Pulse Oximetry: Even a Simple Device Requires User Understanding
Jorge Pineda JR MD
Stephen T. Robinson MD
Pulse oximetry has become an invaluable tool in the current practice of perioperative medicine. It offers dynamic assessment of oxygenation status, which allows for rapid recognition and evaluation of interventions in cases during times of hypoxia on a nearly moment-to-moment basis. Advantages of this monitor include its noninvasiveness, wide availability, and simplicity. However, studies have shown a lack of knowledge concerning the limitations and interpretation of pulse oximetry saturation among both physicians and medical staff.
Pulse oximetry is based on three principles. First, every substance has a unique absorbance spectrum. Different types of hemoglobin have different absorption spectra. The two light-emitting diodes (LEDs) of the pulse oximeter each emit a different, specific wavelength of light within the red to near-infrared range, and a photo detector measures the amount of light transmitted through the tissue. The second principle is based on the Beer-Lambert Law which states that the absorption of light as it passes through a clear nonabsorbing solvent is proportional to the concentration of the solute and the length of the path along which the light travels in that solvent. Finally, the third principle states that the presence of a pulsatile signal generated by arterial blood is relatively independent of the nonpulsatile venous blood in the tissues. By measuring transmitted light several hundred times per second, the pulse oximeter can distinguish the variable, pulsatile component of arterial blood from the unchanging component of the signal made up of the tissue, venous blood, and nonpulsatile arterial blood. The pulsatile or alternating component, which generally comprises 1% to 5% of the total signal, can then be isolated by canceling out the static component.
ACCURACY
Pulse oximetry, or the determination of SpO2 level, is derived from calibration curves of oxygen saturation that were experimentally measured in healthy volunteers. Values obtained with coincident determination of oxygen saturation by the pulse oximeter were compared with values obtained in in vitro laboratory co-oximetry during normal oxygenation and induced hypoxia. Researchers were limited in the degree of hypoxemia inducible
in these volunteers to an arterial saturation (SaO2) of approximately 75% to 80%. Thus, the shape of the curve below these levels must be extrapolated. For peripheral arterial saturation, most manufacturers report accuracy to within ±2% for SpO2 of 70% to 100% and ±3% for SpO2 of 50% to 70%. There is no reported accuracy below 50% saturation. These claims are largely supported by several review studies addressing accuracy by correlating SaO2 with SpO2. As an SaO2 level of 75% represents a PaO2 level of 40 mmHg, reduced accuracy in this low range does not have any meaningful clinical implications.
in these volunteers to an arterial saturation (SaO2) of approximately 75% to 80%. Thus, the shape of the curve below these levels must be extrapolated. For peripheral arterial saturation, most manufacturers report accuracy to within ±2% for SpO2 of 70% to 100% and ±3% for SpO2 of 50% to 70%. There is no reported accuracy below 50% saturation. These claims are largely supported by several review studies addressing accuracy by correlating SaO2 with SpO2. As an SaO2 level of 75% represents a PaO2 level of 40 mmHg, reduced accuracy in this low range does not have any meaningful clinical implications.
There is a delay between when alveolar oxygen delivery changes and when the SpO2 level shows a change. In general, finger probes are slower (24 to 35 seconds) when compared with earlobe probes (10 to 20 seconds). Changing ventilator management to improve oxygenation requires allowing time for the alveolar oxygen concentration to rise, for the blood with higher oxygen content to reach the site of the pulse oximeter probe, for signal processing to occur, and then for display of the processed signal on the monitor as an SpO2 value. Sufficient time should be allowed when interventions are made based on pulse oximetry readings.
SIGNAL ARTIFACTS
The major limitations of pulse oximetry can be divided into two major categories: those arising from optical signal artifact and those resulting from optical interference. Most problems with pulse oximetry arise from signal artifact. Signal artifact can result from false sources of signal or from a low signal-to-noise ratio. The presence of a sharp pulsatile waveform on the oximeter does not guarantee no signal artifact.
Sources of false signal include detection of nontransmitted light (ambient light or optical shunt) and nonarterial sources of alternating or pulsatile signal. Because light can be a potentially major source of interference, designers divided the LED and photo detector activities into three sensing periods, which can cycle at hundreds of times per second. Of these three periods, two use light emitted by the LEDs at each of the two incident wavelengths. During the third period, neither LED is activated and the photo detector measures only ambient light. This measurement is then eliminated from the LED-illuminated sensing periods. Despite this, ambient light still interferes, and implicated sources include fluorescent lights, surgical lamps, fiberoptic instruments, and sunlight. A simple solution is covering the probe with an opaque shield.
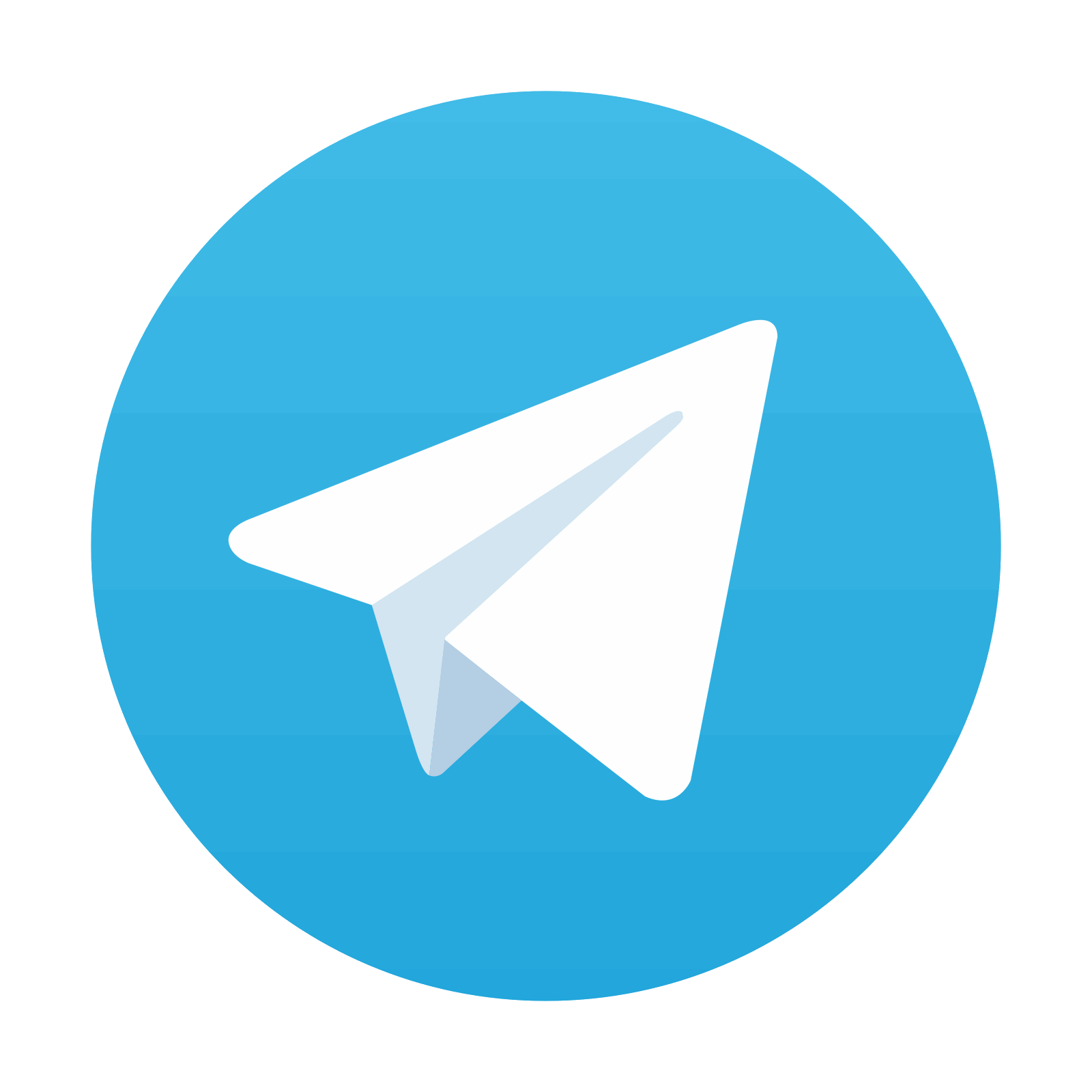
Stay updated, free articles. Join our Telegram channel

Full access? Get Clinical Tree
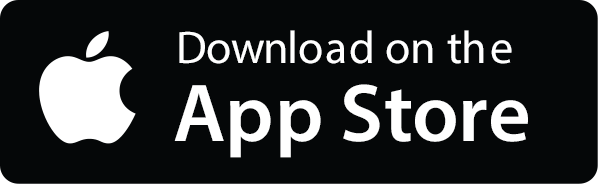
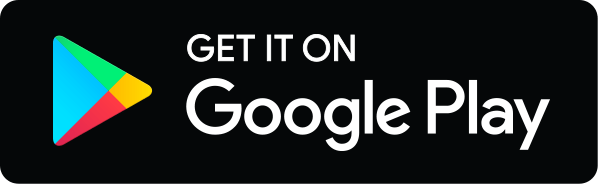