html xmlns=”http://www.w3.org/1999/xhtml” xml:lang=”en”>
Acute Respiratory Distress Syndrome
Ann Marie LeVine, MD, FCCM
Objectives
- Describe the pathophysiological process of acute respiratory distress syndrome
- Review ventilatory strategies for acute respiratory distress syndrome
- Discuss therapies for treatment of acute respiratory distress syndrome
Key words: acute respiratory distress syndrome, prone, corticosteroids, surfactant, nitric oxide
Definition of ARDS
Acute respiratory distress syndrome (ARDS) refers to the syndrome of lung injury characterized by dyspnea, severe hypoxia, decreased lung compliance, and diffuse bilateral infiltrates. Before 1992, the term ARDS referred to the adult respiratory distress syndrome. In 1994, the American–European Consensus Committee on ARDS standardized the definition of the syndrome and renamed it acute rather than adult respiratory distress syndrome since all age groups can acquire the disease. The term acute lung injury (ALI) was also introduced at this time. The committee recommended that ALI be defined as “a syndrome of inflammation and increased permeability that is associated with clinical, radiologic and physiologic abnormalities that cannot be explained by left atrial or pulmonary capillary hypertension.” The distinction between ALI and ARDS is the degree of hypoxemia, defined by the ratio of partial pressure of arterial oxygen (Pao2) to fraction of inspired oxygen (FIO2); ALI is indicated by Pao2/FIO2 less than 300 mm Hg and ARDS by less than 200 mm Hg.1
Risk Factors
Multiple risk factors have been associated with the development of ARDS and are listed in Table 3-1
Table 3-1. Clinical Conditions Associated With the Development of Acute Respiratory Distress Syndrome | |
Direct Lung Injury | Indirect Lung Injury |
Aspiration of gastric contents | Sepsis |
Pulmonary contusion | Severe trauma |
Fat emboli | Cardiopulmonary bypass |
Near drowning | Drug overdose |
Pneumonia | Acute pancreatitis |
Reperfusion edema (post transplant) | Multiple blood transfusions |
Inhalational injury |
Pathogenesis
Acute respiratory distress syndrome is characterized by an initial insult that triggers cell-mediated mechanisms, releasing a cascade of a variety of mediators. These mediators disturb the integrity and function of the cellular lining of the alveolar–capillary unit. Hyaline membranes, alveoli flooded with protein-rich edema fluid, infiltrates of polymorphonuclear neutrophils, macrophages, and erythrocytes are histological characteristics of ARDS. Proinflammatory mediators are released from lung alveolar and endothelial cells. The degree of inflammation depends on the biological activity and the imbalance between pro- and anti-inflammatory cytokines.
Inflammatory mediators also influence the coagulation cascade in ARDS. Activation of procoagulation factors (tissue factors) and inhibition of fibrinolysis (plasminogen activation inhibitor) produce platelet–fibrin thrombi in small pulmonary vessels.2 This interplay occurs intra- and extravascularly and in the alveolar compartment, resulting in fibrin deposition and alveolar hyaline membranes. Surfactant function is inactivated by protein leakage, and surfactant production is reduced by alveolar type II cell damage.
In ARDS, the lungs progress through 3 phases: exudative, proliferative, and fibrotic. The overall disease progression can vary significantly between patients. The exudative phase is characterized by damage to the alveolar epithelium and vascular endothelium, resulting in leakage of water, protein, and inflammatory and red blood cells into the interstitium and alveolar space. Type I alveolar cells are irreversibly damaged and replaced by the deposition of proteins, fibrin, and cellular debris, which produces hyaline membranes. Damage to the type II alveolar cells contributes to surfactant loss and collapse of the alveoli. In the proliferative phase, type II cells proliferate with some epithelial cell regeneration, fibroblast reaction, and remodeling. Some patients progress to an irreversible fibrotic phase involving collagen deposition in the alveolus, vasculature, and interstitium with microcyst formation.1
Treatment
Nutrition
The importance of nutritional support in critically ill patients with ARDS cannot be overstated. This syndrome is characterized by a proinflammatory response associated with hypercatabolism that can lead to significant nutrition deficit. Nutrition support is necessary to prevent cumulative calorie deficit, malnutrition, loss of lean body mass, and deterioration of respiratory muscle strength. Early institution of enteral nutrition has been associated with modulation of stress and the systemic immune response as well as reduction in disease severity.3
Although the importance of nutrition in patients with ARDS is widely accepted, determining nutrition requirements is challenging. Indirect calorimetry is considered the gold standard for assessing energy requirements. When providing nutrition support therapy to critically ill children, clinicians must avoid underfeeding and overfeeding. Table 3-2 summarized the complications of underfeeding or overfeeding.
Table 3-2. Complications of Overfeeding and Underfeeding | |
Underfeeding | Overfeeding |
Decreased ventilatory drive | Hypercapnia |
Depressed respiratory muscle strength | Failure to wean from mechanical ventilation |
Failure to wean from mechanical ventilation | Electrolyte imbalances |
Immunosuppression | Hyperglycemia |
Poor wound healing | Poor wound healing |
Immunosuppression | |
Hepatic steatosis |
Persistent production of oxygen free radicals and arachidonic acid (AA)–derived mediators characterizes ARDS. These mediators induce lung inflammation, edema, and diffuse alveolar damage. The goal for treating children with ARDS is to modulate pulmonary inflammation and permeability to improve oxygen delivery. A diet enriched with eicosapentaenoic acid and γ-linoleic acid has been shown to modify the availability of AA in tissue and cell phospholipids as well as stimulate proinflammatory eicosanoid production from AA.4
The phase 3 ARDSNet EDEN–Omega Study evaluated early versus delayed enteral feeding and omega-3 fatty acid/antioxidant supplementation for treating patients (13 years old and above) with acute lung injury or acute respiratory distress syndrome. This phase III clinical trial was a prospective randomized trial of initial trophic enteral feeding followed by advancement to full caloric enteral feeding or a faster early advancement to full calorie enteral feeding. This trial was run simultaneously with a trial of ω-3 fatty acids, γ-linoleic acid, and antioxidant supplementation. The primary outcome was number of ventilator-free days and mortality before hospital discharge with unassisted breathing. The data safety and monitoring board performed an interim analysis and examined the data of the EDEN–Omega Study. The study was terminated for futility. The mortality rate at 60 days was significantly lower in the control group (16.3%) than in the experimental group (26.6%).4
Fluid Management
Pulmonary edema resulting from increased capillary permeability worsens as intravascular hydrostatic pressure increases and oncotic pressure decreases. The optimal fluid management for children with ARDS is unknown. A randomized study compared conservative and liberal strategies of fluid management for 7 days in patients with acute lung injury. The primary end point was death at 60 days, and secondary end points were the number of ventilator-free days and organ-failure free days. The primary end point, rate of death at 60 days, was 25.5% in the conservative strategy group and 28.4% in the liberal strategy group (P = 0.3). The conservative strategy improved the oxygenation index [(Mean Airway Pressure × Pao2/FIO2) × 100] and increased the number of ventilator-free days (14.6 ± 0.5 compared with 12.1 ± 0.5, P < 0.001). The results support the use of conservative fluid management in patients with acute lung injury to improve oxygenation, yet the effect on survival remains in question.5
Mechanical Ventilation
Mechanical stress on alveolar walls can produce several different consequences in injured lungs, including physical disruption of alveolar epithelial and endothelial cells, disruption of the alveolar wall basement membrane, and activation of stretch-response signaling pathways in the alveolar walls and airspace leukocytes, which produce inflammation and increase endothelial and epithelial permeability. In the NIH ARDSNet trial of 2 different mechanical ventilation strategies in patients with acute lung injury, the patients treated with lower tidal volumes had significantly more days alive and free of circulatory failure, renal dysfunction, and serious coagulation abnormalities.6 Reducing set tidal volume and using “lung-protective” ventilation in patients with acute lung injury is the recommended practice.
Prone Positioning
The prone position has been shown to induce an increase in both end-expiratory lung volume and alveolar recruitment. When a patient with ARDS is prone, the mass of the dorsal lung, which reinflates (ie, dorsal regions become the nondependent lung regions), is greater than the potential mass of the ventral (now dependent) lung regions, which may collapse. The overall ventilation perfusion matching improves as new pulmonary units are recruited for more effective gas exchange.7
A multicenter, randomized control clinical trial of supine compared with prone position in 102 pediatric patients failed to demonstrate a significant difference in its primary end point (ventilator-free days to day 28) or in any of its secondary end points.8 It remains to be determined whether there are select subpopulations of those with ARDS (ie, based on age or disease severity) who may benefit from prone positioning.
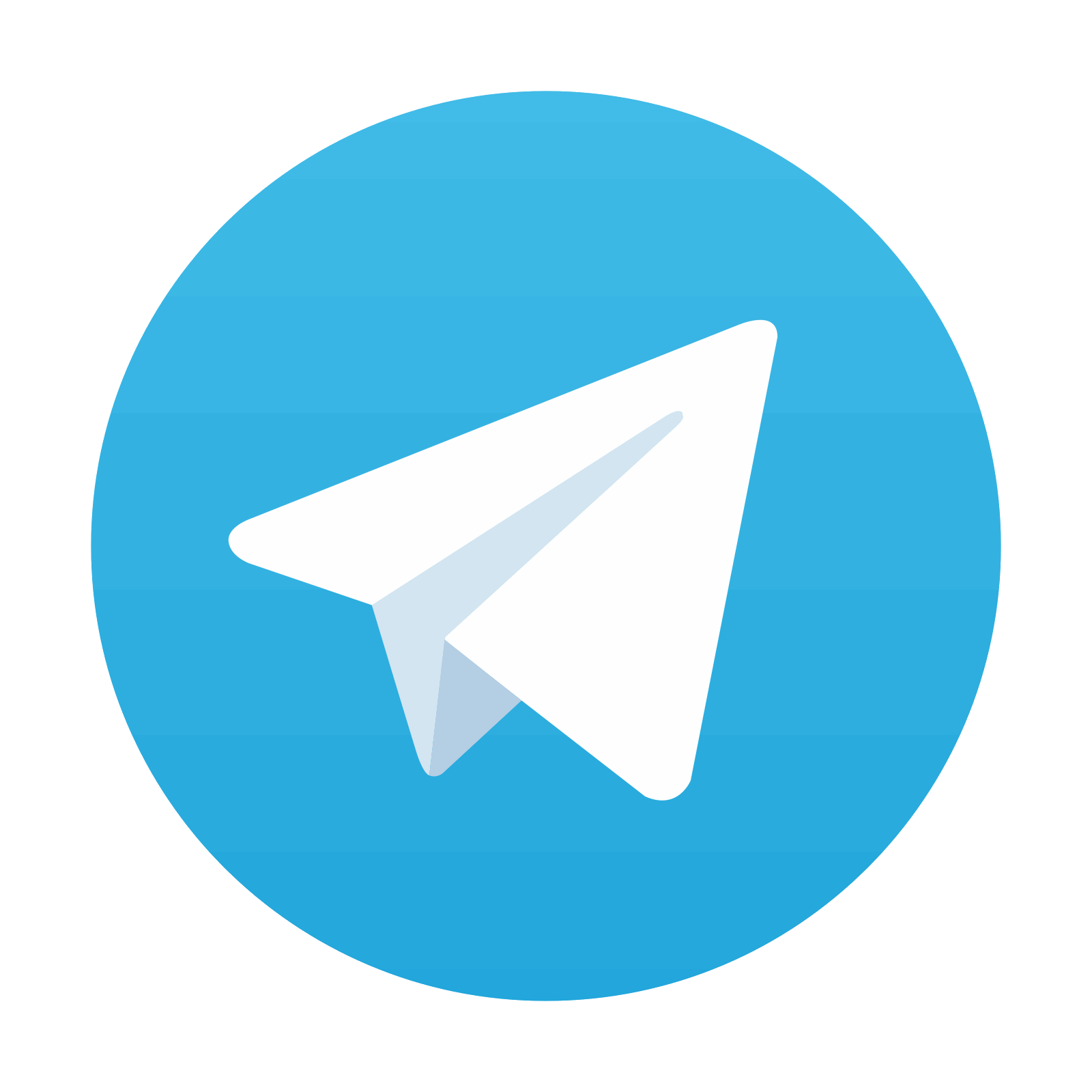
Stay updated, free articles. Join our Telegram channel

Full access? Get Clinical Tree
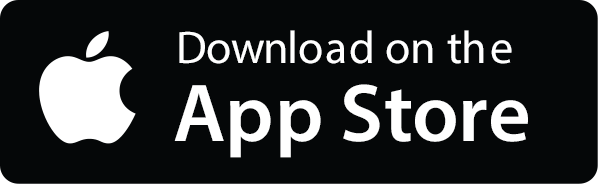
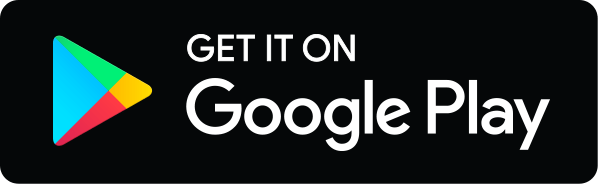