KEYWORDS
pulse oximetry
alarms and safety features
oxygen
Imagine performing an anesthetic without a pulse oximeter. Unless you have been an anesthesiologist or anesthetist for a very long time, you have never done a case without a pulse oximeter. In fact, if you had to have just one single monitor, you would probably choose a “pulse ox.” It tells you at least three things: it tells you the patient’s oxygen saturation, naturally, but it also tells you the patient’s pulse, the fact that the patient’s heart is beating, and that the patient has enough of a systolic blood pressure to allow the probe to pick up a signal.
But anesthetics were done for around 130 years before pulse oximetry became commonplace. It is a testament to how important and how revolutionary the pulse oximetry is that a generation or two after its introduction, none of us would ever do a case without one.
“Revolutionary” is a good word to use because the pulse oximeter and its colleague, the capnograph, came into common clinical practice at around the same time. Anesthetic practice received not one but two blessings in the 1980s. Both technologies quickly became standard of care. Nothing since then has come close to rivaling the benefits that these monitors give us for each and every case.
Anesthesia monitors can be divided into two groups: patient or physiologic monitors, such as a noninvasive blood pressure monitor, and safety monitors, such as an oxygen sensor. A pulse oximeter is a combination of both a physiologic monitor and a safety monitor. It tells us something about the physiologic status of the patient, with regard to ventilation, oxygenation, pulse, and so forth, plus it lets us know that the patient is in fact being oxygenated or not. Remember, the delivery of oxygen is the main function of an anesthesia machine, in our minds, as we discussed earlier in this book.
PHYSICS
Physics, although perhaps having a reputation among those of us who majored in biology, zoology, and so forth in college as being hard and boring, is really just the study of how things work. Therefore, to understand how something works, you have to talk about physics at least a little bit. Again, you can go into the physics of pulse oximetry as deeply as you desire, but in this discussion, we will only discuss the physics of pulse oximetry that we think is important for you to know.
When you were a kid, you probably played with a flashlight by holding your hand over the beam and seeing how the light made your hand look. Some of the light was absorbed by your hand because the beam was not as bright leaving your hand as it was entering your hand. A pulse oximeter shines light through your hand (or wherever you have attached the probe) and measures how much light comes out the other side.
Pulse oximetry works because of the differences in the type and amount of light that oxygenated and deoxygenated hemoglobin absorb, respectively. The pulse oximetry probe shines two LED lights through the vascular bed. One light is red, at a wavelength of 660 nanometers (nm), and the other is an infrared beam of 940 nm of wavelength. The red light is absorbed by reduced hemoglobin more than oxygenated hemoglobin. Conversely, the infrared light is absorbed by oxygenated hemoglobin more than reduced hemoglobin.
The other side of the probe is the sensor, or photodiode. It picks up the amount of red and infrared light that was not absorbed by the reduced and oxygenated hemoglobin. Software in the monitor itself takes the data received and translates it into a number and a waveform for us.
But remember that it is called a pulse
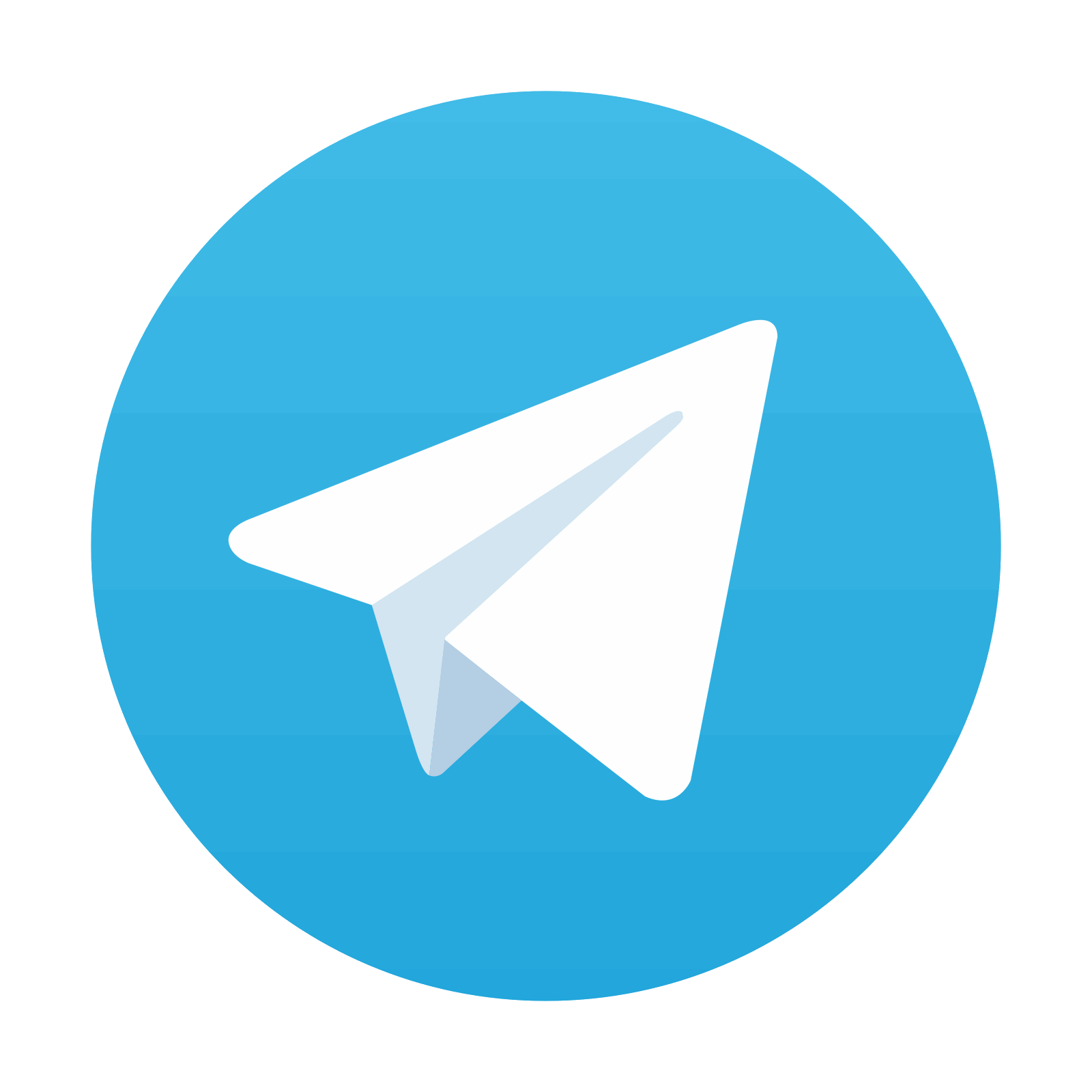
Stay updated, free articles. Join our Telegram channel

Full access? Get Clinical Tree
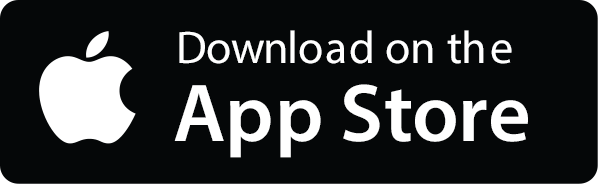
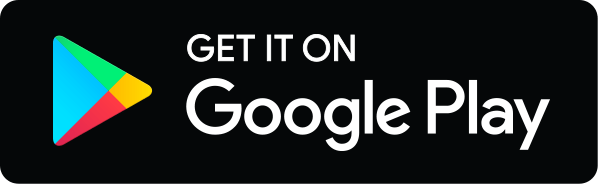