Merriam Webster’s Collegiate Dictionary , 10th edition, lists 1936 as the year of the earliest known use of the word decontaminate in English. Decontaminate is defined as follows: “to rid of contamination (as radioactive material).” To better appreciate the meaning of the word, one should first understand the concept of radiation contamination. Contamination occurs when material containing radioactive particles is deposited on skin, clothing, or any surface area of an inanimate object. A person contaminated with radioactive material will continue to be irradiated until the radioactive material (source of radiation) is eliminated or removed. Interestingly, radiation does not spread in a person; instead, it is the radioactive contamination that can spread. External contamination of a person may occur if radioactive material is deposited on external body surfaces or clothing. Internal contamination occurs if radioactive material is inhaled, ingested, injected into, or absorbed through wounds. The environment can also become contaminated if radioactive materials are uncontained or spread about.
Comprehensive concepts of radiation decontamination are presented in this chapter. The aim is to provide a framework in which to understand the principles of radiation decontamination and their application in controlling exposures to radioactive contamination. The chapter provides a brief historical perspective of radioactivity, summarizes current practices of radiation decontamination, and highlights obstacles to the execution of an optimal radiation decontamination response plan.
Historical perspective
Radiation injury to human cells was first recognized within months after Roentgen’s discovery of x-rays in 1895. In 1896 Becquerel discovered natural radioactivity—the emission of fast electrons from the nuclei of salts of uranium. This mode of radioactive decay was termed beta decay . This discovery eventually led to another discovery and isolation of radium by Marie Curie in 1898. In 1899 Rutherford described radioactive decay by the emission of alpha particles (helium nuclei). And in 1900 Villard was the first to describe electromagnetic radiation release during radioactive decay known as gamma radiation. The three major forms of radioactivity are alpha (α), beta (β), and gamma (γ), named for alpha particles, beta particles, and photon energy, respectively.
After their discovery, x-rays and radioactive materials were used with little regard for their biological effects. The consequences of careless handling and use of radiation sources soon became apparent. Many of the early workers who pioneered the medical applications of ionizing radiation experienced firsthand the deleterious effects of radiation. Curie’s discovery of radium led her to receive the Nobel Prize for chemistry in 1911 and brought about the introduction of radium use in medicine and industry. Internal contamination from radium caused injury to many workers of the radium watch dial–painting industry in the 1920s.
In the early twentieth century the understanding of atomic physics increased rapidly and culminated in the nuclear era. The development of human-made radioactive isotopes during and after World War II was a factor in the establishment of the nuclear industry that had a workforce of several hundred thousand people. Only 97 cases of clinical radiation injury had occurred in this population by 1969. Knowledge of radiation-monitoring procedures was an integral component of the civil defense programs of the 1960s directed against the threat of nuclear weapons use and thermonuclear war. Most of what is known today about radiation decontamination came about after the atomic bomb explosions of World War II and has evolved from three primary areas: therapeutic radiation exposures, radiation accidents, and military preparedness training directed at nuclear weapons–related injuries. The military experience has provided some of the most comprehensive and useful information on radiation decontamination.
Current practice
Basic techniques of radiation decontamination derived from the military can be applied to nonmilitary settings depending on the situation and resources available. Radiation is given off by radioactive particles, most of which appear as dust or debris as in the case of a detonation of a nuclear weapon or radiological dispersal device (RDD). For decontamination purposes, radiation is generally thought of as a solid. Four categories of decontamination are recognized : (1) personal decontamination is decontamination of one’s self; (2) casualty decontamination denotes decontamination of patient casualties; (3) personnel decontamination generally means decontamination of workers who are not patients; and (4) mechanical decontamination includes procedures to physically remove radioactive particulates. Radiation decontamination is not an emergency. Decontamination of casualties is a labor-intensive task. The process demands the dedication of a significant number of personnel and large amounts of time. Appropriate planning and training are a necessity. The demands may require a major contribution of resources.
Monitoring Instruments
A variety of instruments are available for detecting and measuring radiation. Radiation monitoring entails the measurement of radiation fields in the vicinity of a radiation source, measurement of surface contamination, and measurement of airborne radioactivity. Such monitoring methods are also known as radiation surveys. Radiation survey meters are used to evaluate radiation contamination of patients, equipment, or the environment. Old civil defense instruments, such as the CD V-700 and CD V-715 survey meters, can be used. The CD V-700 meter is used to detect low-intensity gamma and most beta radiation. It can measure only up to 50 mR/h. The CD V-715 meter is used to measure high-intensity gamma radiation. It can measure up to 500 R/h; however, it cannot detect beta or alpha radiation. These instruments are also called Geiger counters or Geiger-Mueller meters.
Newer portable and compact radiation monitor units with digital readouts and alarm systems are commercially available to measure alpha, beta, and/or gamma radiation. Because alpha radiation travels a very short distance in air and is not penetrating, radiation survey instruments cannot detect alpha radiation through even a thin layer of water, blood, dust, paper, or other materials. Most beta emitters can be detected with a survey instrument, such as a CD V-700, provided the metal probe cover is opened. Because gamma radiation or x-rays frequently accompany the emission of alpha and beta radiation from radioactive isotopes, the latter constitute both an external and internal hazard to humans. Gamma radiation is readily detected with survey instruments.
Radiological Assessment
In the period immediately after a detonation of, for example, a nuclear or large RDD, there will be considerable uncertainty about the nature and extent of radioactive contamination. It is imperative that radiation measurements be obtained as soon as possible to implement proper protective actions against potential radiological hazards. On-scene radiological assessments can be easily and rapidly performed using exposure rate measurements. However, assessments of long-term consequences require knowledge of the specific radioactive isotopes and more specialized skills. An RDD that contains nuclear spent fuel as a radiation source will probably release one or a few radioisotopes on detonation. This simple source is easier to measure and characterize than mixed radiation sources potentially released from nuclear reactors or weapons. For nearly a century, epidemiologic studies of various human populations exposed to ionizing radiation have provided significant quantitative information on health risks. Much data are known about radiation and its risks; however, the important unanswered question is what the level of risk is from low dose exposures.
Prehospital Decontamination
Most health care workers, such as emergency first responders, hospital first receivers, and others, lack radiation-related training. These responders have not received sufficient training about ionizing radiation, radiation safety, and managing, diagnosing, or treating radiation-related injuries. The effectiveness of any medical response and recovery effort can be improved by providing a radiation training strategy for the health care delivery team. The detonation of a nuclear or radiological device within a U.S. city would create a national need for health care practitioners to manage radiation casualties. To provide guidance for health care providers about the diagnosis and treatment of radiation injury during nuclear and radiological emergencies the Department of Health and Human Services launched the Radiation Emergency Medical Management (REMM) website in 2007. Since that time REMM content has expanded significantly with a variety of REMM versions having been launched. State radiation safety or health departments should provide field teams to assist in radiation monitoring at the scene of a nuclear or radiological incident. The radiological evaluation of injured patients should be performed by persons with radiation health and safety training under the supervision of on-scene medical personnel. Patient decontamination performed by emergency responders should be brief. The goal should be to remove all gross radioactive dust or debris from body surfaces. If clothing and shoes are contaminated, they should also be removed. These measures may benefit the patient by eliminating sources of radiation exposure and reducing the cumulative absorbed dose the patient would have received. Depending on the severity of injuries and the extent of radiation exposure, these simple decontamination methods may be life-saving while the patient is en route to more definitive care at the nearest hospital emergency department.
Hospital Decontamination
To prevent or minimize the occurrence of radiological contamination of the hospital facility and staff, a decontamination area should be established outside the hospital, preferably downwind from the clean treatment area or hospital entrance. A patient arrival/triage area should be downwind from the decontamination area. Wind direction is vital because resuspension of radioactive dust may occur downwind from the contaminated area. Outdoor patient decontamination is always performed downwind from the patient arrival/triage area. Ideally the decontamination area should be set up to take advantage of the prevailing wind. The setup should be adaptable. Consideration must be given to the security of the decontamination area. An outdoor shower system may also be considered for use of mass decontamination. Portable vacuum units with high-efficiency particulate air filters have reportedly been used to facilitate rapid decontamination outdoors.
An entry control point is necessary to identify and manage movement of clean and contaminated vehicles to the decontamination site. Control of patient and staff movement is critical to ensure that contaminated ambulatory patients and staff do not accidentally contaminate clean areas. A hotline (i.e., division between contaminated and clean zone) should be established and secured. Any people or equipment leaving a contaminated area must undergo radiological monitoring to make sure that radioactive contaminants do not enter clean areas. In addition, a radiation emergency area (a location for indoor decontamination) should be part of the radiation emergency plans in the event that patients contaminate the hospital. Hospitals with nuclear medicine departments have an added resource—a gamma camera. A gamma camera is a perfect device for detecting nuclear fission products from either nuclear detonations or nuclear power plant reactors accidents.
Patient Decontamination
Removal of outer clothing and rapid washing of exposed skin and hair remove 95% of contamination. Standard patient decontamination is normally performed under the supervision of medical personnel. Moist cotton swabs of the nasal mucosa from both sides of the nose should be obtained, labeled, and sealed in separate bags. These swabs can be used as evidence for inhalation of radioactive particles. A 0.5% sodium hypochlorite solution can be used to remove radioactive contamination from intact skin. Radioactive material removed from the patient should be preserved for later analysis to identify the specific radioisotope. Maintain care not to irritate the skin. If skin becomes erythematous, some radioactive isotopes can be absorbed directly through the skin. Surgical irrigating solutions, such as normal saline or lactated Ringer’s solution, should be used liberally in wounds, the abdomen, and the chest. These solutions should be removed using suction instead of wiping or sponging. For the eyes, only abundant amounts of water, normal saline, or eye wash solutions are recommended. If feasible, skin wash water should be contained and held for disposal. Contaminated tourniquets are changed with clean ones. Wounds should be covered after adjacent skin is decontaminated to prevent skin contaminants from entering the wound.
Wound Decontamination
During initial decontamination in the receiving/triage area, bandages should be removed and all wounds flushed. If bleeding persists, apply fresh bandages. Highly energetic gamma emitters can present an immediate hazard to contaminated wounds. Particulate matter contaminating a wound should be removed if possible. Alpha and beta emitters left in a wound can cause extensive local injury and may be absorbed into the systemic circulation, where they will be redistributed as internal contaminants; this can cause additional internal organ injury from irradiation. After adequate decontamination of the wound is achieved, it should be copiously irrigated with saline or some other physiological solution. Aggressive surgery, such as amputation, is not necessary and should never be used to manage radioactive contamination of a limb. Partial-thickness burns should be extensively irrigated and cleaned with mild solutions to prevent irritation of burned skin. In full-thickness burns, the presence of radioactive contaminants requires specialized surgical treatment.
Mechanical Decontamination
Radiological contamination may involve one or more radioactive elements. This section addresses the specific decontamination of six common radioactive elements. The decontamination principles discussed here are also applicable to radiological contamination by other elements with chemical properties similar to those discussed below.
Cesium
The most common radioisotope of cesium is cesium-137. It emits beta and gamma radiation, decaying to stable barium-137. Cesium-137 is widely used in gamma sources. It occurs in these sources as cesium chloride pellets. Cesium chloride is a soluble salt. The contamination from a sealed-source leak absorbs water, becomes damp, and creeps. Contamination from a sealed cesium source is best decontaminated by wet procedures unless the contamination is on a porous surface, in which case, vacuuming should precede wet procedures. Cesium is known to adsorb from a solution onto glass surfaces. Decontaminating a liquid cesium–contaminated surface is best accomplished by wetting the surface, absorbing the solution with a rag or other absorbent material, and rinsing the area several times with water. If the contamination persists, use a detergent solution and scrub with a brush.
Cobalt
The most common radioisotope of cobalt is cobalt-60, which is a beta and gamma emitter. Metallic cobalt-60 is commonly used in sealed gamma sources. Particles of cobalt dust adhering to small articles are readily removed by ultrasonic cleaners or by dipping the article in a dilute solution of nitric, hydrochloric, or sulfuric acid. Cobalt dust contamination that exists over a large area is best removed by vacuuming. Sealed cobalt sources may leak as a result of electrolytic action between the cobalt and the container. The result is often a soluble cobalt salt, which creeps and spreads. This is best decontaminated with a detergent or an ethylenediamine tetraacetic acid solution, followed by treatment with mineral acids. Contamination from solutions containing cobalt may be treated with water.
Plutonium
The most common isotope in which plutonium may be present as a contaminant is plutonium-239, which is an alpha emitter. Plutonium contamination may be the result of a nuclear weapons accident, in which case the plutonium will be scattered as a metal or oxide in a dust form. Both forms of plutonium are insoluble. Aging of plutonium-239 contamination is impractical because it has a 24,000-year half-life. Plutonium contamination that covers a small area is best decontaminated by vacuuming. If contamination remains, the area should be washed with a detergent solution. Any contamination that remains can be sealed in a protective coating of paint, varnish, or plastic. Plutonium oxide or metal dust spread over a large area, such as a field, is best decontaminated by removing the top layer of soil and disposing of it as radioactive waste. Because inhalation is generally considered the most likely route of entry of plutonium during a nuclear accident or nuclear terrorist incident there can be considerable harm to humans from plutonium exposure. Personnel should wear respiratory protection when decontaminating or removing the soil.
Strontium
The most common radioisotope of strontium is strontium-90, which is a beta emitter. The daughter particle of strontium-90 is yttrium-90, which is also a beta emitter. Strontium-90-yttrium-90 is commonly used in sealed beta sources. Generally it is present as a chlorine or carbonate. The chlorine is hygroscopic; it absorbs water and creeps out of the container. This contamination is best decontaminated by vacuuming, followed by treatment with water, a complexing agent solution (i.e., substance capable of forming a complex compound with another material in solution), and a mineral acid, in that order. Contamination resulting from a strontium-containing solution is best decontaminated by absorbing the solution with rags or other absorbing materials and washing the area with a detergent solution. If strontium contamination persists, the top layer of the surface should be removed by abrasives or other removal procedures and a sealing coat should be placed over the surface.
Tritium
Tritium is the radioisotope of hydrogen and is a weak beta emitter. If it is released to an area as a gas, the best decontamination method is to flush the area with air. As inhalation tritium can present an internal hazard, personnel entering an area containing tritium gas should wear an appropriate self-contained type of breathing apparatus. Objects in an area exposed to tritium for any great length of time may absorb the gas and should be disposed of, if possible. They may be degassed, under a vacuum, by flushing with helium or hydrogen. A surface that is monitored as clean may become contaminated again in a matter of hours by percolation. There is no practical way of removing tritium oxide (T 2 O) from water due to its similarity to natural water.
Uranium
The most probable source of uranium contamination is a nuclear weapon accident in which the fissionable uranium is spread as a metal or oxide dust. The common isotopes of uranium contamination are uranium-235 and uranium-238. This metal or oxide is insoluble and is best removed from a contaminated surface by brushing or vacuuming, followed by a treatment with mineral acids or oxidizing acids, and then the area should be sealed. Large-area uranium contamination is best decontaminated by removing the top layer of the surface or by sealing it.
Equipment and Building Decontamination
In most instances of equipment and building contamination, a mixture of normal household cleaning practices will remove the radioactive material. Vacuum cleaners that can handle wet material and have high-efficiency particulate air filters are suggested.
Personal Protective Equipment
Members of on-scene field radiological decontamination teams and hospital-based teams should have appropriate protective equipment to meet all requirements for radiation decontamination. Emergency medical services and hospital personnel responsible for patient decontamination should also be appropriately equipped to protect themselves from the hazards of radioactive contamination.
Respiratory Protective Devices
There are two types of respiratory protective devices: air-purified respirators, which remove contaminants from breathing air by filtering or chemical absorption, and air-supplied respirators, which provide clean air from an outside source or from a tank. Most air-purified respirators (i.e., protective masks) afford excellent protection from inhalation of radioactive material. Radioisotopes such as radon and tritium gas will pass through these filters. However, short exposures to these gases are not considered medically significant. The device providing the greatest factor of safety for a particular radiation incident should be used.
In nuclear weapons accidents or terrorist incidents, most nuclear weapons will contain high explosives in varying amounts—even as much as hundreds of pounds. Detonation of high explosives in nuclear weapons will cause a major radiological threat—the release of plutonium-239. When associated with a fire, metallic plutonium may burn, producing radioactive plutonium oxide particles and serious inhalation and wound deposition hazards. In these situations use of self-contained breathing apparatuses should be considered.
In RDD incidents in which radioactive contamination is associated with fire and dangerous chemical fumes from burning metals and plastics, use of self-contained breathing apparatuses should be considered. Such devices enable radiation response and decontamination workers to enter a contaminated or oxygen-deficient environment, up to the limits of the respirator. They should be used when it is necessary to enter a highly contaminated environment to rescue persons, for example, from RDD incidents or nuclear power plant accidents.
Protective Clothing
Anticontamination suits are commercially available for use in nuclear and radiological disasters. Chemical-protective clothing provides excellent protection against radioactive contamination while also offering protection from chemical and biological agents or hazards. A wide variety of chemical-protective clothing is available to protect the body, including gloves, boots, coveralls, and total-encapsulation protective suits. Standard hospital barrier clothing that is used in universal precautions is adequate for protection of hospital personnel who provide emergency evaluation and treatment to limited numbers of radiologically contaminated patients. In these instances, hospital personnel should be decontaminated after the patients’ emergency treatment and decontamination.
Radiation Dosimeters
There are a variety of detectors used to measure a person’s level of radiation exposure. Film badges, thermoluminescent dosimeters (TLDs), pocket dosimeters, or other devices should be used by radiation decontamination personnel, emergency responders, and hospital medical providers who are involved in any nuclear accident or terrorist incident.
Film badges are the most common dosimeter in use. They are worn on the outer clothing and are used to measure gamma, x-ray, and high-energy beta radiation. A badge consists of a small piece of photographic film wrapped in an opaque cover and held in a metal frame. It can be worn as a ring or pinned to clothing. Radiation interacts with the atoms in the film to expose the film. At periodic intervals, the film is removed and is developed to determine the amount of radiation exposure. A film badge provides a permanent record of radiation exposure.
TLDs are used for measuring gamma, x-ray, and beta radiation exposures. They can be worn as rings or body badges. They contain small chips of lithium fluoride, which absorb ionizing radiation energy and displace electrons from their ground state. The electrons then become trapped in a metastable state but can be restored to their original ground state by heating. When heated, the electrons return to their ground state and light is emitted. A TLD readout instrument is used to heat the chips and measure the emitted light. The amount of light emitted is related to the dose of radiation absorbed by the TLD and to the radiation exposure dose of the individual. TLDs are beginning to replace film badges.
A pocket dosimeter is a direct-reading portable unit shaped like a fountain pen with a pocket clip. It is worn on the trunk of the body and is generally used to measure x-ray and gamma radiation. It should be used in conjunction with a TLD rather than in place of TLD use. The pocket dosimeter consists of a quartz fiber, a scale, a lens to observe movement of the fiber across the scale, and an ionization chamber. The quartz fiber is charged electrostatically until it reaches zero on the scale. When the dosimeter is exposed to radiation, some of the atoms of air in the chamber become ionized. This causes the static electricity charge to leak from the quartz fiber in direct response to the amount of radiation present. As the charge is lost, the fiber moves to some new position on the scale that indicates the amount of radiation exposure.
The main advantage of the pocket dosimeter is that it can be read immediately by the wearer, even while working in a radiation-contaminated environment, instead of waiting for processing of a film badge or TLD. However, because pocket dosimeters lose their electrical charge over time, they may give a false indication of radiation exposure. When practicable, use of two dosimeters can prevent false interpretation of a person’s exposure. One should assume that the lower reading is the actual exposure.
Basic Radiation Safety
During a nuclear or radiological disaster it is vital that emergency medical responders, decontamination team personnel, and hospital health care providers adhere to the basic principles of radiation safety. These actions will help to prevent or minimize the risk of these persons becoming radiation casualties due to exposure to radioactive contaminants. The three basic principles of radiation protection are time, distance, and shielding.
Time
The longer the exposure to radioactive contaminants, the possibility of radiation injury is greater. There is a direct relationship between the exposure dose received and the duration of exposure. Reducing exposure time in a contaminated area will reduce the radiation exposure. The maximum acceptable exposure time can be calculated based on the exposure dose rate measured using radiation survey meters at a given incident scene and the maximum dose that is needed to accomplish radiation decontamination or other task. In practice the dose received in completing a task may be spread over several workers so that no one person’s exposure exceeds guide levels.
Distance
The inverse square law states that the dose from a radiation point source decreases with the square of the distance from the source. For example, doubling the distance from the source of radiation, the exposure would be decreased to ( <SPAN role=presentation tabIndex=0 id=MathJax-Element-1-Frame class=MathJax style="POSITION: relative" data-mathml='½’>½½
½
) , or one fourth of the original amount. In nuclear or radiological incidents, the radiation source may not be equivalent to a point source and so the inverse square law can be used as an approximation. In most instances, the approximation should be adequate. Maintaining a safe distance is especially critical. The larger the distance from a radiation source, the lower the dose.
There are certain emergency response operations that cannot be performed without some exposure of workers. In these situations, all unnecessary exposure to radiation should be avoided, even if it means barring workers from entering contaminated areas. These hazardous areas should be barricaded or roped off to form a restricted area that cannot be entered by non–radiation workers or bystanders.
Shielding
Shielding is generally used to safeguard against radiation from radioactive sources. The more mass placed between a source and a person, the less radiation the person will receive. This should be the guiding principle. Proper shielding from a source requires knowledge of the type of radiation hazard. Different types of shielding are required for alpha, beta, and gamma radiation. Light clothing will provide protection and prevent contamination of the body from alpha radiation. Light metals such as aluminum are preferred for shielding from beta emissions. A sheet of aluminum can stop most beta radiation. Plexiglas is another shielding material that is effective against beta particles. Because gamma radiation is more penetrating than alpha and beta particles, higher-density materials such as lead, tungsten, steel, and concrete are ideal for shielding gamma rays. As the thickness of these materials increases, the intensity of the gamma radiation will decrease.
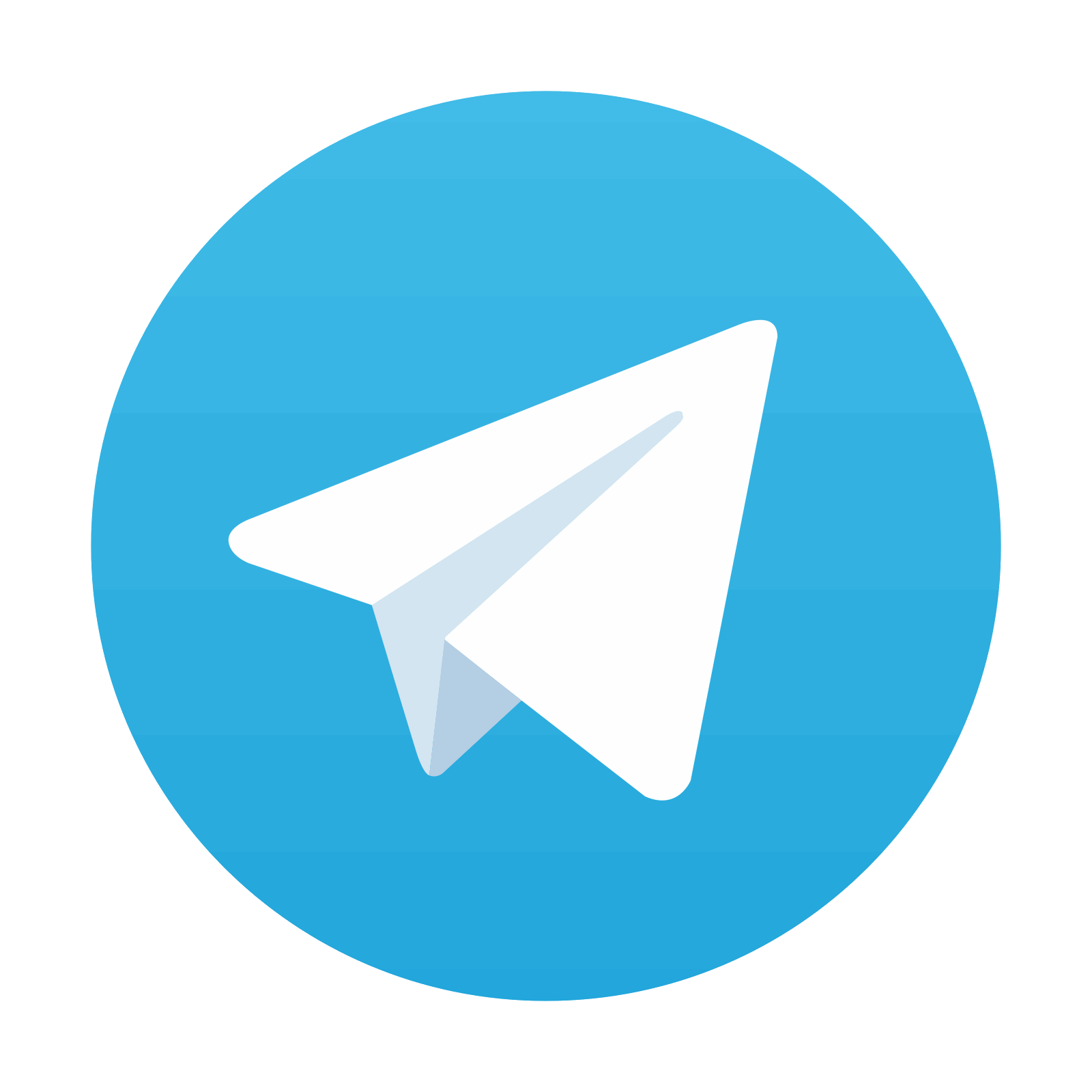
Stay updated, free articles. Join our Telegram channel

Full access? Get Clinical Tree
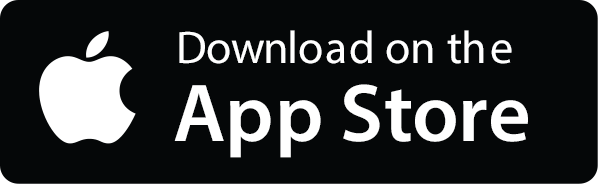
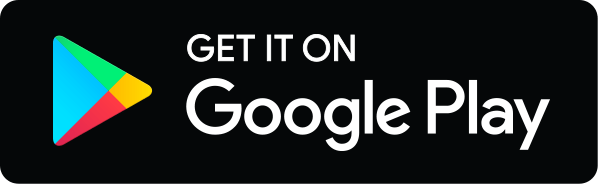