INTRODUCTION
Hypoxemia during emergency airway management is a feared complication and associated with dysrhythmias, hypoxic brain injury, and cardiac arrest. Critical hypoxemia often occurs when providers are focused primarily on laryngoscopy and tube placement rather than gas exchange and oxygenation. Hypoxemia could be avoided in many cases by optimal preparatory oxygenation, but the principles of preparatory oxygenation are often poorly understood and applied.
It is crucial for emergency airway providers to understand that robust preparatory oxygenation as well as the ability to reoxygenate patients with bag-mask ventilation (BMV) (Chapter 9) are the most important aspects of safe emergency airway management. The main goal of airway management is gas exchange, and although this can be accomplished through laryngoscopy and successful placement of an endotracheal tube, preventing hypoxia while this takes place is of critical importance.
Rapid sequence intubation (RSI) (Chapter 20) is the most common method for emergency airway management. Optimal preparatory oxygenation improves patient safety and reduces operator stress after neuromuscular blocking agents have been administered by prolonging the safe apnea time. “Safe apnea time” begins at the onset of paralysis and continues until the patient desaturates below 90%. Safe apnea time varies from several minutes to several seconds, depending on the patient’s body habitus, comorbidities, acuity of illness, oxygen consumption, and oxygen reservoir created through the preoxygenation efforts (Fig. 5-1). A longer safe apnea time allows for unrushed and methodical laryngoscopy and endotracheal tube placement, while attempts at intubation can feel rushed and frantic when oxygenation levels start to fall. The stress of placing the tube correctly before critical hypoxemia ensues can transform what might have been a routine intubation into one complicated by uncertainty and poor technique, even in the hands of skilled and experienced providers.
The goal of preparatory oxygenation is to maximize this period of safe apnea, and facilitate calm and confident intubation success without hypoxemia. In this chapter, we will describe best-practice preparatory oxygenation techniques focusing on oxygen delivery, patient positioning, noninvasive positive pressure ventilation, and apneic oxygenation. We will also discuss when to abandon less aggressive techniques and take control of ventilation and oxygenation with active BMV.
• FIGURE 5-1. Time to desaturation is affected by many factors, including underlying medical condition, age, body habitus, and how well preoxygenation is performed. All patients requiring emergency intubation should be assumed to be at risk for rapid desaturation, and should have maximal preoxygenation. (From Benumof JL, Dagg R, Benumof R. Critical hemoglobin desaturation will occur before return to an unparalyzed state following 1 mg/kg intravenous succinylcholine. Anesthesiology. 1997;87(4):979–982.)
PREOXYGENATION
The goal of preoxygenation is to establish a reservoir of oxygen within the lungs by replacing mixed alveolar gases (mostly nitrogen) with oxygen. The volume available for this “oxygen reserve” is defined by the patient’s functional residual capacity (FRC), which is approximately 30 mL per kg in adult patients. This provides a source of oxygen that the pulmonary circulation can draw from even after the patient is rendered apneic. Ideally, complete pulmonary denitrogenation is achieved, and, if measured, the fraction of expired oxygen (FeO2) would be near or above 90%. In patients with healthy lungs, preoxygenation can be maximized by having the patient tidally breathe the highest fraction of inspired oxygen (FIO2) as possible, ideally an FIO2 of 100%, for 3 to 5 minutes. Alternatively, cooperative patients with healthy lungs can be preoxygenated by having them perform eight maximal volume deep breaths or vital capacity breaths while taking in 100% oxygen. It is imperative that emergency providers understand the differences in FIO2 provided by the variety of oxygen delivery systems common to the emergency department. Preoxygenation is best accomplished with patients in the sitting or head-up position. Patients with lung pathology and decreased functional reserve capacity may require positive end-expiratory pressure (PEEP) for maximal preoxygenation. The details and rationale for these techniques are described in the following section.
High-Concentration Oxygen versus Traditional Supplemental Oxygen
There are widespread misconceptions about the fraction of inspired oxygen (FIO2) supplied by common oxygen delivery devices. Optimal preoxygenation requires delivery of high-concentration oxygen (100% FIO2), but common ED oxygen delivery methods do not provide 100% FIO2 (Table 5-1). The primary limitation with traditional oxygen delivery is the low oxygen flow rate used (≤15 L per minute) and the presence of significant mask leaks.
For spontaneously breathing patients, a bag-valve mask (BVM) and non-rebreather mask (NRM) can both theoretically provide 100% FIO2 with a low source oxygen flow rate (15 L per minute) if they incorporate a reservoir bag, a one-way exhalation valve, and a perfect mask seal. This is hard to accomplish in ED patients, and attempts at oxygenation can be impeded by large volumes of room air entrainment. In low flow systems with an imperfect mask seal, FIO2 decreases as the patient’s minute ventilation and inspiratory flow increase, because the ventilatory demands of the patient are higher than the oxygen delivery provided by the device, and room air makes up the difference.
Low FIO2 Oxygen Delivery Systems |
System | Source O2 Flow Rate (L/min) | FIO2 (approximate) (%) |
Nasal cannula | 2–4 | 30–35 |
6 | 40 | |
Simple face mask | 6 | 45 |
10 | 55 | |
Venturi mask | 15 | 50 |
Non-rebreather mask | 15 | 70 |
Bag-valve mask (BVM) with mask leak or without one-way valve | 15 | <50 |
BVM without mask leak and with one-way valve | 15 | 90–100 |
By altering standard flow rates, accomplished by opening a standard meter to the “flush” rate (>40 L per minute), it is possible to approach an FIO2 of 100%. Table 5-2 lists systems and accompanying flow rates that are able to deliver high FIO2 regardless of the patient’s minute ventilation, inspiratory flow, or mask seal. The key is to deliver 100% oxygen at a flow rate well above the patient’s inspiratory needs, so that room air volume is not required to satisfy the patient’s inspiratory effort.
The oxygen flow rate is likely more important than the oxygen delivery device. Although high flow rates are noisy and somewhat uncomfortable for patients, it allows delivery of high-concentration oxygen using a standard face mask or nasal cannula. In addition, very high oxygen flow rates create a small amount of PEEP, which can improve alveolar recruitment and preoxygenation efforts.
High FIO2 Oxygen Delivery Systems |
System | Source O2 Flow Rate (L/min) | Approximate FIO2 (%) |
Anesthesia machine (flush valve open)a | 30–35 | 100 |
Resuscitation bag (one-way valve, no mask leak) | 15 | 100 |
NPPV machine | (wall O2, ≥50 L/min) | 100 |
High-flow nasal cannula | 40–60 | 100 |
Non-rebreather mask | ≥40 | 100 |
Simple maskb | ≥40 | 70–90 |
aHigh-flow flush compensates for a mask leak.
bFIO2 is less predictable with simple masks because of room air entrainment and turbulent flow.
Nasal Cannula
Low-flow nasal cannula is often the first-line of oxygen supplementation and is appropriate in patients with mild oxygen debt. Common initial flow rates are 2 to 4 L per minute. The traditional teaching was that the maximal effective flow rate for nasal cannula oxygen is 6 L per minute, which delivers a maximal FIO2 of 35% to 40%. In the last several years, oxygen delivery by high-flow nasal cannula (HFNC) has become common. Commercial HFNC systems (Optiflow, Vapotherm, Comfort Flo) provide nearly 100% FIO2 by using flow rates of 40 to 70 L per minute through larger caliber tubing. They also heat and humidify the oxygen, making the high flow rate more tolerable for the patient. HFNC systems have been successfully used to support patients in hypoxemic respiratory failure, although its role in preoxygenation prior to intubation is yet to be defined. The positive benefits of HFNC are washout of upper airway dead space, reduction in work of breathing by providing adequate flow, and modest amounts of PEEP. There is no literature describing the effectiveness of high-flow oxygen through standard nasal cannula tubing, but it is possible that it is more effective at preoxygenating and tolerable for short-term use.
Simple Face Mask
A simple face mask is a non-form-fitting plastic mask that covers the entirety of the nose and mouth. It does not have an external bag reservoir; so the volume of oxygen available for each breath at low oxygen flow rates is only the volume contained within the mask (~100 to 150 mL). Traditionally, a simple face mask was used with flow rates between 4 and 10 L per minute. At these low flow rates, the FIO2 is highly variable and is dictated by the patient’s respiratory pattern and the volume of room air drawn in around the mask. The traditional teaching was that the maximal FIO2 that could be delivered by a simple face mask is approximately 50% (with an oxygen flow rate of 15 L per minute). However, there is good evidence that high-concentration oxygen (FIO2 > 90%) can be delivered by a simple face mask when the source oxygen flow rate is set to a higher level (≥40 L per minute). In addition, high-flow face mask oxygen should theoretically provide the same benefits as a HFNC—washout of upper airway dead space, reduction in work of breathing by providing adequate flow, and mild PEEP. For all of these reasons, some experts believe that a simple face mask with a high flow rate (
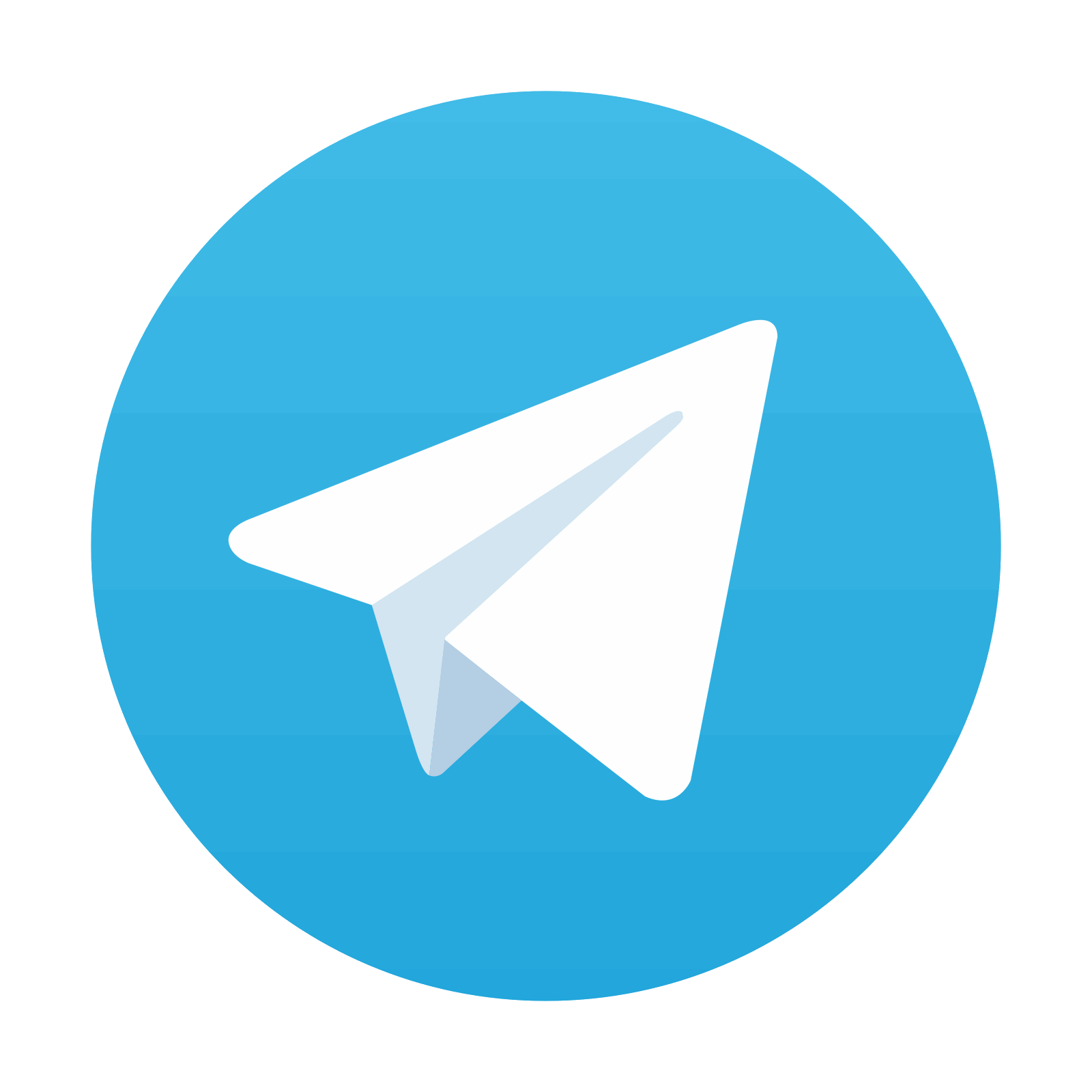
Stay updated, free articles. Join our Telegram channel

Full access? Get Clinical Tree
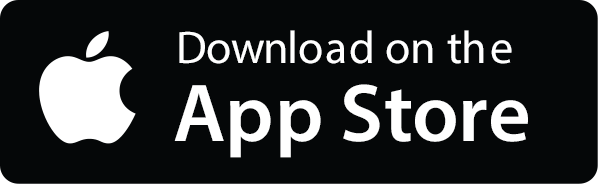
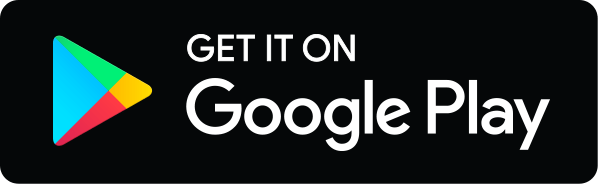