Preterm Labor and Delivery
Carlo Pancaro
Preterm delivery, defined as infant birth at less than 37 weeks of gestation, is the leading cause of infant mortality worldwide (1). The United States preterm birth (PTB) rate rose by more than one-third from the early 1980s through 2006, the year it reached its peak. Since that time, PTB rates have declined significantly in 35 states. Only one state, Hawaii, has reported an increase in PTBs (2). Infant mortality rates for preterm infants are lower in the United States than in most European countries; however, infant mortality rates for term infants (born at 37 weeks of gestation or more) are higher in the United States than in most European countries (3). The very high percentage of PTBs in the United States accounts for its comparatively high infant mortality (Fig. 19-1). The increase in PTBs has been associated with a significant increase in the rate of cesarean delivery, thereby increasing the need for anesthesia providers to be immediately available for these deliveries (2).
In 2006, 12.8% of US births occurred preterm with 3.66% occurring at less than 34 weeks of gestation. Approximately 50% of PTBs are idiopathic. Of these 50%, 30% are related to preterm rupture of membranes (PROM) and 20% are attributed to medical indications or performed electively (4).
The major reason for the increase in PTB is a higher occurrence of multiple gestation pregnancies. Between 1996 and 2002, the multiple birth ratio increased more than 20% to 33% per 1,000 live births (twin birth rate: 31/1,000). Pregnancies complicated by multiple gestation are prone to PTB; approximately 50% of twins and 90% of triplets are born preterm compared to less than 10% of singletons.
Definitions
Full-term birth is defined as one occurring from 39 to 41 weeks of gestation. “Early-term birth” occurs from 37 to 38 weeks of gestation. PTB refers to one that occurs before 37 completed weeks of gestation. PTB may be further classified on the basis of gestational age as follows: Late preterm (34 to 36 weeks and 6 days); moderately preterm (32 to 33 weeks and 6 days); very preterm (≤32 weeks); and extremely preterm (≤28 weeks). Since it is more accurate to correlate infant and neonatal outcomes with birth weight than with gestational age, the scientific community has also used birth weight to define PTB such that low birth weight (LBW) is considered less than 2,500 g, very low birth weight (VLBW) is less than 1,500 g, and extremely low birth weight (ELBW) is less than 1,000 g.
Pathogenesis
Preterm labor may reflect a number of pathogenic processes leading to a final common pathway. The four primary processes are: (1) Premature activation of the maternal or fetal hypothalamic–pituitary–adrenal axis; (2) an exaggerated response to inflammation and infection; (3) decidual hemorrhage; and (4) pathologic uterine distention. These processes are not mutually exclusive and may be initiated long before preterm labor or preterm premature rupture of membranes (PPROM) is clinically diagnosed. In addition, these mechanisms share a final common pathway involving the formation of uterotonic agents and proteases that weaken the fetal membranes and cervical stroma.
Activation of the Hypothalamic–Pituitary Axis
The increased placental production of corticotropin-releasing hormone appears to program a “placental clock” which prematurely activates the hypothalamic–pituitary–adrenal axis in the mother (5,6). In addition, there is an increased fetal pituitary adrenocorticotropic hormone secretion which stimulates the production of placental estrogenic compounds thereby activating the myometrium and initiating labor (7).
Major maternal physical or psychological stressors such as depression can activate the maternal hypothalamic–pituitary axis and have been associated with a slightly higher rate of PTB (8). Women with depressive symptoms early in pregnancy have almost twice the PTB risk compared with women without such symptoms (9). Notably, this risk increases with the severity of depression.
In contrast to women with uncomplicated first pregnancies, women whose first pregnancy ends in PTB are at increased risk of PTB, preeclampsia, and fetal growth restriction in their second pregnancy (10).
Exaggerated Response to Inflammation and Infection
There is a link between PTB and systemic or genitourinary tract pathogens (11,12,13,14,15,16,17). In a large retrospective study of nearly 200,000 deliveries, 2.5% of patients had asymptomatic bacteriuria which was independently associated with PTB (11). The diagnosis and treatment of asymptomatic bacteriuria appears to reduce the risk of PTB (12).
In a different study of 759 women, those without abnormalities of the vaginal flora during their first trimester had a 75% lower risk of experiencing delivery before 35 weeks than women with abnormal vaginal flora (13). The absence of lactobacilli, the presence of bacterial vaginosis, and the presence of gram-positive coccus aerobic vaginitis are associated with a 2- to 3-fold increased risk of PTB. However, treatment of bacterial vaginosis (BV) does not appear to consistently reduce PTB rates in low-risk patients (18). Similarly, periodontal disease is associated with higher rates of PTB, but treatment does not lower this risk (14,15). Lastly, both clinical and subclinical chorioamnionitis are much more common in preterm than in term deliveries and may account for 50% of PTBs before 30 weeks of gestation (19). These data suggest
that disorders of maternal innate or acquired immunity, rather than the mere presence of certain genital tract bacteria, are the primary causes of inflammation-associated PTB.
that disorders of maternal innate or acquired immunity, rather than the mere presence of certain genital tract bacteria, are the primary causes of inflammation-associated PTB.
Bacterial factors trigger a maternal and/or fetal inflammatory response in susceptible individuals that is linked to PTB. This response is characterized by activated neutrophils, activated macrophages, and various pro-inflammatory mediators. Interleukin-1 beta (IL-1 β) and tumor necrosis factor alpha (TNF-α) appear to be the key initial mediators of this response, enhancing prostaglandin production in the amnion and decidua while inhibiting prostaglandin metabolizing enzyme in the chorion (20).
Bacteria also produce phospholipase A2 and endotoxin, substances that stimulate uterine contractions and can cause preterm labor (21).
Furthermore, chorioamnionitis is associated with intense neutrophil recruitment and activation in the decidua. Neutrophils are capable of releasing pro-inflammatory mediators that further enhance decidual and fetal membrane prostaglandin production, thereby recruiting and activating additional neutrophils (22,23). Complement activation also appears to play a role (24). Thus, both maternal and fetal inflammatory responses to infection can lead to preterm labor.
Decidual Hemorrhage
Vaginal bleeding from decidual hemorrhage is associated with a high risk of preterm labor and PPROM (27,28,29). Vaginal bleeding in more than one trimester has been shown to increase the risk of PPROM by 7-fold (27).
The development of PPROM in the setting of abruption may be related to high decidual concentrations of tissue. In laboratory studies, small quantities of thrombin produced during coagulation increased the frequency and intensity of myometrial contractions, an effect that is suppressed by blood containing thrombin inhibitors (30,31).
Pathologic Uterine Distention
Multiple gestation, polyhydramnios, and other causes of excessive uterine distention are risk factors for PTB. Enhanced stretching of the myometrium induces the formation of gap junctions, upregulation of oxytocin receptors, and production of prostaglandins and myosin light chain kinase. All of these are critical events that precede uterine contractions and cervical dilation (32,33).
Risk Factors
Three types of factors may contribute to spontaneous PTB: Social stress and race, infection and inflammation, and genetics.
Social Stress and Race
Poverty, limited maternal education, young maternal age, unmarried status, and inadequate prenatal care are clearly associated with increased risk of PTB and LBW. Another consistent factor that increases risk is maternal race. In the United States, the rate of PTB among black women is twice as high as the rate among white women (Fig. 19-2); furthermore, the rate of recurrent PTB in black women is 4 times as high as the rate among white women.
Infection and Inflammation
Chorioamnionitis is the most obvious example of infection associated with early labor and delivery. Approximately 25% of PTBs are caused by infection, with bacterial colonization ranging from 11% to 79% (34,35). In most cases of preterm labor and delivery, intrauterine infection is not clinically apparent (17). However, histologic evidence of inflammation in the decidua, fetal membranes, or umbilical cord is relatively common.
Genetic Factors
Maternal history of PTB is a strong risk factor for PTB (36). PTB might be considered a common complex disorder that involves gene–gene and gene–environment interactions. Maternal genes, or maternal genes acting in the fetus, largely determine the risk of PTB with a smaller role for paternal genes (37). Common environmental factors influence racial differences in pregnancy outcomes. These influences may overwhelm the effect of what might seem to be small genetic differences. A recent large review of retrospective data (38) as shown that the rate of deaths for infants born during the entire term period has fallen in the past decade. However, the analysis revealed large differences in the decline in the infant death rate between racial and ethnic groups during the early-term period. For example, over the 11-year period of the analysis, the infant death rate for infants born at 37 weeks fell by 35 % and 22 % for Hispanics and non-Hispanic whites respectively, and by 6.8 % for non-Hispanic African-American infants. The rate of deaths within the first month of life for non-Hispanic black infants born at 37 weeks did not decline at all, and in 2006 was the same as the neonatal mortality rate in 1995 for Hispanic and non-Hispanic infants born at 37 weeks.
Diagnosis
The diagnosis of preterm labor is clinical. Cervical change or cervical effacement of at least 80%, or cervical dilatation greater than 2 cm together with four regular painful contractions every 20 minutes or eight every 60 minutes commonly establish the diagnosis of preterm labor in a patient at 37 weeks or less of gestational age (39).
The next diagnostic steps commonly performed in women with suspected or diagnosed preterm labor aim at determining whether there is uterine bleeding (possible placenta previa or placental abruption), whether the fetal membranes have ruptured (possible PPROM), and whether there is concomitant chorioamnionitis. In addition, an accurate gestational age and fetal well-being are estimated by ultrasonography and cardiotocography.
In many women, uterine contractions cease spontaneously. In fact, only 20% of women evaluated for preterm labor have preterm delivery (40).
Therapy for Women in Preterm Labor
Acute tocolysis and antenatal corticosteroids are the main therapeutic strategies for women in preterm labor (41,42). Except in rare circumstances, there is no way to arrest preterm labor. Physicians can only delay birth by 1 to 7 days with the current therapeutic options available (41). Once the diagnosis has been established, the obstetrician must decide whether to start a short course of tocolytic therapy that aims at delaying the delivery for at least 48 hours (Table 19-1). This time frame is extremely important to allow maternal administration of corticosteroids to accelerate fetal lung maturity (42) and administration of antibiotic therapy to prevent neonatal group B streptococcal infection.
Acute Tocolysis
Overview of Tocolytic Therapy
Tocolytic therapy for an acute episode of preterm labor often abolishes contractions temporarily, but it does not remove the underlying cause that initiated the process of parturition (Table 19-2). The most important goal of tocolysis is to delay delivery by at least 48 hours so that
corticosteroids given to the mother can reach their maximum effect. An additional goal of tocolysis is to allow for more time to transport the mother, if needed, to a hospital that can provide appropriate neonatal care in case she delivers preterm. In addition, tocolytic therapy is beneficial in self-limited conditions that might increase the risk of preterm labor such as pyelonephritis and abdominal surgery (43). The underlying etiology of preterm labor, rather than gestational age, determines when to start acute tocolysis. In fact, there are no definitive data from randomized trials on which to base a recommendation for the lowest gestational age at which inhibition of preterm labor should be considered (44). Many experts choose a gestational age of 15 weeks since early pregnancy loss at that age is less commonly attributable to karyotypic abnormality. Others choose 20 weeks because delivery before this age is considered a spontaneous abortion rather than a PTB. However, inhibiting contractions after intra-abdominal surgery, a self-limited event that can cause preterm labor, may be a reasonable choice regardless of the gestational age (45,46). Inhibition of labor is not attempted after 34 weeks of gestation since this age represents the upper threshold at which perinatal morbidity and mortality are adequately reduced to justify the potential maternal and fetal complications associated with tocolytic therapy (44,47,48,49).
corticosteroids given to the mother can reach their maximum effect. An additional goal of tocolysis is to allow for more time to transport the mother, if needed, to a hospital that can provide appropriate neonatal care in case she delivers preterm. In addition, tocolytic therapy is beneficial in self-limited conditions that might increase the risk of preterm labor such as pyelonephritis and abdominal surgery (43). The underlying etiology of preterm labor, rather than gestational age, determines when to start acute tocolysis. In fact, there are no definitive data from randomized trials on which to base a recommendation for the lowest gestational age at which inhibition of preterm labor should be considered (44). Many experts choose a gestational age of 15 weeks since early pregnancy loss at that age is less commonly attributable to karyotypic abnormality. Others choose 20 weeks because delivery before this age is considered a spontaneous abortion rather than a PTB. However, inhibiting contractions after intra-abdominal surgery, a self-limited event that can cause preterm labor, may be a reasonable choice regardless of the gestational age (45,46). Inhibition of labor is not attempted after 34 weeks of gestation since this age represents the upper threshold at which perinatal morbidity and mortality are adequately reduced to justify the potential maternal and fetal complications associated with tocolytic therapy (44,47,48,49).
Table 19-1 Indications and Contraindications for Inhibition of Preterm Labor | |
---|---|
|
Table 19-2 Tocolytic Management of Acute Preterm Labor | ||||||||||||||||||||||||||||||||||||
---|---|---|---|---|---|---|---|---|---|---|---|---|---|---|---|---|---|---|---|---|---|---|---|---|---|---|---|---|---|---|---|---|---|---|---|---|
|
All of the commonly used tocolytic agents are effective for delaying delivery from 48 hours to 7 days (41). However, prolongation
of pregnancy does not significantly reduce overall rates of respiratory distress syndrome or neonatal death.
of pregnancy does not significantly reduce overall rates of respiratory distress syndrome or neonatal death.
Obstetricians use one pharmacologic agent at a time since the concomitant use of different tocolytics offers no additional benefits and adds only side effects (50,51). If the single-agent therapy is not effective, physicians switch to a different agent after ruling out chorioamnionitis. Sixty-five percent of women in whom tocolysis with a single-agent is not successful have positive amniotic fluid cultures (52). In fact, in the absence of clear clinical criteria for infection, some obstetricians might consider amniocentesis to help exclude occult intra-amniotic infection before switching to a second pharmacologic agent.
Contraindications to acute tocolysis include chorioamnionitis, non-reassuring fetal status, severe fetal growth restriction, severe preeclampsia, maternal hemorrhage with hemodynamic instability, lethal fetal anomaly, and intrauterine fetal demise (Table 19-1).
There are two particular situations wherein anesthesia providers may be requested to administer neuraxial analgesia and anesthesia or general anesthesia to preterm labor patients who are receiving tocolytic therapy. The first situation is when tocolysis fails and patients desire labor analgesia or require anesthesia because of a cesarean delivery. The second is when tocolysis is administered before or during the performance of a trans-abdominal or cervical cerclage. Anesthetic management may be easily facilitated when anesthesia providers are familiar with tocolytic pharmacology, side effects, and interaction with anesthetic agents.
Tocolytic Agents
Magnesium Sulfate
Magnesium competes with calcium at the level of the plasma membrane voltage-gated channels. It hyperpolarizes the plasma membrane and inhibits myosin light-chain kinase activity reducing myometrial contractility (53,54,55). Even though its efficacy is questionable (56), magnesium sulfate is still one of the most common drugs used for the treatment of acute tocolysis in North America. Similarly to β-adrenergic agonists, magnesium sulfate can cause pulmonary edema and chest pain (57). Maternal therapy causes a slight decrease in baseline fetal heart rate and fetal heart rate variability, both of which are not clinically significant (58). Biophysical profile score and non-stress test reactivity are not significantly altered.
Anesthetic Implications of Magnesium Therapy
There are no contraindications to perform a neuraxial block or to administer general anesthesia in a parturient receiving magnesium sulfate. When neuraxial analgesia or anesthesia is given, magnesium decreases maternal blood pressure but not uterine blood flow (UBF) (59). Ephedrine (60) and phenylephrine can be administered to prevent and treat maternal hypotension (61). Even though magnesium sulfate potentiates the neuromuscular blockade induced by non-depolarizing neuromuscular blockers (62,63) and might antagonize the block produced by succinylcholine (64), there is no evidence that the intubating dose of any muscle relaxant should be modified for induction of general anesthesia in a preterm laboring patient.
However, in patients receiving magnesium, the maintenance dose of non-depolarizing neuromuscular blocker must be reduced and carefully titrated with a nerve stimulator to ensure adequate recovery of neuromuscular function at the end of the cesarean delivery. In addition, neostigmine-induced recovery is attenuated in patients treated with magnesium (63). Current evidence suggests that postoperative analgesic needs are not decreased in patients who receive intravenous magnesium (65). The reader is referred to Chapter 28 for more extensive information on magnesium sulfate dosing regimens, monitoring, toxicity, and interactions with anesthetic drugs. The neuroprotective effects of in utero exposure to magnesium sulfate are addressed in Chapter 17.
β-adrenergic Receptor Agonists
In the United States, terbutaline is the most commonly used β-adrenergic receptor agonist for acute tocolysis. Ritodrine and terbutaline have been studied in several randomized, placebo-controlled trials but ritodrine is no longer available in the United States. Terbutaline can be administered intravenously, subcutaneously, or orally. The desired uterine relaxation following terbutaline is a result of β2 receptor activity in the uterine smooth muscle. The concomitant stimulation of β1 receptors results in undesirable maternal and fetal side effects (Table 19-2).
A Cochrane review involving 1,332 patients found that β-adrenergic receptor agonists decreased the number of women giving birth within 48 hours (RR 0.63, 95% CI 0.53–0.75) and possibly within 7 days (RR 0.67, 95% CI 0.48–1.01) (66). There was also a trend toward reduction in respiratory distress syndrome that was not statistically significant. The agonists had no effect on the neonatal death rate.
Although both β1– and β2-adrenergic receptors are present in human myometrial tissue at term, uterine relaxation is mediated exclusively by β2-adrenergic receptors (67). Terbutaline is 60 times more selective for the β2– than for the β1-adrenergic receptors (68). It activates the G protein, GS, which then stimulates intracellular adenylate cyclase. Adenylate cyclase enhances the hydrolysis of adenosine triphosphate (ATP) to cyclic adenosine monophosphate (cAMP) and activates protein kinase, resulting in the phosphorylation of intracellular proteins. This causes a drop in intracellular free calcium resulting in inhibition of the interaction between actin and myosin with the consequential myometrial relaxation.
Recently, a β3-adrenergic receptor has been found to be the predominant β-adrenergic receptor subtype in the pregnant and non-pregnant human myometrium (69). Its role in the treatment and prevention of preterm labor is currently under investigation (70).
At the author’s institution, terbutaline is given subcutaneously by intermittent injection. Usually, 0.25 mg is administered every 20 to 30 minutes up to 4 doses or until tocolysis is achieved. Once labor is inhibited, 0.25 mg can be administered every 3 to 4 hours until the uterus is quiescent. If continuous intravenous infusion is used, terbutaline is usually started at 2.5 to 5 μg/min. This rate can be increased by 2.5 to 5 μg/min every 20 to 30 minutes to a maximum of 30 μg/min, or until the contractions have abated. Once this goal is reached, the infusion can be reduced by decrements of 2.5 to 5 μg/min to the lowest dose that maintains uterine quiescence.
Terbutaline administration can cause significant adverse maternal and fetal side effects in the cardiovascular and central nervous systems as well as in metabolism (Table 19-2). During terbutaline administration, the clinician should monitor fluid intake, urine output, and recognize the occurrence of maternal symptoms, especially shortness of breath, chest pain, and tachycardia. In addition, glucose and potassium concentrations should be monitored every 4 to 6 hours since hyperglycemia and hypokalemia commonly occur. Significant hypokalemia should be treated to minimize risk of arrhythmias, and significant hyperglycemia should be treated with insulin.
Cardiovascular Side Effects
Cardiovascular effects are produced directly by the binding of terbutaline on β2-adrenergic receptors on adrenergic nerve
terminals and indirectly by enhancing norepinephrine release after activation of presynaptic β2-receptors (71). Increases in heart rate and inotropism induced by terbutaline are mediated almost exclusively by cardiac β2-adrenergic receptor stimulation (72).
terminals and indirectly by enhancing norepinephrine release after activation of presynaptic β2-receptors (71). Increases in heart rate and inotropism induced by terbutaline are mediated almost exclusively by cardiac β2-adrenergic receptor stimulation (72).
In a study by Jartti et al., a continuous 3-hour intravenous infusion of 10 to 30 μg/min of terbutaline increased the heart rate (from 57 to 109 beats/min) and the minute ventilation (11 to 13 L/min) in healthy volunteers in a dose dependent fashion. In the same experiment, the plasma potassium concentration decreased (from 4 to 2.5 mEq/L) and blood pressure did not change significantly (from 115/65 to 120/64 mm Hg) (73). The cardiovascular response to terbutaline can vary significantly and this phenomenon might be caused by structural differences in the β2-receptor. β2-adrenergic receptors are polymorphic, and there are four major coding sequence polymorphisms: Arg19Cys, Arg16Gly, Gln27Glu, and Thr164Ile (74). Some investigators have found that the β2-adrenergic receptor polymorphism significantly affects the dose–response curves for terbutaline-induced inotropic and chronotropic responses. Subjects with the Thr164Ile receptor show substantial blunting in maximal increase in heart rate and a shortening of the electromechanical systole (75).
It is speculated that the concomitant marked hypokalemia that occurs during terbutaline therapy most likely contributes to the tachycardia by increasing the slope and amplitude of action potentials in the sinoatrial and atrioventricular nodes (73). Similar to cardiac responses, vasodilator responses to β2-adrenergic stimulation are blunted in subjects carrying one allele of the Ile 164 β2-adrenergic receptor when compared with subjects homozygous for the Thr164 β2-adrenergic receptor (76,77). Therefore, stimulation of the β2-adrenergic receptors on blood vessels can result in various degrees of diastolic hypotension (75,78).
Common cardiovascular symptoms associated with the use of β2-adrenergic agonists include palpitations (18%), shortness of breath (15%), and chest discomfort (10%) (79). ST-segment depression and T-wave changes can occur and typically resolve once β-adrenergic therapy has been discontinued (80). Myocardial ischemia is also a potential complication of β-adrenergic therapy (80) even though a retrospective study conducted on 8,709 subjects failed to demonstrate any cardiac ischemic event in pregnant patients who received terbutaline therapy (81).
Pulmonary edema has been reported in 0.3% (3/1,000) of patients receiving β-mimetic therapy (81). A study reviewing 62,917 consecutive pregnancies showed pulmonary edema attributable to tocolytic use in 13 patients. All of these patients (whose pulmonary edema was secondary to tocolytic use) received multiple simultaneous tocolytic agents. The most common combination was intravenous magnesium sulfate used in conjunction with subcutaneous terbutaline (82). Multiple gestations, maternal infection, and multiple tocolytic agents seem to be the main risk factors for the development of pulmonary edema (82,83).
Although normal pulmonary capillary wedge pressures have been found in parturients who showed pulmonary edema during β-adrenergic therapy (84), there are two possible factors that play a role in the pathogenesis of tocolytic-induced pulmonary edema: Fluid overload (85) and increased pulmonary capillary permeability (84).
Fluid overload during β-adrenergic therapy is probably the major pathogenic factor during terbutaline tocolysis. It may occur as a combination of fluid and sodium retention secondary to increase in renin and anti-diuretic hormone activity from β-adrenergic receptor stimulation (85).
Some investigators have observed that when β-adrenergic agents are administered, the risk for development of pulmonary edema changes according to which crystalloid solution is used. Isotonic sodium chloride causes more fluid retention than dextrose-containing solutions (86). In pregnant baboons, ritodrine and lactated Ringer’s have been associated with more fluid and sodium retention than lactated Ringer’s alone (87). In one setting, isotonic solutions cause more sodium and fluid retention; in another setting, dextrose-containing solutions increase the likelihood of hyperglycemia and hypokalemia. Therefore, it seems reasonable to administer the β-adrenergic agent with a hypotonic solution of 0.45% sodium chloride; in addition, it is crucial to carefully monitor the patient’s total fluid daily intake.
The administration of β-adrenergic tocolysis in a parturient with preterm labor and infection may increase the risk of developing non-cardiogenic pulmonary edema, probably because of increased pulmonary vascular permeability through the release of endotoxin (88,89).
Pulmonary edema usually develops within 24 to 72 hours after the start of β-adrenergic therapy (90,91) and the clinical presentation is nearly identical to that of other types of pulmonary edema: Dyspnea, tachypnea, tachycardia, hypoxemia, and diffuse crackles. Chest pain and a cough may also be present. A review of 58 case reports and case series found that the following were the most frequent occurrences in tocolytic-related pulmonary edema: Basilar crackles (100%), shortness of breath (76%), chest pain (24%), cough (17%), bilateral air space disease on chest radiograph (81%), and fever (14%) (84). Tocolytic-related pulmonary edema is a diagnosis of exclusion. Mortality is uncommon and treatment is supportive with most patients responding well to discontinuation of the β2-adrenergic agonist, supplemental oxygen, fluid restriction, and diuresis. Even though mechanical ventilation may be necessary, most cases resolve within 12 to 24 hours. Persistence of symptoms beyond this time period should prompt reconsideration of the diagnosis.
Metabolic Side Effects
β-adrenergic receptor agonist administration is associated with two major metabolic effects: Hyperglycemia (30%) and hypokalemia (39%) (79,92) (Fig. 19-3). By stimulating adenyl cyclase on the membrane of liver cells, β-adrenergic agonists activate hepatic phosphorylase which increases the breakdown of glycogen and results in subsequent production of glucose and development of hyperglycemia. Insulin-dependent diabetics who receive these agents may require more frequent assessment of blood glucose levels and eventually a continuous insulin infusion in order to prevent the development of ketoacidosis (93).
Hypokalemia occurs as a result of the insulin-mediated movement of potassium, together with glucose, from the extracellular to the intracellular space, and to direct stimulation of β2-receptors in skeletal muscle leading to activation of Na+–K+ ATPase (94). Total body potassium remains unchanged since urinary excretion of potassium is not increased during β-adrenergic therapy. Since serum potassium concentration returns to normal within 3 hours of discontinuing β-adrenergic therapy (95,96), intravenous potassium infusion is usually not needed (93,96,97). In addition, a rebound hyperkalemia might follow potassium infusion in patients undergoing general anesthesia regardless of the use of depolarizing or non-depolarizing muscle relaxants (98,99,100).
Miscellaneous Maternal Systemic Side Effects
The main central nervous system symptom associated with use of β2-adrenergic agonists is tremor (79). Cerebral ischemia due to vasospasm has been reported in a patient who had a previous history of migraine (101). There have been four reported cases of terbutaline-associated hepatitis in
pregnancy (102). In each case, discontinuation of terbutaline led to the amelioration of enzymatic markers of liver damage. Muscle weakness and respiratory arrest have been reported in a parturient affected by myasthenia gravis (103).
pregnancy (102). In each case, discontinuation of terbutaline led to the amelioration of enzymatic markers of liver damage. Muscle weakness and respiratory arrest have been reported in a parturient affected by myasthenia gravis (103).
Fetal Side Effects
β-adrenergic receptor agonists promptly cross the placenta into the fetal compartment causing fetal tachycardia. Neonatal hypoglycemia may result from fetal hyperinsulinemia due to prolonged maternal hyperglycemia.
Contraindications
Labor inhibition with β-adrenergic receptor agonists should be used with caution in women with cardiac disease and in women with poorly controlled hyperthyroidism or diabetes mellitus. In women at risk for massive hemorrhage, β-adrenergic receptor agonists may cause maternal tachycardia and hypotension. The presence of these clinical signs may interfere with the mother’s ability to mount a compensatory response to ongoing hemorrhage as well as confuse the clinical presentation.
The final decision of whether or not to administer these agents in these situations requires balancing the perceived benefit of delaying the delivery with potential side effects of treatment.
Anesthetic Implications of β-adrenergic Therapy
Based on published reports of obstetric anesthetic management after administration of β-adrenergic agonists (104,105,106), there is not enough evidence to suggest delaying neuraxial or general anesthesia when the fetus or the mother is in danger. The main concerns about giving anesthetics during the β-adrenergic therapy involve the tachycardic and hypotensive side effects of terbutaline that might exacerbate the maternal hypotension associated with the use of spinal or general anesthetics. Terbutaline’s hemodynamic effects subside within 15 to 30 minutes after the drug is discontinued. The use of phenylephrine, an α1-adrenergic agonist, counteracts the decrease in systemic vascular resistance associated with terbutaline and may cause a reflex bradycardic effect, which would be beneficial when maternal tachycardia follows terbutaline administration. Chestnut et al. (107) observed that prior administration of ritodrine did not worsen maternal hypotension when epidural lidocaine without epinephrine was administered to gravid ewes (Fig. 19-4). The inotropic and chronotropic effects of ritodrine seemed to preserve cardiac output and Please modify to UBF(Uterine Blood Flow) in that study. It is advisable to avoid aggressive fluid administration prior to or during the induction of regional or general anesthesia in patients who have received β-adrenergic agonists. If hypotension occurs, titration of vasopressors rather than fluids seem the best therapeutic option given these patients’ increased propensity to develop pulmonary edema. In gravid animals rendered hypotensive by acute hemorrhage during terbutaline infusion, ephedrine aids restoration of UBF velocity (108) (Fig. 19-5). Some investigators evaluated the prophylactic administration of various vasopressors to normotensive gravid animals pretreated with a ritodrine infusion (109); they have found that while phenylephrine and epinephrine worsened UBF velocity, ephedrine preserved it (Fig. 19-6). However, the doses used to appreciate such a difference (phenylephrine 10 μg/kg and ephedrine 1 mg/kg) in that experiment were nearly 10 times higher than those used for humans in the current
clinical practice. A subsequent study compared the maternal and fetal effects of maternally administered ephedrine to phenylephrine in gravid animals subjected to epidural anesthesia-induced hypotension during ritodrine infusion (110). Ephedrine increased uteroplacental blood flow without changing uterine vascular resistance while phenylephrine administration did not change uteroplacental blood flow but increased uterine vascular resistance (Fig. 19-7). One should be careful in extrapolating laboratory data to clinical practice. To date, the most reasonable approach is to defer to the anesthesia provider’s clinical decision as to whether ephedrine or phenylephrine should be used for the treatment and prevention
of hypotension during regional or general anesthesia in preterm laboring patients who receive β-adrenergic agonists.
clinical practice. A subsequent study compared the maternal and fetal effects of maternally administered ephedrine to phenylephrine in gravid animals subjected to epidural anesthesia-induced hypotension during ritodrine infusion (110). Ephedrine increased uteroplacental blood flow without changing uterine vascular resistance while phenylephrine administration did not change uteroplacental blood flow but increased uterine vascular resistance (Fig. 19-7). One should be careful in extrapolating laboratory data to clinical practice. To date, the most reasonable approach is to defer to the anesthesia provider’s clinical decision as to whether ephedrine or phenylephrine should be used for the treatment and prevention
of hypotension during regional or general anesthesia in preterm laboring patients who receive β-adrenergic agonists.
If general anesthesia is required after discontinuation of a β-adrenergic agonist, one should remember that the underlying tachycardia that results from the β-adrenergic therapy makes it difficult to estimate the depth of anesthesia and fluid status. It is useful to monitor anesthesia depth by any of the devices currently available and to communicate closely with the obstetrics team for the estimation of maternal blood loss. Cardiac arrhythmias are associated with the use of β-adrenergic agonists. Shin and Kim (104) reported one case in which ventricular tachycardia and fibrillation developed after administration of ephedrine for treatment of hypotension (during epidural anesthesia for cesarean delivery) 30 minutes after the discontinuation of a ritodrine infusion. When general anesthesia is required, inhalational anesthetics that do not sensitize the myocardium to catecholamine-induced ventricular dysrhythmias (such as sevoflurane or isoflurane) seem to be the anesthetics of choice. Hyperventilation should be avoided as it may increase uterine vascular resistance and induce respiratory alkalosis. Respiratory alkalosis may contribute to the shift of potassium into the intracellular compartment, exacerbating the hypokalemia induced by β-agonist therapy.
Calcium Channel Blockers
Calcium channel blockers directly block the influx of calcium ions through the cell membrane. They also inhibit the release of intracellular calcium from the sarcoplasmic reticulum and increase calcium efflux from the cell. The ensuing decrease in intracellular free calcium leads to an inhibition of calcium-dependent myosin light-chain kinase phosphorylation and results in myometrial relaxation.
There are no large randomized trials directly examining the efficacy of calcium channel blockers compared with a placebo for treatment of preterm labor.
A meta-analysis of 12 randomized controlled trials involving over 1,000 women compared use of calcium channel blockers to any other tocolytic agent (mainly β-adrenergic receptor agonists) (111). Compared with other tocolytics, calcium channel blockers did not significantly reduce the risk of birth within 48 hours of initiation of treatment, but they did reduce the risk of birth within 7 days. On the contrary, the nine trials comparing use of any calcium channel blockers to any β-adrenergic receptor agonist found that calcium channel blockers significantly decreased the risk of preterm labor within 48 hours of initiating treatment (RR 0.72, 95% CI 0.53–0.97) (111).
Calcium channel blockers also reduce the risk of respiratory distress syndrome, necrotizing enterocolitis (NEC), intraventricular hemorrhage (IVH), and neonatal jaundice. Similar findings were reported from a meta-analysis of nine randomized trials (679 patients) comparing treatment of preterm labor with nifedipine versus terbutaline or ritodrine (112). Nifedipine was more effective than β-adrenergic receptor agonists in delaying delivery for at least 48 hours (OR 1.52, 95% CI 1.03–2.24). A randomized trial comparing nifedipine
with magnesium sulfate did not find a significant difference in the rate of delivery within 48 hours (113). A common dosing regimen is to administer an initial loading dose of nifedipine 20 mg orally, followed by another 20 mg orally 90 minutes later. An alternative regimen is to administer 10 mg orally every 20 minutes for up to 4 doses. If contractions persist, 20 mg can be given orally every 3 to 8 hours for up to 72 hours not to exceed a maximum dose of 180 mg/day (Table 19-2).
with magnesium sulfate did not find a significant difference in the rate of delivery within 48 hours (113). A common dosing regimen is to administer an initial loading dose of nifedipine 20 mg orally, followed by another 20 mg orally 90 minutes later. An alternative regimen is to administer 10 mg orally every 20 minutes for up to 4 doses. If contractions persist, 20 mg can be given orally every 3 to 8 hours for up to 72 hours not to exceed a maximum dose of 180 mg/day (Table 19-2).
A single orally administered dose of nifedipine acts for up to 6 hours. Plasma concentrations peak in 30 to 60 minutes. Nifedipine is almost completely metabolized in the liver and excreted by the kidney.
Maternal and Fetal Side Effects
Nifedipine is a peripheral vasodilator and may cause nausea, flushing, headache, vertigo, and palpitations. Nifedipine causes peripheral vasodilation resulting in a decrease in mean arterial pressure with the subsequent activation of baroreceptors, leading to increased peripheral sympathetic nervous system activity that manifest with maternal tachycardia. These hemodynamic changes are usually mild and less severe than those seen after β-adrenergic agonist treatment (114,115,116). However, severe hypotension has been described in case reports (117,118) and has been reported in a preeclamptic patient while on a magnesium sulfate infusion (119). There are no data regarding fetal side effects related to oral dosing with the doses commonly used for labor inhibition. Animal studies showed a decrease in UBF and decreased fetal oxygen saturation with the administration of calcium channel blockers; however, in humans, Doppler studies of fetal umbilical and uteroplacental blood flow have been reassuring (120). The fetal acid–base status in the umbilical cord at delivery has not shown any fetal hypoxia or acidosis.
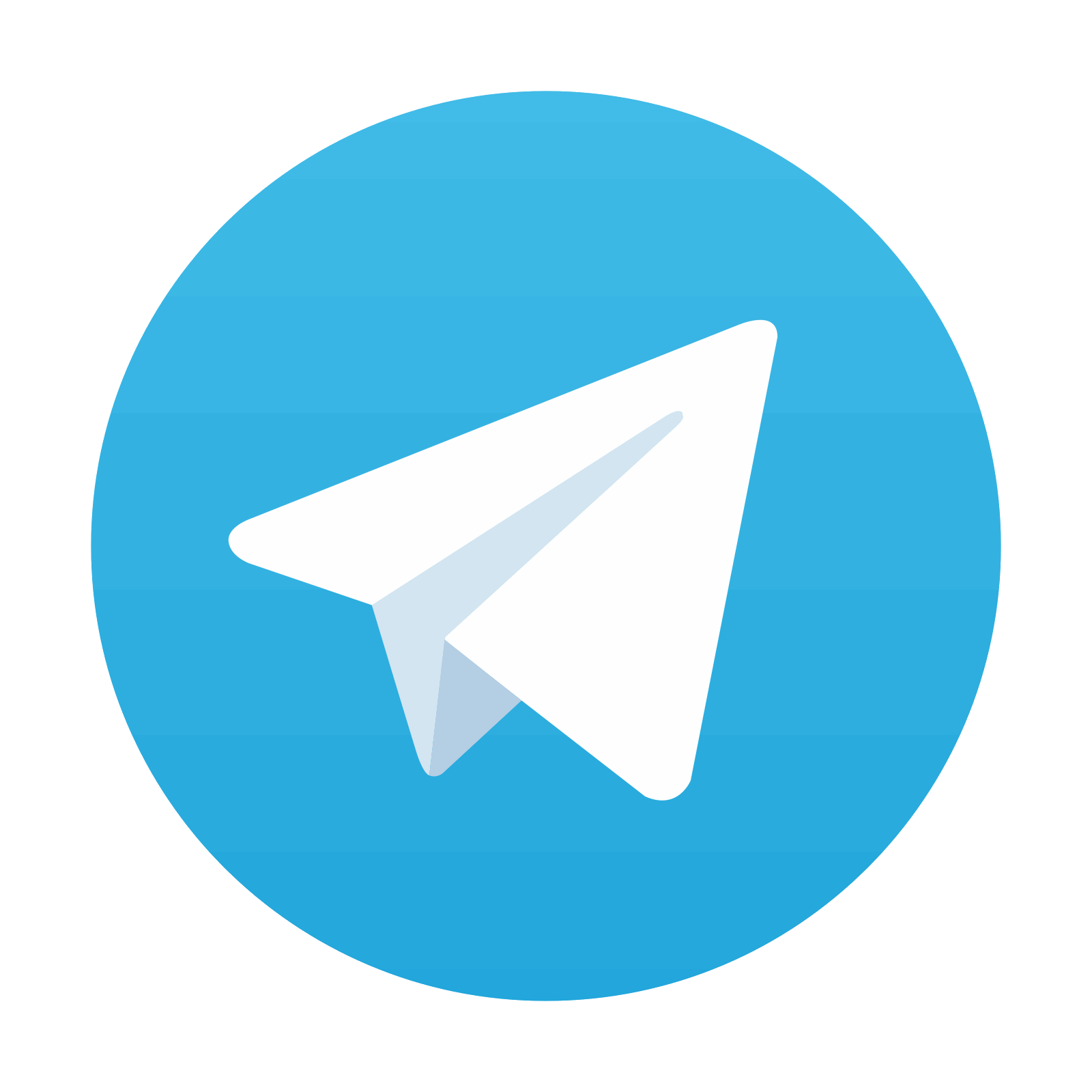
Stay updated, free articles. Join our Telegram channel

Full access? Get Clinical Tree
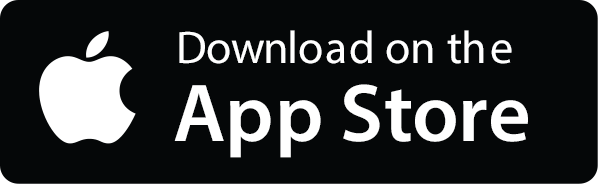
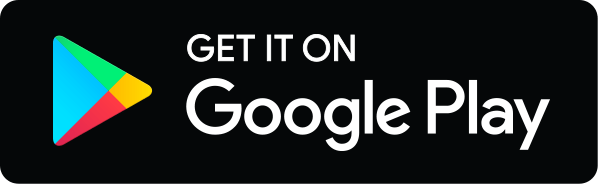
