Over the past three decades, significant advances have been made with respect to the evolution of prosthetic heart valves and the manner in which they are evaluated in vivo. New materials and configurations of mechanical and biologic valves have improved the quality of life for patients around the world. However, despite the benefits they confer by replacing a diseased valve, all prosthetic valves have inherent limitations and possible complications that may develop in the immediate or late post-implantation period. This chapter provides an overview of (1) the clinical indications for implantation of prosthetic heart valves; (2) the major types of prostheses and (3) their evaluation using transesophageal echocardiography (TEE); (4) some inherent limitations of echocardiography; and (5) common prosthetic pathology.
Similar to the assessment of native cardiac valves (see Chapters 7, 9, and 10), TEE can provide a detailed evaluation of the structure, function, and integrity of prosthetic valves using two-dimensional (2D), color-flow Doppler (CFD), continuous-wave Doppler (CWD), pulsed-wave Doppler (PWD), and three-dimensional (3D) imaging modalities. However, several features unique to the intraoperative environment such as dynamic changes to preload, myocardial contractility, and afterload can create challenges for the TEE assessment of prosthetic valves. In addition, prosthesis malfunction can affect adjacent valves and chambers, and this must be considered in the assessment. Nevertheless, the integration of information from the TEE examination can provide a comprehensive assessment of the patient’s overall cardiac status.
A diseased native valve that is symptomatic will ultimately require intervention (repair or replacement) by percutaneous or surgical means. The most commonly replaced valves are those in the aortic and mitral positions commensurate with the high prevalence of diseases that involve these valves. Recently, with significant improvements in mitral valve repair, the numbers of valves implanted for mitral regurgitation have been decreasing and stenosis has become a more common indication for mitral valve replacement. In addition, with a large number of prosthetic valves already implanted, pathologies involving these replaced valves are increasing the frequency of repeat surgery. Often presenting as new or increased valvular regurgitation in the setting of a systemic infection, endocarditis of the native valve or prosthetic grafts is an infrequent yet important indication for placement of a new prosthesis. In addition, inadequate anticoagulation can lead to acute or chronic stenosis of previously placed valves. The myriad of systemic diseases that can cause valvular dysfunction are comprehensively discussed in the ACC/AHA guidelines for evaluation of patients with valve disease.1
When faced with a patient who needs a valve replacement, cardiologists and cardiac surgeons have a wide variety of valves from a number of manufacturers to select from. The decision as to which prosthesis should be implanted depends on a number of factors including the patient’s age, gender (women of child-bearing age), life expectancy, comorbidities, location and size of the diseased valve, surgical expertise or preference, and considerations regarding anticoagulation (noncompliance or likely loss to follow-up).2 A detailed description of the process of valve selection is beyond the scope of this text and is discussed elsewhere.1
All valves can be classified as mechanical or biologic depending on the predominant material of composition. While mechanical valves are further classified according to the occlusion device, biologic valves are subclassified according to the presence of synthetic support structures (stented or stentless) and by the origin of the valve tissue (xenografts, homografts, or autografts).2–4
Mechanical valves are available in a variety of sizes and designs. All mechanical valves are comprised of a sewing ring (used to secure the valve in the patient) and an occluding device (designed to provide one-way flow through the prosthesis). The design of the occluding device is used to further subclassify this type of prosthesis into one of three major categories: (1) ball-in-cage, (2) tilting disk, and (3) bileaflet.
The first successful prosthetic heart valve implantation in the early 1960s was of the ball-in-cage design. The Starr-Edwards valve was the most widely used, with more than 200,000 prostheses placed since its introduction into clinical practice in 1965.3,5 Although it has undergone several modifications, the basic design remains unchanged, with a silastic ball constrained within a sewing ring comprised of Teflon or polypropylene cloth and two stellite alloy U-shaped arches that form a cage (Figure 11–1). The ball travels forward into the cage during antegrade flow, with the blood passing between the sewing ring and under the trailing edge of the ball between the struts. The occluder then moves back to seat snugly against the sewing ring when pressures equalize between the two chambers. There is no regurgitant flow during elevation of pressures in the receiving chamber.
Their bulky design and flow characteristics (the smaller the valve, the more obstructive in nature the valve becomes) limit their use to the mitral position. They are also not typically implanted in individuals with small left ventricular cavities or with small aortic annular diameters. Although durable and therefore still seen in patients presenting for repeat surgery, these valves are no longer implanted, having been supplanted by valves with a better hemodynamic profile.
Representatives of this design include the Medtronic-Hall (Medtronic, Minneapolis, MN), OmniScience (MedicalCV Inc., Inver Grove Heights, MN), and the Bjork-Shiley valves (Figure 11–2). Although each of these valves has its own distinctive design features, a typical tilting disk valve is comprised of a circular sewing ring and an eccentrically hinged or pivoting disk occluder. The pyrolytic carbon disk opens to show two unequal (major and minor) orifices during antegrade blood flow. With reversal of pressures, retrograde flow against the larger leading portion of the disk results in closure by rotation on its hinge or pivot.
The Medtronic-Hall valve, first used clinically in 1977, is the most commonly implanted tilting disk valve and is second only to the St. Jude bileaflet valve as the most frequently implanted mechanical prosthesis. The pyrolytic carbon disk has a small central orifice through which the eccentrically placed hinge mechanism passes. The disk and struts are enclosed within a titanium housing that is attached to the native valve annulus with a Teflon sewing ring. The maximum opening angle of an aortic Medtronic-Hall valve is 75°, whereas a mitral prosthesis generally opens no more than 70°.3,6 The fact that the occluder disk opens less than 90° generates resistance to forward flow and small eddies of stagnant flow proximally that predispose to thrombus development. The small hole in the occluder mechanism results in a characteristic central regurgitant jet that is visible with CFD.
The OmniScience valve also consists of a pyrolytic carbon disk suspended within a titanium housing; however, the sewing ring is comprised of polyester knit rather than of Teflon. Unlike the Medtronic-Hall valve, the OmniScience prosthesis does not have a central hinge; rather, the motion of the disk is restricted by a series of struts. Because there is no central hinge and prerequisite hole, the OmniScience valve does not have a central regurgitant jet when in the closed position. The maximum opening angle of this design is approximately 80°.2
The Bjork-Shiley prosthesis, the first successful low-profile tilting disk design (1969), is no longer available in the United States because of problems with strut fracture. However, more than 360,000 valves of this type were implanted, so one occasionally may encounter a patient with this design in situ. The disk of this design has a convex-concave design, with a maximum opening angle of 70°.2
The major representatives of the bileaflet design are the St. Jude (St. Jude Medical, St. Paul, MN), the Carbomedics (Sulzer Carbomedics, Austin, TX) and the On-X (On-X LTI, Austin, TX) prostheses (Figure 11–3). The St. Jude bileaflet prosthesis is the most widely used mechanical valve in the world, with more than 600,000 implantations since its introduction in 1977. Each of these prostheses has two semicircular pyrolytic carbon occluders attached by small midline hinges to a support ring (pyrolytic carbon ring in St. Jude) and a Dacron sewing cuff. The manner in which the two occluder disks are hinged means they require no supporting struts, and therefore have an extremely favorable flow profile with lower transvalvular gradients as compared with the ball-in-cage and single tilting disk designs at similar annular diameters.3 With antegrade flow, both leaflets open to a maximum angle of 85°, resulting in two large, semicircular lateral orifices and a much smaller central rectangular orifice. With sufficient back pressure, the leaflets rotate on their hinges to close with an angle of approximately 25° to the plane of the supporting ring.2
The Carbomedics prosthesis is similar to the St. Jude in design; however, it has an adjustable titanium housing that can be rotated to position the leaflets in such a manner that they avoid contact with subvalvular tissue. The On-X valve is a newer valve made of pure pyrolitic carbon with a longer flow channel and an inlet that is flared outward to improve the flow dynamics through the valve. In addition, two leaflet guards are present to limit pannus encroachment onto the leaflets.7
Biologic tissue valves may be stented or stentless, depending on the presence of synthetic support structures. They may also be composed of either porcine or bovine leaflet tissue (xenografts), human cadaveric tissue (homografts), or native human tissue (autografts, as in the Ross procedure). Some biologic valves contain a mix of synthetic and natural biological materials (heterografts). Frequently, xenografts or homografts, especially for the aortic position, will include surrounding aortic tissue for improved natural structural support (composite grafts). The principal advantage of biologic over mechanical valves is related to the need for anticoagulation: mechanical valves require long-term anticoagulation, whereas biologic valves require only a short period (8 to 12 weeks) during endothelialization of the sewing ring.
Stented biologic valves combine synthetic structural and supporting elements, with leaflets comprised of porcine valve leaflets (Hancock [Medtronic, Minneapolis, MN] and Carpentier-Edwards [Edwards Lifesciences, Irvine, CA] valves) or shaped pericardial tissue (Ionescu-Shiley [discontinued] and Carpentier-Edwards Perimount [Edwards Lifesciences, Irvine, CA] valves; Figure 11–4). The biologic elements of these valves are treated with glutaraldehyde to reduce antigenicity and increase tissue strength. However, this same process renders the tissue less pliable than native human valves and can promote calcification and degeneration in the long term.
The porcine valve leaflets in the Carpentier-Edwards prosthesis are mounted on a flexible Elgiloy frame, with stents manufactured from a single piece of wire attached to a Dacron sewing ring. The individual leaflets are mounted above the sewing ring, which allows for a larger orifice area and an improved hemodynamic profile as compared with valves with a similar ring size. However, the addition of the supporting struts (stents) does reduce the effective orifice area (EOA) as compared with a native human valve.8 The Hancock valve has many similarities to the Carpentier-Edwards valve on gross visual inspection. Nevertheless, each has a distinctive radiographic shape, with the Carpentier-Edwards valve appearing as a crown and the Hancock valve appearing as a circular ring because its stents are constructed from polypropylene, which is not radiopaque. During antegrade flow, the valve leaflets assume a somewhat irregular cone shape secondary to the slight restriction in opening caused by the valve stents. With pressure reversal, the leaflets coapt and commonly show a small central regurgitant jet, even in a normally functioning biologic valve.
Pericardial biologic valves (Ionescu-Shiley and Carpentier-Edwards Perimount) are not restricted by the physiologic size of the donor valve leaflets, thus allowing for the construction of larger valves. The Ionescu-Shiley valve was a low-profile prosthesis with three bovine pericardial cusps mounted to a Dacron-covered titanium frame using retention sutures. This prosthesis had difficulties with leaflet dehiscence and was discontinued after 10 years. The Perimount valve by Carpentier-Edwards has its pericardial leaflets mounted within the stent to maximize leaflet opening and to reduce abrasion between the leaflet and the stent.8
The development of stentless bioprostheses progressed with the desire to improve hemodynamics and long-term durability and preserve the benefits afforded by a tissue valve. The removal of the stent and sewing cuff permit the implantation of a relatively larger valve as compared with a stented biologic or mechanical valve of the same circumference. In addition, removal of the stent appears to significantly reduce stress at the base of the leaflets, which reduces calcification and slows valve degeneration. These valves are used only in the aortic position in which the patient’s annulus and aortic root provide support and flexibility to the implanted materials. The typical stentless valve is comprised of an intact porcine aortic valve with an outer layer of polyester fabric to lend support and to facilitate implantation (Figure 11–5). Prior to the addition of the fabric, the valves are processed at very low fixation pressures to maintain collagen pliability. In addition, the Medtronic Freestyle valve is treated with amino-oleic acid to decrease calcium deposition. Several stentless valves are currently available, including the Toronto SPV (St. Jude Medical, St. Paul, MN), Medtronic Freestyle (Medtronic, Minneapolis, MN), and the CryoLife-O’Brien (CryoLife, Kennesaw, GA). These valves are technically more challenging to implant, and the exact surgical technique depends on how much of the recipient’s aortic root and native sinus tissue are used in the process (full root technique with reimplantation of the coronary arteries, the root inclusion technique with preservation of the native coronary arteries, or a complete subcoronary or modified subcoronary technique).
The operative mortality rate for the stentless valves is slightly higher than that for other biologic valves (3% to 6%) partly due to the increased complexity of surgical technique. However, stentless valves have a very favorable 12-month complication rate (low rates of endocarditis, thrombosis, and hemorrhage) and survival rate of 91 ± 4% at 6 years. Currently, the engineered durability has held true, with no reported structural failures.9
Homografts (human cadaveric tissue) are collected from the aortic and pulmonic positions within 24 hours of donor death and are treated with antibiotics before sterilization and preservation in liquid nitrogen at –196°C. These valves provide excellent hemodynamics, have low rates of thrombogenesis, are relatively resistant to infection, and are therefore ideal for use in the setting of acute native or prosthetic endocarditis.10,11 However, these cryopreserved valves are subject to accelerated degeneration as compared with native and mechanical valves. In addition, they require ongoing cryopreservation, have limited availability (most institutions carry a limited number of sizes, if at all), and require additional surgical time if the valve is not sized and thawed until the annulus is directly measured after arrest of the heart rather than relying on TEE valve sizing.12 The implantation of a homograft requires a highly skilled surgeon. Mitral homografts have been attempted in the past, but are no longer common.
Pulmonary autograft for aortic valve replacement (Ross procedure) uses the patient’s native pulmonic valve and proximal main pulmonary artery to replace the diseased aortic valve and ascending aorta, with reimplantation of the native coronary arteries into the neo-ascending aorta. A stentless homograft (or other bioprosthesis) is usually placed in the pulmonic position so that anticoagulation is not required in the long term. Autografts are used most commonly in children, adolescents, adults with a longer than 20-year life expectancy, and those individuals in whom long-term anticoagulation is contraindicated or unwanted because of lifestyle factors.2,3 These valves have excellent hemodynamic profiles and are extremely durable with the “life expectancy” of a native valve. Limitations to its use include a technically more complex operation with the necessary replacement of two heart valves and the degeneration and/or obstruction of the new “pulmonic” valve and proximal pulmonary artery necessitating reoperation.
Endovascular transcatheter aortic valve implantation (TAVI) has recently become an option for those patients with calcific aortic stenosis who were previously deemed to be too high risk for a conventional valve replacement. The combination of advanced age with multiple comorbidities including renal, pulmonary, and cerebrovascular dysfunction increases the operative risk to over 25%.13 Following the first successful human implant in 2002, several different techniques have been developed (antegrade–transapical, and retrograde–transarterial) to more safely position and deploy the expandable bovine pericardial valves.
Currently there are two types of TAVI valves. The balloon expandable bovine pericardial “Sapien” valve (Figure 11–6) (Edwards Lifesciences, Irvine, California) is mounted onto a stainless steel stent and comes in two sizes (23 and 26 mm). The 23-mm valve is preferred for annular diameters of 18 to 21 mm, and the larger 26-mm valve for larger annular diameters up to 24 mm. The CoreValve (Medtronic, Minneapolis, MN) is also manufactured using bovine pericardium, but it is mounted on a self expanding Nitinol stent and is available in two sizes: a 26-mm valve for an annulus diameter of 20 to 23 mm, and a 29-mm valve for a 23- to 27-mm annulus. The valve chosen must be larger than the annulus diameter to reduce the risk of paravalvular leaks while allowing adequate anchoring of the valve system.
Proper positioning of these valves is critical. Incorrect positioning can lead to embolization into the left ventricular cavity or anywhere along the aorta, paravalvular leaks, coronary ostial obstruction, or interference with the mitral valve (native or prosthetic). Both fluoroscopy and TEE are utilized in many centers, with TEE becoming the preferred imaging modality because of its versatility. TEE allows for a full assessment of ventricular function, a complete evaluation of all valves, sizing of the annulus and other structures, measurement of gradients, and quantification of trans- or paravalvular regurgitant jets. Echocardiography can also be utilized to direct the advancement of guidewires and delivery devices prior to and during the placement and delivery of the valve.14
The evaluation of patients with prosthetic valves begins with an understanding of the patient’s symptoms, which often provide clues to the expected pathology. It is also important to record the blood pressure, heart rate, and cardiac output (when available) since Doppler-derived gradients are dependent upon flow and diastolic filling time. Finally, the height, weight, and body surface should be noted in order to assess patient-prosthesis mismatch and cardiac chamber size.15
Evaluation of prosthetic heart valves using TEE can be technically challenging, but it provides a vitally important means to image and interrogate the normal and abnormal structure and function of an implanted valve prosthesis. Rather than being a “standalone” technique, TEE often complements methods such as fluoroscopy, magnetic resonance imaging, transthoracic echocardiography (TTE), and catheterization studies in the evaluation and routine follow-up of patients with prosthetic heart valves.1 TEE is minimally invasive, especially in the intraoperative setting, and provides superior 2D images as compared with traditional TTE because of the use of higher-frequency probes, the proximity of the ultrasound beam to the area of interest, and the lack of intervening structures to absorb or interfere with the beam. The development of real-time three-dimensional TEE (RT3DTEE) has also been shown to be beneficial in the assessment of indwelling prostheses.16,17
Synthetic materials (metals, plastics, fabrics, and pyrolytic carbon) on prosthetic valves present distinct challenges to a thorough echocardiographic examination. Interference with ultrasound transmission and reflection can produce several types of artifacts that make comprehensive assessment challenging. While mechanical valves are more prone to imaging artifacts, biologic valves also include synthetic components that can generate similar difficulties. Ultrasound artifacts are directly related to the reflective and absorptive properties of the prosthetic materials employed. In addition, the speed of sound through the synthetic materials may be faster or slower than that through human tissue, which can lead to alterations in the displayed size and location of the image. One must always keep these artifacts and imaging limitations in mind to avoid the misinterpretation of information that can result in false or missed diagnoses.
Reverberation artifact and acoustic shadowing (see Chapter 3) can conceal anatomic structures distal to the reflector and often render CFD evaluation impossible. The ability to place the transducer directly posterior to the left atrium with TEE allows for an unobstructed view of the mitral surface of the prosthesis and a comprehensive Doppler evaluation. From the midesophageal position, all shadowing and reverberation artifacts reside on the ventricular aspect of the valve. Similarly, the transgastric and deep transgastric views usually allow for the evaluation of the ventricular aspect of the valve, with the artifacts now located within the atria.
TEE imaging of prosthetic valves is not different from regular ultrasound imaging. The best 2D images are generated when the reflector is perpendicular to the ultrasound beam, whereas accurate Doppler studies require an angle of interrogation of less than 20° from parallel to the jet or flow. The measurement of transprosthetic gradients and flows using Doppler ultrasound follows the same limitations and caveats described in Chapters 7, 9, and 10, namely that it may be difficult to obtain the proper position or angle to locate the maximal jet or flow. The multiplane transducer frequently needs to be rotated to adjust the ultrasound beam so that imaging artifacts are minimized and proper identification of normal and pathologic structures can be elucidated. The acquisition of 3D images is described in Chapter 24.
While all prosthetic valves are obstructive by the nature of their design, each class and subclass of valve has unique echocardiographic structural characteristics that can be used to identify the specific type, and subsequently the proper function, of the valve. Regardless of the type of valve undergoing evaluation, a complete examination includes imaging the area in question with a variety of views to obtain an accurate assessment. Although usually not immediately available in the operating room setting, comparisons with previous studies can be invaluable in the evaluation of a prosthetic valve. Flow patterns and transvalvular gradients can change over time and new findings should be compared with previous measurements.18,19
Additionally, there are special considerations unique to the intraoperative setting. Loading conditions often fluctuate in the immediate post-CPB phase, making transvalvular gradients difficult to quantify. Moreover, frequent changes in inotrope levels and pacing strategies also result in changing hemodynamics. These must all be considered while interpreting the results of Doppler-based measurements.15
The sewing ring (if any) should be examined for movement. A rocking motion is suggestive of valve dehiscence, loose or fractured sutures, or a perivalvular abscess. The periannular area is often somewhat obscured by calcification or artifacts from the valve itself. However, it is important to check for small periannular or perivalvular echolucent areas that may indicate fistula or abscess formation. Frequently, the aortic root can appear thickened as a result of hematoma or edema after surgery. This appearance may be prone to misinterpretation and should be distinguished from an abscess.15 Next, valve leaflet or occluding device motion is evaluated. Mechanical occluders should move rapidly and extend to the full limit of the design specifications. Restricted or incomplete opening is suggestive of primary valve dysfunction or of an obstructing material or object (suture material, perivalvular tissue, pannus, thrombus, endocarditis, vegetations, or masses). In addition, residual periannular materials may interfere with the proper closure of the occluders of mechanical prostheses resulting in significant regurgitation through the valve (Figure 11–7). The leaflets on a biologic valve should be thin and have the same appearance as native nondiseased leaflets. A close inspection for tears, perforations, thickening, and calcification should be carried out. Information from the rest of the TEE examination should be incorporated into the assessment of the prosthetic valve. Left atrial “smoke,” or thrombus, is suggestive of a functionally stenotic mitral prosthesis. Similarly, LV dilation or LV hypertrophy with new native mitral regurgitation could be suggestive of prosthetic aortic regurgitation or stenosis, respectively.
Figure 11-7.

Periannular tissue (remnant of posterior leaflet; arrow) trapped between sewing ring and occluder of a dual tilting disk mechanical prosthesis. The patient had moderate mitral regurgitation within the sewing ring on TEE immediately following the termination of CPB. Subsequent excision of the redundant tissue resulted in no regurgitation other than the washing jets.
Infrequently an intraoperative TEE exam cannot be performed because either a TEE probe could not be inserted or there was a contraindication to probe insertion. In these circumstances, the evaluation of prosthetic valves can be performed using epicardial echocardiography (EE). The performance of a complete EE exam is discussed further in Chapter 20 and in the guidelines published by the American Society of Echocardiography and the Society of Cardiovascular Anesthesiologists.20 Although not specifically outlined in these guidelines, the majority of normal cardiac structures (including prosthetic materials) can be visualized using a high-frequency epicardial ultrasound probe.
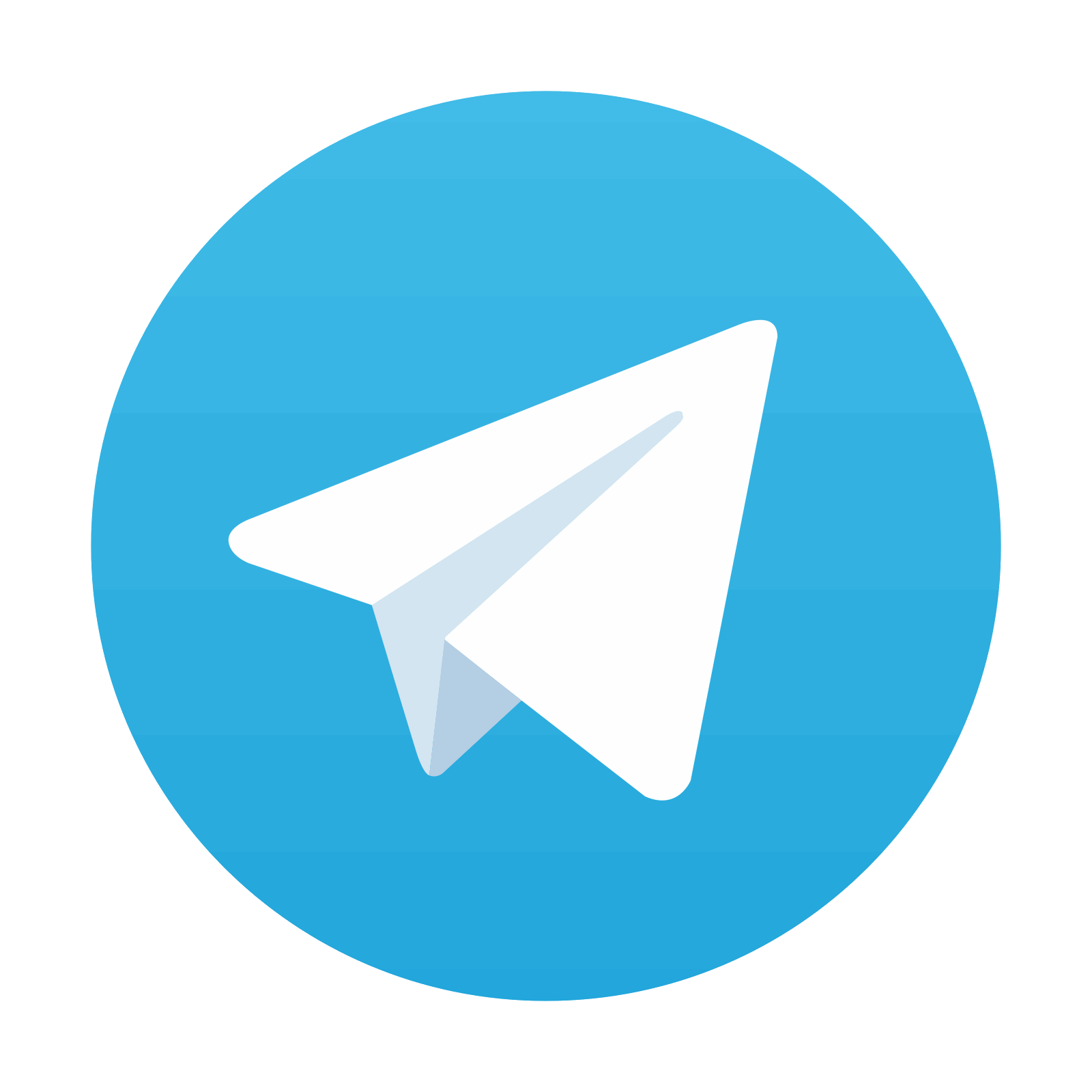
Stay updated, free articles. Join our Telegram channel

Full access? Get Clinical Tree
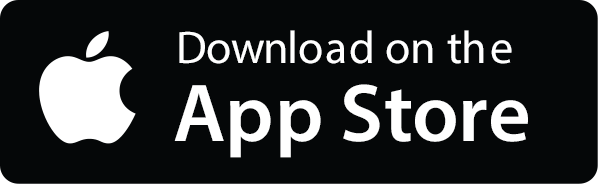
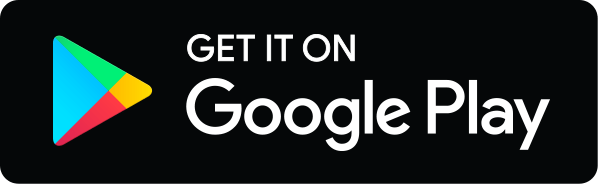