategies for patients in Zone IV. Current American Society of Anesthesiology guidelines additionally recommend available supplemental oxygen for health care providers, helium venting strategies, and a plan for removal of the patient from Zone IV, in the event of an initiated quench.4
The nature of how equipment interacts with the static and time-varying magnetic fields generated by the magnet coils dictates safety and compatibility in the MRI suite. Equipment is broadly categorized into physiologic monitors, invasive monitors, intubation equipment, and oxygenation and ventilation equipment.4 For each category, equipment is further stratified between “Acceptable,” “Unacceptable,” and “Cautionary” with regards to safety in the MRI suite. It is important to note that even “MRI acceptable” equipment may only be rated for use up to a maximum magnetic field strength. Other “Cautionary” equipment may only be suitable for use in Zone III. It is up to the anesthesia practitioner and radiology department to be knowledgeable in the limitations of the equipment stocked and available for use in the MRI suite.4 A broader reference on equipment and monitoring is available in the Practice Advisory on Anesthetic Care for Magnetic Resonance Imaging by the American Society of Anesthesiologists, revised in 2015,4 however the focus of this chapter will be on airway equipment and management.
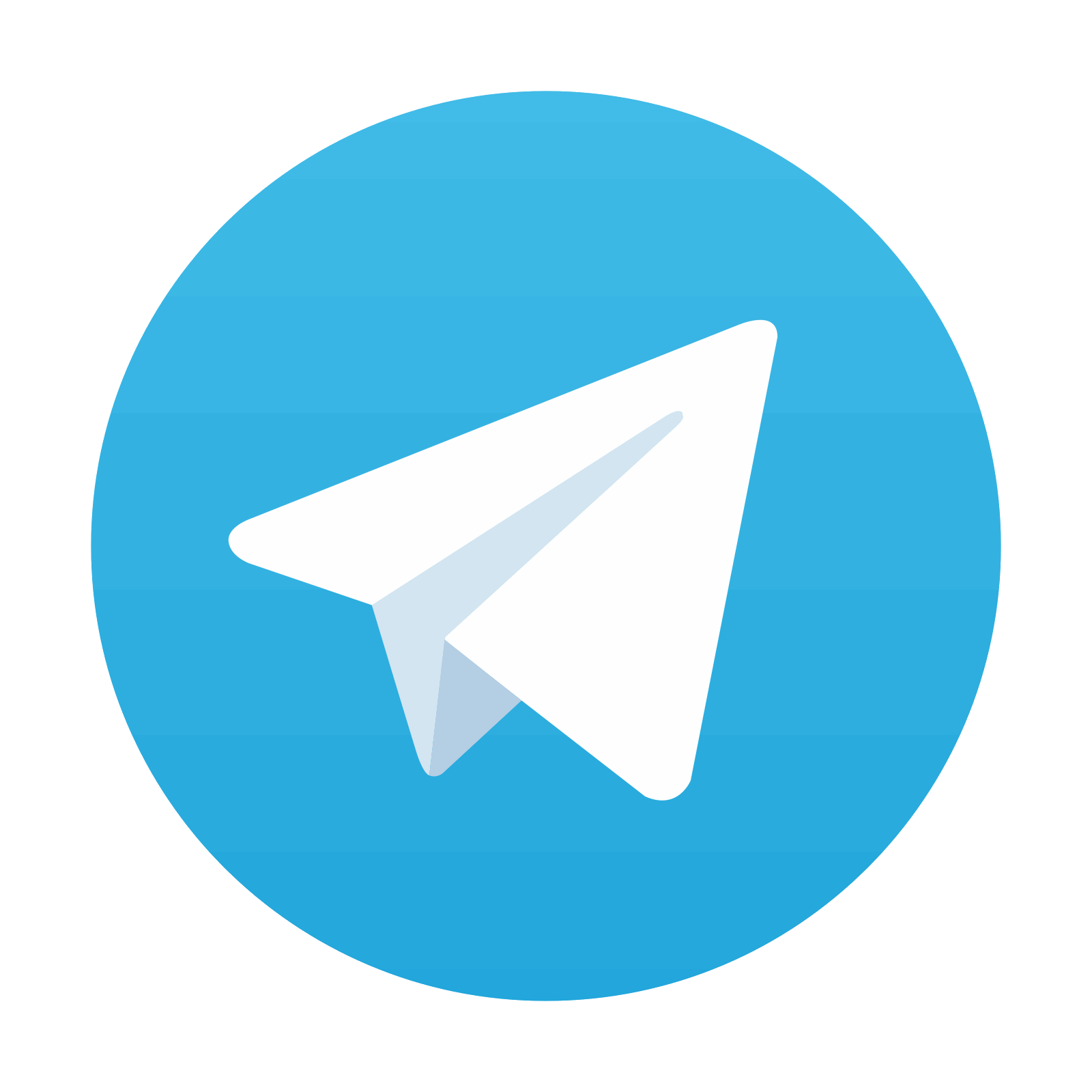
Stay updated, free articles. Join our Telegram channel

Full access? Get Clinical Tree
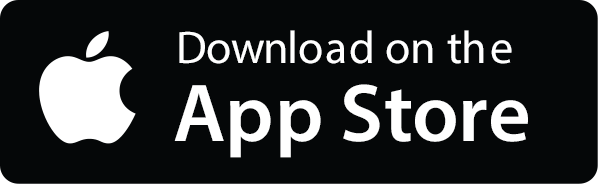
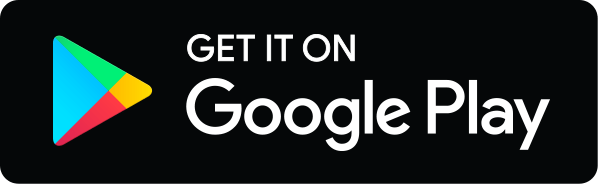