Respiratory Adjunct Therapy
Scott E. Kopec
Richard S. Irwin
Various adjunct therapies are available to aid in the management of critically ill patients with existing or anticipated pulmonary dysfunction. In this chapter, we review several adjunct therapies, emphasizing any randomized trials determining efficacy and indications. We will specifically discuss the following: (a) aerosol therapy and humidification; (b) lung expansion techniques; (c) airway clearance techniques; (d) administration of medical gases; (e) nasal continuous positive airway pressure (CPAP) and bilevel positive airway pressure for sleep-related breathing disorders; and (f) communication alternatives for the patient with an artificial airway. A discussion of the use of bilevel positive airway pressure to provide noninvasive ventilatory support can be found in Chapter 59.
Aerosol Therapy
An aerosol is a stable suspension of solid or liquid particles dispersed in air as a fine mist. Bland aerosols are generally used to humidify inspired gases. Aerosol drug therapy represents the optimal modality for site-specific delivery of pharmacologic agents to the lungs in the treatment of a number of acute and chronic pulmonary diseases. Due to the cost and potential hazards of aerosol therapy, use should be limited to aerosols whose clinical value has been objectively shown [1].
Bland Aerosols
Bland aerosols include sterile water or hypotonic, normotonic, and hypertonic saline delivered with or without oxygen. These are typically delivered via an ultrasonic nebulizer in an effort to decrease or aid in the clearance of pulmonary secretions. The routine use of bland aerosols in the treatment of some specific diseases has demonstrated mixed results. An evidence-based recommendation for the use of bland aerosols has recently been released by the British Thoracic Society (BTS) [2]. The use of bland aerosols in the treatment of chronic obstructive pulmonary disease (COPD) and croup appears not to be of any benefit [2,3]. For patients with cystic fibrosis (CF), the use of 7% (hypertonic) saline, administered twice daily, may result in a significantly higher forced vital capacity (FVC) and forced expiratory volume in 1 second (FEV1), and a decrease in the number of acute exacerbations when compared to the use of normotonic saline [4]. The use of nebulized saline or sterile water may improve sputum clearance in patients with non-CF bronchiectasis [2]. Delivery of bland aerosols is ineffective in liquefying secretions because sufficient volumes of water fail to reach the lower airways. Furthermore, bland aerosols may provoke bronchospasm and place patients at risk for nosocomial pneumonia [3,5].
Mist therapy, the delivery of a continuous aerosol of sterile water or saline, is frequently used to treat upper-airway infections in children, but has not been shown to be more effective than air humidification [3].
Humidity Therapy
Theoretic reasons for using humidified inspired gas are to prevent drying of the upper and lower airways, hydrate dry mucosal surfaces in patients with inflamed upper airways (vocal cords and above), enhance expectoration of lower-airway secretions, and induce sputum expectoration for diagnostic purposes [3]. Although adequate humidification is critical when dry medical gases are administered through an artificial airway (endotracheal or tracheostomy tube), there is little evidence to support the use of humidification in the nonintubated patient.
Humidity therapy is water vapor and, at times, heat added to inspired gas with the goal of achieving near-normal inspiratory conditions when the gas enters the airway [6]. Because adequate levels of humidity and heat are necessary to ensure proper function of the mucociliary transport system, humidification is imperative when the structures of the upper airway that normally warm and humidify inspired gases have been bypassed by an artificial airway. During mechanical ventilation, humidification is crucial to avoid hypothermia, atelectasis, inspissation of airway secretions, and destruction of airway epithelium because of heat loss, moisture loss, and altered pulmonary function [7]. Optimal humidification is the point at which normal conditions that prevail in the respiratory tract are simulated [8].
Several external devices are available to artificially deliver heat and moisture. Two such devices for mechanically ventilated patients are: (a) a heated waterbath humidifier, which is an external active source of heat and water, and (b) a heat and moisture exchanger filter (HMEF), which passively retains the heat and humidity, leaving the trachea during expiration and recycles it during the next inspiration. HMEFs are also known as hygroscopic condenser humidifiers or artificial noses. The HMEF is designed to combine air-conditioning and bacterial filtration. In a randomized controlled trial, both devices were shown to be equally safe [9]. Potential advantages of HMEFs over heated waterbath humidifiers include reduced cost and avoidance of airway burns and overhydration. A potential disadvantage is that resistance of airflow through an HMEF may progressively rise, increasing the work of breathing and conceivably impeding weaning from the ventilator [9].
Cold-water devices such as bubble humidifiers are frequently used to add humidity to supplemental oxygen administered to spontaneously breathing patients. Due to a lack of objective evidence to support the practice, the American College of Chest Physicians recommends elimination of the routine use of humidification of oxygen at flow rates of 1 to 4 L per minute when environmental humidity is sufficient [10], while the BTS does not recommend its use [2].
Patients requiring high flow rates of oxygen (> 10 L per minute) frequently develop discomfort due to upper-airway dryness. There are several devices available to deliver humidification via nasal cannulae at high flow rates (high flow oxygen delivery), including Vapotherm (Vapotherm, Annapolis, MD) and the Fisher & Paykel 850 (Fisher and Paykel Healthcare Corp, Auckland, New Zealand). Although these devices have been shown to improve patients’ comfort [11], we are not aware of any studies determining therapeutic benefits. Potential risk of exposure to Ralstonia spp in patients using Vapotherm has been reported [12], but by switching to disposable filters, the problem appears to have been addressed.
Pharmacologically Active Aerosols
Inhaled therapy has several well-recognized advantages over other drug delivery routes. The drug is delivered directly to its targeted site of action; therefore, when compared to other routes of administration, a therapeutic response usually requires fewer drugs, there are fewer side effects, and the onset of action is generally faster [13]. A broad range of drugs is available as aerosols to treat obstructive lung diseases. These include β-adrenergic agonists, anticholinergics, anti-inflammatory agents, and anti-infectives. Additionally, the inhaled route is used to deliver drugs that are not effective when delivered by the oral route (e.g., pentamidine) [14].
Although a variety of drugs are currently available in aerosolized form, dosing to the lung remains inexact because deposition is affected by several patient-, environment-, and equipment-related factors. Potential hazards of aerosol drug therapy include (a) a reaction to the drug being administered, (b) the risk of infection, (c) bronchospasm, and (d) the potential for delivering too much or too little of the drug [14]. With respect to the use of aerosolized ribavirin, there are potential hazards to healthcare providers administering the medication (see later).
Bronchodilators
There are two classes of inhaled bronchodilators: (a) β2-adrenergic receptor agonists (short-acting and long-acting) and (b) anticholinergic agents.
Short-Acting β2-Adrenergic Receptor Agonists
Although β1– and β2-adrenergic receptors are present in the lungs, β2-adrenergic receptors appear to be entirely responsible for bronchodilation. Therefore, β2-adrenergic receptor agonists (e.g., albuterol, pirbuterol, and terbutaline) are the agents commonly preferred for the relief of acute symptoms of bronchospasm. In addition to the bronchodilating properties of β2-adrenergic receptor agonists, other actions include augmentation of mucociliary clearance; enhancement of vascular integrity; metabolic responses; and inhibition of mediator release from mast cells, basophils, and possibly other cells [3].
Inhalation of β2-selective agonists is considered first-line therapy for the critically ill asthmatic [15] and COPD patient [5,16]. Although these agents can be administered orally, by inhalation, or parenterally, the inhaled route is generally preferred because fewer side effects occur for any degree of bronchodilation [3]. For most patients experiencing acute asthma attacks, inhalation is at least as effective as the parenteral route [3]. Inhaled β2 agonists can be delivered as an aerosol from a jet or ultrasonic nebulizer or from a metered-dose inhaler (MDI). The relative efficacies of the nebulizer and MDI are dependent on the adequacy of technique. Although it was formerly a standard practice to deliver bronchodilators by nebulizer, several prospective, randomized controlled trials have challenged this practice. Delivering β2 agonists by MDI with a spacer device (holding chamber) under the supervision of trained personnel is as effective in the emergency setting as delivery by nebulizer for adults and children [3]. In hospitalized patients, β2 agonists delivered by MDI are as effective as therapy with a nebulizer and can result in a considerable cost savings [3]. An analysis of 16 trials (686 children and 375 adults) to assess the effects of MDIs with holding chambers compared to nebulizers for the administration of β2 agonists for acute asthma concluded that MDI with a holding chamber produced at least equivalent outcomes as nebulizer delivery [17].
Ideal frequency of administration and dosing of β2 agonists has not been determined. For emergency department and hospital-based care of asthma, the National Institutes of Health Expert Panel Report 2 [15] recommends up to three treatments in the first hour. Subsequent treatments should be titrated to the severity of symptoms and the occurrence of adverse side effects, ranging from hourly treatments for moderate severity to hourly or continuous treatments for severe exacerbations. Recommendations for initial treatment of severe acute exacerbations of COPD are for the administration of short-acting β2 agonists every 2 to 4 hours if tolerated [5].
When given by jet nebulizer, the usual adult dose of albuterol is 0.5 mL of an 0.5% solution (2.5 mg) diluted in 2.5 mL of saline (or 3 mL of 0.083% unit-dose nebulizer solution). The frequency of dosing varies depending on the disease and the situation. It can range from every 4 to 6 hours in patients with COPD and stable asthma to every 20 to 30 minutes for six doses in patients with status asthmaticus [3]. In patients with acute asthma, albuterol solution has also been continuously nebulized for 2 hours [18]. In this randomized controlled trial of spontaneously breathing patients with FEV1 less than 40% predicted, continuous delivery of high-dose (7.5 mg per hour) or standard-dose (2.5 mg per hour) albuterol were both superior to hourly intermittent treatments with 2.5 mg in increasing FEV1. Although there was no difference in FEV1 improvement between the two continuous doses, the standard dose had fewer side effects.
Although the usual dosage of bronchodilator by MDI is two puffs (90 μg per puff) every 4 to 6 hours in stable hospitalized and ambulatory adult patients, the dosage must be increased up to sixfold in acute severe asthma to achieve results equivalent to those achieved with small-volume nebulizers [3]. In an emergency department treatment study of severe asthma, four puffs of albuterol by MDI every 30 minutes for a total of six dosing intervals (24 puffs) was found to be safe and equivalent to 2.5 mg of albuterol diluted in 2 mL of saline given every 30 minutes for six doses [3]. Others have treated acute episodes of asthma in the emergency department in a dose-to-result fashion as follows: initially four puffs by MDI of bronchodilator of choice, followed by one additional puff every minute until the patient subjectively or objectively improved or side effects (e.g., tremor, tachycardia, arrhythmia) occurred [3]. In mechanically ventilated patients, the bronchodilator effect obtained with four puffs (0.4 mg) of albuterol from an MDI with holding chamber is comparable to that obtained with 6 to 12 times the same dose given by a nebulizer and is likely to be more cost-effective [19].
Tremor is the principal side effect of β2 agonists, due to the direct stimulation of β2-adrenergic receptors in skeletal muscle. Tachycardia and palpitations are less frequent with the selective β2 agonists (e.g., albuterol) than with nonselective β1-β2 agonists such as isoproterenol. Although vasodilation, reflex tachycardia, and direct stimulation of the heart can occur even with the use of selective β2 agonists, cardiac adverse occurrences are uncommon when usual doses of inhaled β2 agonists are administered. A transient decrease in arterial oxygen tension may occur in patients with acute, severe asthma. This response is likely
due to the relaxation of the compensatory vasoconstriction in areas of decreased ventilation together with increased blood flow due to increased cardiac output [3].
due to the relaxation of the compensatory vasoconstriction in areas of decreased ventilation together with increased blood flow due to increased cardiac output [3].
β2-adrenergic agonists can cause acute metabolic responses including hyperglycemia, hypokalemia, and hypomagnesemia [3]. Although typically not seen in standard doses, if large and frequent doses of β agonists are given, electrocardiogram and serum potassium monitoring are indicated. After inhalation of 10- and 20-mg doses, the maximal decreases in potassium can be 0.62 ± 0.09 mmol per L and 0.98 ± 0.14 mmol per L, respectively [20].
Perinatal outcomes of 259 pregnant women with asthma who were treated with β2-adrenergic agonists during pregnancy were compared to those of 101 women who were not treated with these agents, and 295 nonasthmatic women [3]. There were no differences in perinatal mortality rates, congenital abnormalities, preterm delivery, low birth weights, mean birth weights, or the number of small-for-gestational-age infants. In addition, there were no differences in Apgar scores, labor or delivery complications, or postpartum bleeding.
Levalbuterol (Xopenex, Sepracor Inc, Marlborough, MA) inhalation solution, the (R)-enantiomer of racemic albuterol, is a relatively selective, third-generation β2-adrenergic receptor agonist approved for treatment of bronchospasm in adults and children aged 12 years or older. Levalbuterol appears to offer little benefit over albuterol in improving FEV1 in patients with asthma, and is not associated with any fewer systemic side effects such as tachycardia and hypokalemia [21]. For further discussion of aerosolized β agonists in asthma and COPD, see Chapters 48 and 49.
Long-Acting Inhaled β2 Agonists
Long-acting inhaled β2 agonists (e.g., salmeterol and formoterol) are currently not recommended for use in acute exacerbations of asthma (Expert Panel Report 2) [15] or COPD [5]. One prospective, double-blind, randomized, placebo-controlled trial demonstrated a possible role for salmeterol as an adjunct to conventional therapy for hospitalized asthmatic patients [22], but larger studies are needed to clarify whether there is a potential benefit in the setting of acute asthma. If patients are using these agents as controller medications for asthma or COPD and are hospitalized for other reasons, consider continuing them for asthma maintenance during the hospitalization. These agents should be administered at regular intervals; additional doses to relieve symptoms should not be prescribed.
Anticholinergics
Anticholinergics appear to have a role in acute asthma when combined with sympathomimetic drugs [3], in exacerbations of COPD when combined with albuterol [5], in intubated patients to prevent bradycardia induced by suctioning [23], and in selected patients with severe bronchorrhea [24]. Ipratropium bromide is dosed at 500 μg in 2.5 mL normal saline (1 unit 0.02% unit-dose vial) or two to six puffs by MDI (18 μg per puff) every 6 to 8 hours. Ipratropium (18 μg per puff) and albuterol (103 μg per puff) are available as a combined MDI product (Combivent, Boehringer, Ingelheim; Ridgefield, CT). Ipratropium by MDI can be given to ventilated patients with the same spacer device used for β-agonist delivery. Tiotropium, a selective muscarinic antagonist, is available in a dry powdered form. Its use should be limited to the chronic management of patients with COPD. For further discussion of anticholinergic use in asthma and COPD, see Chapters 48 and 49.
Combined Bronchodilator Therapy
Although inhaled short-acting β-adrenergic receptor agonists remain first-line agents in the treatment of acute asthma, the addition of ipratropium bromide may result in an added benefit [25]. Anticholinergics may be of benefit as additive agents or as single agents in situations in which the patient cannot tolerate β-adrenergic side effects. Both agents appear effective in smoking-related chronic bronchitis.
Mucolytics
N-Acetylcysteine
Theoretically, mucolytic agents facilitate expectoration of excessive lower-airway secretions and improve lung function [3]. Although N-acetylcysteine (Mucomyst, Apothecon, Princeton, NJ), the prototypic mucolytic agent, liquefies inspissated mucous plugs when administered by direct intratracheal instillation [26], it is of questionable clinical use when administered as an aerosol to nonintubated patients because very little of the drug is actually delivered to the lower respiratory tract. Inhaled N-acetylcysteine failed to prevent deterioration in lung function or exacerbations in patients with COPD [27], and failed to demonstrate any benefit of nebulized N-acetylcysteine in patients with CF [28]. However, a small randomized trial suggested that nebulized N-acetylcysteine in combination with aerosolized heparin reduced the incidence of acute lung injury (ALI) and decreased mortality in patients with acute smoke inhalational injuries [29]. Because mucolytic instillations or aerosols can induce bronchospasm in patients with airway disease [30] (especially asthma), mucolytics should be administered to these patients in combination with a bronchodilator [3]. However, given the lack of evidence from randomized trials supporting its benefits, we do not recommend the routine use of aerosolized N-acetylcysteine.
Recombinant Human DNase
Recombinant human DNase (Pulmozyme, Genentech, South San Francisco, CA), when given as an aerosol in a dose of 2.5 mg once or twice a day to patients with CF, led to a moderate but significant decrease in dyspnea, a reduction in costs related to exacerbations of respiratory symptoms, and a modest improvement in FEV1 after 3 months [2]. However, there may not be any statistically significant therapeutic benefit of rhDNase when added to antibiotics and chest physical therapy [31].
Two double-blind, placebo-controlled clinical trials evaluated the safety and efficacy of nebulized rhDNase in the treatment of non-CF–related bronchiectasis [32,33]. In these studies, rhDNase was consistently found ineffective (and possibly harmful [32]) to patients with non-CF–related bronchiectasis.
In a randomized double-blind, placebo-controlled trial of patients with respiratory syncytial virus (RSV) bronchiolitis, significant improvement in chest radiographs occurred with the use of nebulized rhDNase compared to significant worsening in a placebo group. Although further investigation is needed, results of this trial indicate a possible future role for this therapy in the treatment of RSV in infants and young children [34].
Other Mucolytics
Studies to determine the efficacy of other mucolytic agents, including water, have produced conflicting results. Current evidence does not appear to justify their use in clinical practice. Consensus guidelines for asthma [15] and COPD [5] do not recommend the use of mucolytic agents in the treatment of acute exacerbations.
Anti-infectives
Aerosolization of antimicrobial solutions has been shown to be effective in CF patients with tracheobronchial infections and colonization [2]. In addition, inhaled antibiotics have also been used to treat tracheobronchial infections in patients with non-CF–related bronchiectasis, to treat and prevent ventilator-associated pneumonia, to treat chronic bronchitis in patients with COPD, to treat bronchiolitis in children, and to treat patients with multidrug-resistant tuberculosis (MDR-Tb) and mycobacterium avium complex (MAC) [13]. However, unlike their use in treating patients with CF, the benefits of using
inhaled antibiotics for these other indications is less defined. Inhaled tobramycin has been demonstrated to decrease sputum bacteria counts, improve lung function, decrease the number of exacerbations, and improve quality of life in patients with pulmonary infections or colonization from CF [35]. For patients with non-CF–related bronchiectasis, inhaled antibiotics are not as well studied, but may decrease sputum bacteria counts and decrease the number of hospitalizations, but have no impact on lung function or survival [36]. Inhaled antibiotics have not been shown to provide any benefit in patients with chronic bronchitis or COPD [37]. Prophylactic use of inhaled antibiotics to decrease the risk of developing ventilator-associated pneumonia has not been shown to be of any benefit [37]. In addition, inhaled antibiotics appear to have no benefit over systemic antibiotics in treating ventilator-associated pneumonia [37]. A few small studies suggest that inhaled amikacin and rifampicin may be of some benefit in treating severe MDR-Tb and severe infections with MAC [38].
inhaled antibiotics for these other indications is less defined. Inhaled tobramycin has been demonstrated to decrease sputum bacteria counts, improve lung function, decrease the number of exacerbations, and improve quality of life in patients with pulmonary infections or colonization from CF [35]. For patients with non-CF–related bronchiectasis, inhaled antibiotics are not as well studied, but may decrease sputum bacteria counts and decrease the number of hospitalizations, but have no impact on lung function or survival [36]. Inhaled antibiotics have not been shown to provide any benefit in patients with chronic bronchitis or COPD [37]. Prophylactic use of inhaled antibiotics to decrease the risk of developing ventilator-associated pneumonia has not been shown to be of any benefit [37]. In addition, inhaled antibiotics appear to have no benefit over systemic antibiotics in treating ventilator-associated pneumonia [37]. A few small studies suggest that inhaled amikacin and rifampicin may be of some benefit in treating severe MDR-Tb and severe infections with MAC [38].
Only tobramycin is currently FDA approved for inhalational use. Other antibiotics occasionally administered via an aerosol include colistin, amikacin, gentamicin, aztreonam, azithromycin, vancomycin, ceftazidime, and imipenem. Inhaled colistin should be used with great caution. Colistin decomposes into several toxic compounds that, if inhaled, can result in acute lung injury and respiratory failure. Colistin suspension should be administered within 6 hours after it is prepared [39].
Inhaled tobramycin is approved for treatment of patients with CF who are (a) at least 6 years of age, (b) have FEV1 greater than or equal to 25% and less than or equal to 75% predicted, (c) are colonized with Pseudomonas aeruginosa, and (d) are able to comply with the prescribed medical regimen [35]. When nebulizing tobramycin, it has been shown that different nebulizers and solutions and techniques may result in very different amounts of tobramycin being inhaled [40]. For example, the addition of albuterol lowered the surface tension of the solution in the nebulizer and resulted in a greater output of tobramycin. A prospective study [41] determined that antibiotics aerosolized by nebulizer could be effectively delivered to tracheostomized, mechanically ventilated patients. In this study, antibiotic concentrations similar to or greater than those achieved in spontaneously breathing individuals were “consistently demonstrated” in patients with a tracheostomy tube.
Aerosolized ribavirin has been used for patients with RSV infection and severe lower respiratory tract disease, or infants with chronic underlying conditions such as cardiac disease, pulmonary disease, or a history of prematurity [3]. However, proof of effectiveness in treating RSV infections is lacking. One study failed to establish the efficacy of inhaled ribavirin in immunocompromised adults with RSV infections [42]. Two prospective double-blind, randomized, placebo-controlled trials addressing the use of aerosolized ribavirin in treating children and adults with respiratory failure from RSV infections failed to show any improvement in length of time requiring mechanical ventilation, length of stay in the intensive care unit, and oxygen requirements or alter immediate outcome [3]. Aerosolized ribavirin has been suggested to be beneficial in treating infections due to influenza A and B [43]. However, a randomized double-blind, placebo-controlled trial found that aerosolized ribavirin only resulted in accelerating normalization of temperature in children with influenza, but had no effect on respiratory rate, pulse rate, cough, or level of consciousness [44].
Ribavirin, in combination with systemic corticosteroids, was used empirically for the treatment of severe acute respiratory syndrome (SARS). However, a review of 14 clinical reports failed to demonstrate that ribavirin decreased the need for mechanical ventilation, or mortality, in patients with SARS [45].
There are several potential hazardous effects of aerosolized ribavirin. It can cause nausea, headaches, and bronchospasm [46]. In addition, it poses potential risks to healthcare workers who administer the medication. It has been shown to cause conjunctivitis as it can precipitate on contact lenses, and bronchospasm in healthcare workers administering the medication [46]. In addition, ribavirin is highly teratogenic. Although studies suggest that absorption of ribavirin by healthcare workers administering the medication is minimal [3], the short-term and long-term risks to women remain unknown. Therefore, conservative safety practices must be followed [3,46]. Given the lack of evidence supporting its efficacy, its known and potential side effects, and the availability of more efficacious treatment options, we do not recommend the use of aerosolized ribavirin in treating infections with RSV. Further studies are needed to determine its efficacy in treating influenza.
Although studies in patients with acquired immunodeficiency syndrome suggest that aerosolized pentamidine can be effective and well tolerated in mild Pneumocystis jiroveci pneumonia, it is not recommended for routine clinical practice [47]. Although aerosolized pentamidine has been used with success for primary and secondary P. jiroveci pneumonia prophylaxis [47], trimethoprim-sulfamethoxazole has been recommended as the drug of choice for prophylaxis in both situations. Aerosolized pentamidine (300 mg reconstituted with sterile water, administered every 4 weeks), delivered by a Respirgard II nebulizer (Marquest, Englewood, CO), has been approved for P. jiroveci pneumonia prophylaxis [47]. A retrospective study suggested that a standard ultrasonic nebulizer (Fisoneb, Fisons, NY) would yield similar effects to Respirgard II, a jet nebulizer, in providing primary and secondary prophylaxis with aerosolized pentamidine [48]. Because toxicity studies on the secondhand effects of aerosolized pentamidine exposure on healthcare personnel are limited [49], conservative safety practices are necessary.
Corticosteroids
At present, there is no indication for the use of inhaled corticosteroids in the treatment of the critically ill with acute exacerbations of obstructive lung disease. Systemic corticosteroids (oral or intravenous) are the recommended first-line agents for the treatment of acute asthma [15] and COPD [5]. Because inhaled corticosteroids are an integral component of asthma therapy, on discharge, they should be used in all patients receiving tapering doses of oral prednisone. They are considered the most effective anti-inflammatory therapy for control of persistent asthma [15]. Inhaled corticosteroids are available as MDIs, dry-powder inhalers, or inhalation suspension (budesonide) for aerosolized use.
When patients are hospitalized for reasons other than acute airway obstruction, inhaled corticosteroids may be continued if patients have been taking these agents for asthma or COPD maintenance therapy. To reduce the risk of oral candidiasis, mouth rinsing and use of a spacer device with MDI are recommended.
Racemic Epinephrine
Racemic epinephrine is effective in decreasing laryngeal edema by causing vasoconstriction [3]. The usual adult dose is 0.5 mL of a 2.25% solution diluted in 3 mL of normal saline every 4 to 6 hours. Because rebound edema frequently occurs, patients must be observed closely. Tachycardia is common during treatment and may precipitate angina in patients with coronary artery disease [3]. The role of racemic epinephrine aerosol in epiglottitis is not known. Similarly, inhaled racemic epinephrine is used to treat postextubation stridor, but this use has not been rigorously studied. Nebulized racemic epinephrine appears to have no benefit over nebulized albuterol in the management of bronchiolitis [50]. Because racemic epinephrine aerosol is associated with potentially serious side effects in
patients with coronary artery disease, administration of inhaled mixtures of helium and oxygen should be considered first to decrease airway resistance and, therefore, the work of breathing associated with laryngeal edema or other upper-airway diseases (see the section Helium-Oxygen [Heliox]).
patients with coronary artery disease, administration of inhaled mixtures of helium and oxygen should be considered first to decrease airway resistance and, therefore, the work of breathing associated with laryngeal edema or other upper-airway diseases (see the section Helium-Oxygen [Heliox]).
Aerosolized Vasodilators
Iloprost is an approved inhaled prostacyclin analog used for the chronic treatment of primary pulmonary hypertension and pulmonary hypertension due to use of appetite suppressants, portopulmonary syndrome, connective tissue disease, and chronic thromboembolic disease. It has also been used in patients with acute pulmonary hypertension after coronary bypass surgery, and may be more effective than inhaled nitric oxide [51]. It is currently FDA approved for patients with primary pulmonary hypertension and New York Heart Association (NYHA) class III (symptoms with minimal activity) and class IV (symptoms at rest) symptoms. Iloprost is administered as 2.5 to 5 μg doses, six to nine times per day. It needs to be delivered via a specialized nebulizer system, the Prodose AAD system (Respironics, Murrysville, PA), to ensure proper dosing. A randomized double-blind, placebo-controlled trial demonstrated that iloprost produced improvements in 6-minute walk, hemodynamics, dyspnea, and quality of life after 12 weeks of therapy [52].
Inhaled Cyclosporin
A randomized double-blind, placebo-controlled trial demonstrated improvement in survival and longer periods free of chronic rejection in lung transplant patients treated with inhaled cyclosporin [53]. The patients in the treatment group received 300 mg of aerosolized cyclosporin (Novartis, East Hanover, NJ) three times a week for the first 2 years after lung transplantation, in addition to usual systemic immunosuppression. There was no increase risk of side effects or opportunistic infections in the treated group.
Modes of Delivery
In the critical care setting, there are generally two types of aerosol delivery devices in use: those that create and deliver wet particles (air-jet nebulizers) and those that deliver preformed particles (pressurized MDIs) with or without MDI auxiliary delivery systems (spacers). Patients on mechanical ventilation or patients breathing through a tracheostomy cannot use dry-powder inhalers. Successful aerosol therapy is dependent on the percentage of the drug that is delivered to the lungs. Factors that influence aerosol deposition and effectiveness, such as flow rate, breathing pattern, and incoordination, have been largely overcome with newer and more advanced designs.
Nebulizers
Air-jet nebulizers are a nonpropellant-based option for inhaled drug delivery. Jet nebulizers rely on a high gas flow (provided by a portable compressor, compressed gas cylinder, or 50-psi wall outlet), Venturi orifices, and baffles to generate respirable particles, generally in the range of 1 to 5 μm diameter [3]. Small-volume nebulizers, equipped with small fluid reservoirs, are used for drug delivery [3]. Factors that affect their performance include design, characteristics of the medication, and gas source. Large-volume nebulizers have reservoir volumes greater than 100 mL and can be used to deliver aerosolized solutions over an extended period. Large versions are used to deliver bland aerosols into mist tents.
Nebulizers are frequently used in pediatric and elderly populations as well as in the hospital setting. Nebulizer delivery of aerosolized drugs is indicated when a drug is not available in MDI form and when a patient cannot coordinate the use of an MDI. Disadvantages include the need for a gas flow source, lack of portability, cost, and the risk of bacterial contamination if not properly cleaned [54].
Metered-Dose Inhalers
An MDI is a pressurized canister that contains drug suspended in a propellant and combined with a dispersing agent. The canister is inverted, placed in a plastic actuator, and, when pressed, delivers a metered dose of drug. The MDI is capable of delivering a more concentrated drug aerosol, as a bolus, than the solutions commonly available for nebulizers [3]. Delivery of a therapeutic dose is dependent on the quality of the patient’s technique, which requires a slow, deep inhalation followed by a breath hold (approximately 10 seconds). Because this maneuver can be difficult, especially if the patient is experiencing respiratory distress, it is essential that the technique be taught and supervised by trained personnel.
Older MDIs use chlorofluorocarbon propellants (CFCs). Their use has now been phased out after the United Nations passed the 1987 Montreal Protocol that called for the banning of substances that may adversely affect the ozone layer. Although medical devices were initially exempted, many pharmaceutical companies began to formulate alternative preparations and delivery systems. Hydrofluoroalkane-134a (HFA) has been found to be an effective alternative to chlorofluorocarbon propellants. In addition, dry-powder inhalers for long- and short-acting β agonists, corticosteroids, and tiotropium have been developed. Another advantage of the HFA-containing MDIs and the dry-powder inhalers is that lung deposition of the medication appears to be greater when compared to the CFC-containing MDIs.
Metered-Dose Inhaler Auxiliary Devices
To overcome problems such as incorrect administration, oropharyngeal deposition, and inconsistent dosing associated with MDI aerosol delivery, several auxiliary devices (i.e., spacer, holding chamber) were developed [3]. When used properly, these devices have the following advantages: (a) a smaller, more therapeutic particle size is achieved; (b) oropharyngeal impaction is decreased; (c) fewer systemic side effects are experienced due to less oropharyngeal deposition compared to MDI alone; and (d) the risk of oral thrush associated with inhaled corticosteroids is decreased. It has been shown that among patients who have difficulty with coordination—particularly the elderly, handicapped, infants, and children younger than 5 years of age—spacer devices improve the efficacy of MDIs [55].
Choice of Delivery System
Since the development of the first MDI in the 1960s, there has been continuing debate about which aerosol delivery system, nebulizers, or MDI is superior. In 1997, Turner et al. [56] published a meta-analysis of 12 studies that compared bronchodilator delivery via nebulizer to delivery via MDI. Studies included in the review were all randomized clinical trials of adults with acute asthma or COPD who were treated in the emergency department or hospital and measured FEV1 or peak expiratory flow rate. In all but two of the trials, spacers were used with MDIs. Based on the results of these studies, the authors concluded that there was no difference in effectiveness between the two delivery methods.
A Cochrane Library meta-analysis by Cates et al. [17] compared the clinical outcomes of adults and children with acute asthma who received β2 agonists by nebulizer or MDI with spacer. In this review that included 16 randomized controlled trials, the authors concluded that the outcomes (hospital admission, length of stay in the emergency department, respiratory rate, heart rate, arterial blood gases, tremor and lung function) of both groups were equivalent.
In the United States, MDIs are underused in the acute care setting [3]. Barriers to selection of these devices include reimbursement issues and the misconception of clinicians regarding efficacy. Many third-party payors reimburse for the nebulizer/drug package but not for the MDI. In the critical care setting, selection of an aerosol delivery system for the spontaneously breathing patients should be based on several factors. In general, because the MDI with or without spacer is the most convenient and cost-effective method of delivery, it should be chosen whenever possible. Its use may be limited by factors such as the patient’s ability to actuate and coordinate the device, either of which can affect aerosol deposition to the lungs; patient preference; practice situations; and economic evaluations. Additionally, parenchymal dosing with drugs such as pentamidine and ribavirin requires the use of a nebulizer [3]. Cost considerations may determine which delivery system is chosen in different settings. Studies show that use of MDIs with spacers likely produce considerable reductions in hospital costs [57]. The cost of a disposable nebulizer system in a hospital setting may be lower than the cost of a MDI and spacer device if patients are discharged with a second spacer device [17].
Aerosols can be delivered to intubated and mechanically ventilated patients with small-volume side-stream nebulizers connected to the inspiratory tubing or MDIs with an aerosol holding chamber. Although both delivery systems are effective in delivering aerosolized medications to the ventilated patient [3], drug delivery can be significantly reduced if proper technique in setting up and using both devices is not followed.
Lung-Expansion Techniques
A lung-expansion technique is any technique that increases lung volume or assists the patient in increasing lung volume above that reached at his or her usual unassisted or uncoached inspiration. Rationales for the use of various strategies to promote lung inflation include (a) increasing pulmonary compliance, (b) increasing partial arterial pressure of oxygen (PaO2), (c) decreasing work of breathing, and (d) increasing removal of secretions [58]. Lung-expansion techniques are meant to duplicate a normal sigh maneuver. Theoretically, sighs or periodic hyperinflations to near-total lung capacity reverse microatelectasis [3].
Lung-expansion techniques are indicated to prevent atelectasis and pneumonia in patients who cannot or will not take periodic hyperinflations [3], such as postoperative upper-abdominal and thoracic surgical patients and patients with respiratory disorders due to neuromuscular and chest wall diseases. Adequately performed, maximum inspirations 10 times each hour while awake significantly decrease the incidence of pulmonary complications after laparotomy [59]. Whatever technique is used postoperatively (e.g., coached sustained maximal inspiration with cough, incentive spirometry, volume-oriented intermittent positive-pressure breathing, intermittent CPAP, or positive expiratory pressure [PEP] mask therapy [60]), it should be taught and practiced preoperatively. When properly used, coached sustained maximal inspiration with cough and incentive spirometry—the least expensive and safest techniques—are as effective as any other method [61]. Of the several commercially available incentive spirometers, the one chosen should combine accuracy, low price, and maximum patient accessibility [62]. Because there are no definitive studies comparing the relative efficacy of volume- and flow-oriented incentive spirometers, the choice of equipment must be based on empiric assessment of patient acceptance, ease of use, and cost. When chest percussion with postural drainage is added to the previously mentioned expansion techniques in patients without prior lung disease, it has failed to affect the incidence of postoperative pulmonary complications [63].
Airway Clearance
Efficient mucociliary clearance and effective cough are the two basic processes necessary for normal clearance of the airways. In abnormal situations, this system may be dysfunctional and lead to mucus retention. Recently both the ACCP [64] and the BTS [2] have published evidence-based guidelines reviewing both pharmacological and nonpharmacological methods of augmenting pulmonary clearance. Both guidelines are complete reviews on this topic. A summarized discussion of techniques aimed at enhancing airway clearance follows.
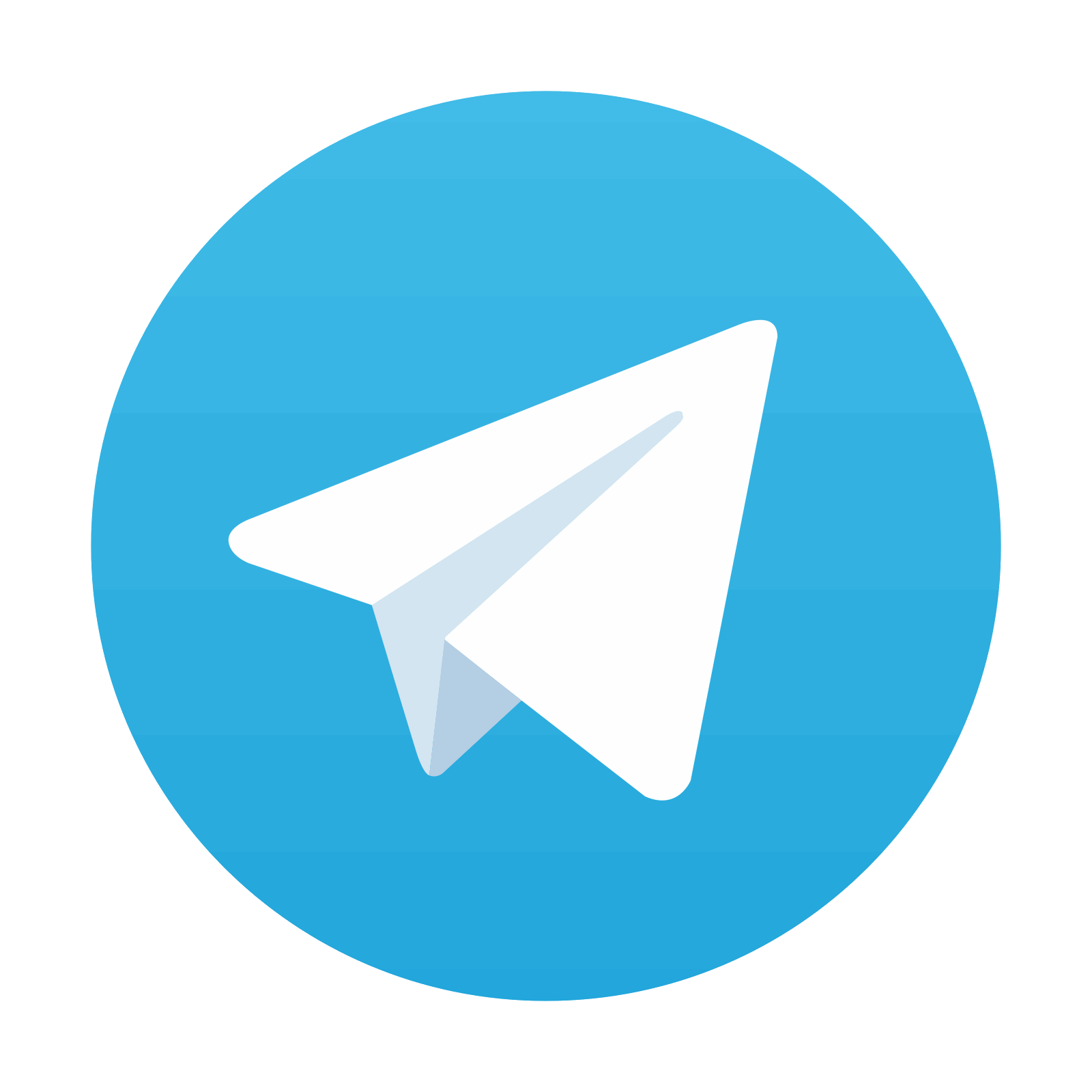
Stay updated, free articles. Join our Telegram channel

Full access? Get Clinical Tree
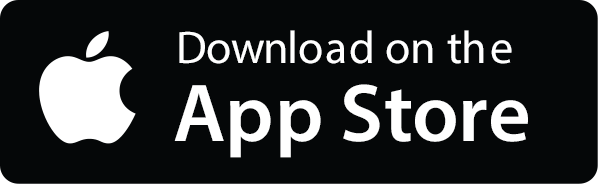
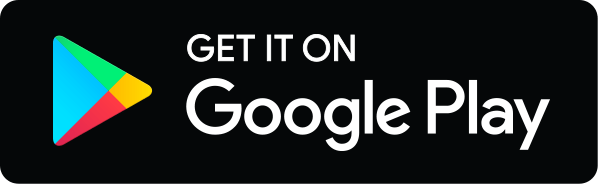