Chapter 13 Principles of rehabilitation and considerations for sport, performance and fitness
Introduction
It is now widely accepted that for most individuals, well-designed exercise programmes and participation in appropriate physical activity not only reduce the risk of ill health, but are also an effective tool for improving the health of asymptomatic and symptomatic individuals (Bird et al 1998, Woolf-May 2006).
There is increasing evidence to support the beneficial effects of exercise in the management of individuals with joint hypermobility syndrome (JHS). Kerr et al (2000) report a good response to a daily monitored and modified exercise programme in children, and Shoen et al (1982) and Ferrell et al (2004), reported similar beneficial effects in adults. In their case studies, Simmonds and Keer (2008) and Hinton (1986), report favourable outcomes using carefully prescribed exercise as a primary intervention along with behaviour modification and lifestyle advice in both adults and adolescents.
The management of JHS can be complicated. Not only is there the challenge of improving static and dynamic joint stability and exercise tolerance, but also the associated problems of chronic pain and fatigue. Furthermore, joint hypermobility (JHM) is associated with fibromyalgia (FM) in adults and children (Chapter 5) (Goldman 1991, Gedalia et al 1993, Acasuso-Diaz & Collantes-Estevez 1998, Ofluoglu et al 2006, Sendur et al 2007).
Nevertheless, exercise plays a significant role in reducing symptoms. In the study by Goldman (1991), between 79% and 85% of the hypermobile patients diagnosed with FM displayed significant symptomatic improvements after performing a regular exercise programme. Although Cherpel and Marks (1999) cite criticisms of the study design, they make the comment that it is one of the few published papers demonstrating the effects of exercise on individuals with JHM and FM and its potential for modifying the course of the syndrome. In addition, Busch et al (2008) report ‘gold’ level evidence for beneficial effects of supervised aerobic exercise training on physical capacity and FM symptoms, in their impressive systematic review of 34 studies. The research also suggests that strength training may be beneficial for some FM symptoms.
Physical therapies that have not taken account of the presence of JHM or JHS may either be non-beneficial or detrimental (Hakim & Ashton 2005).
The principles of rehabilitation, health and fitness
Physical fitness
Rehabilitation and fitness reconditioning programmes are dynamic systems of prescribed exercise and activities aimed at reversing and preventing the deleterious effects of injury, disease and inactivity. They aim to restore the individual to their full health and physical fitness potential. The definition of health has been updated as not just being a disease-free state, but has been extended to include the physical, mental, social, emotional and spiritual state of the individual on a continuum from near death to optimal functioning (Bird et al 1998).
Physical fitness has been described by Lamb (1984) as ‘the capacity of the individual to successfully meet the present and potential physical challenges of life and comprises of elements, such as flexibility, strength, anaerobic power, speed, and aerobic endurance’. Other parameters of balance, skill and co-ordination, speed and muscular endurance may also be added to give an even more comprehensive definition.
Research has repeatedly demonstrated that regular participation in appropriate physical activity or exercise benefits all members of the population, regardless of age, gender or whether they suffer from recognized medical conditions or not (Bird et al 1998, Dishman et al 2006, Woolf-May 2006). The benefits of exercise are listed in Box 13.1.
Exercise prescription
Joint instability has been suggested as the underlying cause of microtrauma and subsequent joint and muscle pain in children with JHS (Gedalia et al 1993). It may be further postulated that it is responsible for at least some of the pain experienced in the adult JHS population. Joint stability is determined by the efficient functioning of the neuromusculoskeletal system. Deficiency in any part of this system, resulting from inflammatory disease, trauma or neural impairment, compromises stability (Kerr et al 2000). Panjabi’s model of spinal stability (Panjabi 1992a, 1992b) explores the interrelation between passive and dynamic joint stabilizers and the neutral zone and provides us with a good biomechanical model for understanding spinal and joint stability (Chapter 12.8). This theory, in combination with our growing understanding of the effects of pain on muscle inhibition, motor control and subtle neurophysiological abnormalities, often associated with JHS (Ferrell et al 2007) (Chapter 6.4), helps us to understand the susceptibility to instability, which individuals with generalized tissue laxity and JHS experience.
Tissue response to immobilization and reduced physical activity
Connective tissue
The effects of immobilization on connective tissue have been studied extensively. Animal studies on rabbit knees by Akeson et al (1980) showed changes in collagen, glycosaminoglycans (GAG) and water content. It was also observed that the connective tissue when immobilized appeared ‘woody’, rather than glistening and this was believed to be due to water loss. Theories regarding the pathomechanics of joint contracture suggest that the loss of water and GAG increased the space between the collagen fibres, thereby altering the amount of free movement between fibres. This lack of movement made the tissue less elastic, less plastic and more brittle; the implication being that capsular structures, including ligaments, would fail at lower loads after periods of immobilization.
Ligaments
In addition to the effect of immobilization on connective tissue, specific changes in ligaments as a result of immobilization have been well documented. Ligaments, like bone, remodel as a response to mechanical stress. The effects of immobilization on knee joint ligaments have been widely researched and it is now well established in the literature that the amount of time the ligament is immobilized is much shorter than the amount of remobilization time necessary for the ligament to reach its full pre-immobilization strength. The ligaments become stronger and stiffer when subject to load. A study on the anterior cruciate ligaments of rats revealed that inactivity and rest resulted in weaker, less-compliant tissue (Cabaud et al 1980). Again, early animal studies performed on dogs and primates suggest that the alterations in the mechanical properties of ligaments are a result of increased osteoclastic activity at the bone–ligament junction rather than actual ligament atrophy (Laros 1971, Noyes et al 1974). These alterations lead to a decrease in tensile strength and reduce the ability of ligaments to provide joint stability.
Bone
Equilibrium of bone absorption and accretion are maintained by weight-bearing, muscular contraction, adequate nutrition and hormonal status. The rate of bone cell turnover decreases after 10–15 days of immobilization. Loss of bone density is evidenced by a loss of the normal trabeculae pattern. The mechanical properties of bone, including elastic resistance and hardness, steadily decline with increased duration of immobilization (Burdeaux & Huychinson 1953, Landry & Fleisch 1964, Hardt 1972). By 12 weeks of immobilization, bone hardness is reduced by 55–60% of normal. Most of these losses are recoverable by muscle contraction and weight bearing in a comparable period of time (Steinberg 1980). However, they will be influenced by adequate nutrition and hormonal status. Bed rest studies and space research have increased our understanding of the effects of loading on bone metabolism. Reduced physical activity prevents the normal mechanical load signals that act to maintain bone balance, resulting in increased bone resorption and insufficient bone formation. Temporary bed rest has been shown to result in bone loss averaging 1% per week (Donaldson et al 1970, LeBlanc et al 1990). Bone loss is proportional to the relative changes in loading with greater percentage of loss. A greater percentage of bone loss is seen in the lower limbs than upper limbs when physical activity is reduced.
Muscle
The effects of immobilization and inactivity on muscle have been thoroughly investigated and reported in the literature. Changes include the following; a decrease in fibre size, changes in sarcomere alignment and configuration, and reduced muscle mass (MacDougall et al 1977, Booth 1987, Harrelson 1998). Mitochondria are also reduced in number, size and function and together with the histochemical changes that occur with immobilization, result in a reduction in the oxidative capacity of muscle (Booth & Kelso 1973, MacDougall 1977, Booth 1987). This increases the fatigability of muscles and reduces muscle endurance, which has been shown to occur as early as 7 days post immobilization (Rifenberick & Max 1974).
Disuse atrophy occurs in both fast-twitch (type I) and slow-twitch (type II) muscle fibres, but it is generally accepted that there is greater degeneration in slow-twitch fibres (Harrelson 1998). The rate of muscle atrophy is greatest in the first 5–7 days of the immobilization period, after which the rate of loss slows significantly (Booth 1987).
Muscle atrophy resulting from immobilization appears to be selective. Like bone, muscle loss is proportional to function. For example, muscle associated with postural joint stability (vastus medialis obliquus (VMO) and hip abductors) appears to atrophy at a faster rate than muscle with a more global stabilizing function, and is commonly seen in the deconditioned JHS population. Muscles also atrophy as a result of reflex inhibition due to injury, pain and fear of pain, which are often significant factors in this client group, as well as inflammation (Harrelson 1998), which is less common.
Nerve
Central and peripheral nervous system changes including a reduction in the production of neurotrophic enzymes and reduced dendritic branches in the motor cortex (Chapter 3), reduced dopamine release and/or loss of dopamine receptors in the brain have been observed in association with reduced physical activity (Dishman et al 2006). These changes may result in an alteration in muscle fibre recruitment patterns and timing, leading to altered movement patterns and reduction in co-ordination and skill. Empirical data, however, suggest that there is a neural reflex causing muscle atrophy resulting from joint damage and immobilization (Engles 1994, Harrelson 1998).
Clinical implications of immobilization
Bone that has become osteopenic or osteoporotic as a result of immobilization is less able to bear weight or withstand the normal forces of compression, tension and shear. Due care must be taken when remobilizing (using manual techniques and exercise), particularly in vulnerable groups: the elderly, post-menopausal women and individuals who have been taking long-term steroids. Individuals with JHS may also be considered a risk group as there is evidence to show that low bone density is associated with Ehlers-Danlos syndrome (EDS) hypermobility type (formerly type III) and JHS (Mishra et al 1996, Nijs et al 2000). It can be postulated that in the case of JHS, reduced activity due to pain or following injury may confer a significant risk for developing osteoporosis, particularly in post-menopausal women. Osteoporosis is a very large public health burden (WHO 2003) and the prevalence and cost associated with managing the disease are expected to escalate, therefore addressing the issue of bone health early in a person’s life is very important.
Immobilization and inactivity of muscles results in weakness due to loss of muscle mass. Additionally, muscle stiffness occurs with the laying down of irregular cross-linked connective tissue. Postural slow-twitch muscle fibres crossing one joint tend to atrophy the fastest (Engles 1994). This will be evidenced in activities requiring sustained or repeated muscle contraction, i.e. standing, sitting and walking. Such changes also contribute to joint instability, a common feature in JHS. Rehabilitation should therefore focus initially on developing the endurance capacity and strength of the local postural antigravity muscles.
It has been suggested that abnormality of collagen synthesis is the pathogenic root of JHS (Child 1986). If this already fragile connective tissue is further compromised in terms of strength and stiffness as a result of immobilization, inactivity and deconditioning, it could have a major impact on joint stability for individuals with JHM. Changes in the muscle and connective tissues, in addition to alterations of neurodynamic function that occur as a result of de-conditioning and immobilization, may provide some explanation of the observation in clinical practice of individuals with JHS who have become inactive because of pain or injury often displaying considerable joint instability, reduced muscle strength, endurance, poor proprioception and vulnerability to injury.
Tissue response to remobilization, exercise and physical activity
Most studies investigating the response of bone and soft tissues to mobilization have primarily studied normal subjects. Muscles begin to regenerate within 3–5 days of the start of a reconditioning programme (Cooper 1972, Zarins 1982). Within the first week, muscle weight increases. Witzmann et al (1982) report that muscle strength can be regained by 6 weeks of exercise, however, normal muscle weight may not be fully achieved until 3 months. Early strength gains are attributed to neurological recruitment pattern changes while longer-term strength gains are observed later when adaptation of tissue has taken place (Sale 1988).
Recovery of bone, nerve and connective tissue occurs at a much slower rate than muscle, due to differences in vascularity. A healing ligament may take between 6 months and 3 years to recover fully, depending on the severity of the injury and length of time it has been immobilized (Tipton et al 1990). Thus, although applying the overload principle to connective tissues in the recovery phase is necessary, it needs to be undertaken with caution. Moderate-frequency, low-intensity endurance exercises have been shown to have a beneficial effect on the mechanical properties of ligaments. It has been suggested that such exercises result in increased collagen production, hypertrophy and realignment of fibre bundles, rendering them stiffer and able to tolerate heavier loads (Woo et al 1982). This claim is supported by the work of Cabaud et al (1980) and Williams et al (1988). Non-weight-bearing isometric exercises, although helpful, are not a substitute for weight-bearing. Future research investigating the tissue response of collagen-defective tissue to exercise will be important in understanding and prescribing exercises for individuals with JHM and connective tissue fragility.
Applied principles of rehabilitation and training
It is important to have a holistic view when designing rehabilitation and fitness programmes for hypermobile individuals. Physiological (Chapter 6), psychological and sociological factors (Chapter 8) need to be considered and addressed. The programme should include well-paced and monitored training to improve (Chapter 9):
It is essential for rehabilitation professionals to have an understanding of motor learning theory and be able to apply these principles to individuals recovering from injury and when reconditioning. It is beyond the scope of this chapter to provide an in-depth overview of motor learning, but the reader is directed to two well-known models which have been proposed. Fitts and Posner (1967) present a three-stage model consisting of a cognitive, associative and autonomous stage and a two-stage model was developed by Gentile in 1972 and further enhanced in 1986. In this model the learner moves from ‘getting the idea’ to ‘fixation and diversification’ in the second stage.
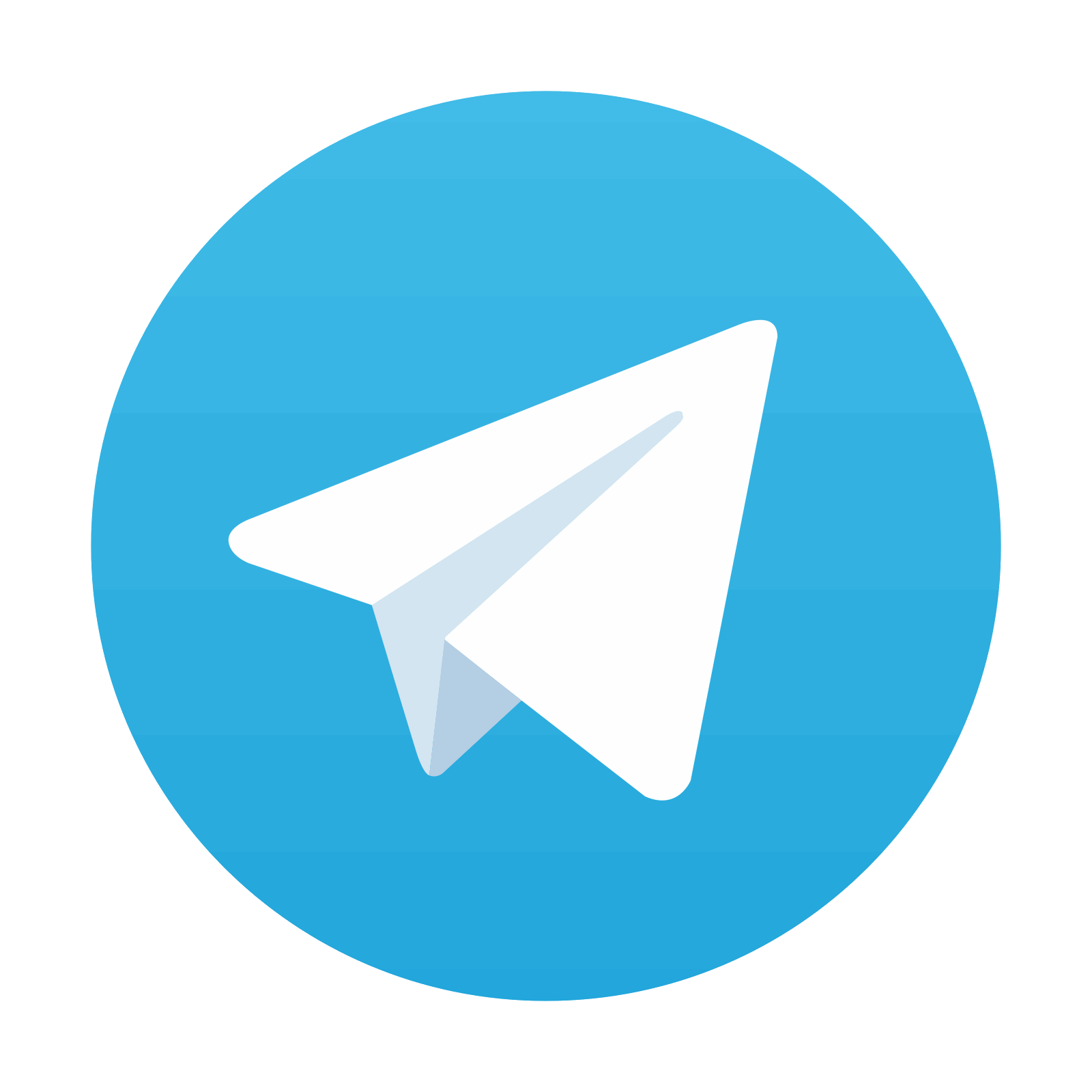
Stay updated, free articles. Join our Telegram channel

Full access? Get Clinical Tree
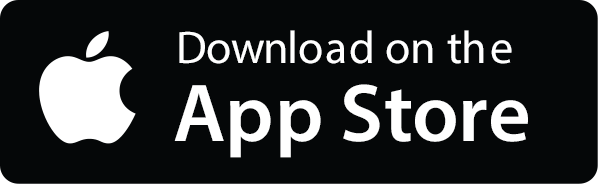
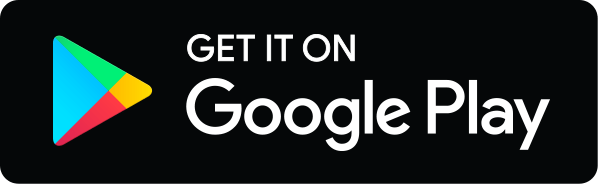