Case: A 60-year-old female with carcinoma of the colon is scheduled for laparoscopic colon resection. She has hypertension, obstructive sleep apnea (OSA), and is morbidly obese with a body mass index (BMI) of 41 kg·m−2. She provides a history of difficult airway management at another institution 10 years previously at cholecystectomy. Records are not available. Examination of her airway reveals mouth opening of 3 cm (approximately 2 fingerbreadths), full dentition with prominent upper incisors, and a receding mandible. Mandibular mobility is poor and she is unable to bite her upper lip with her lower incisors. Her neck is short and has a circumference of 44 cm. Her cricothyroid membrane (CTM) is difficult to identify. Her neck extension is unremarkable and she has a Mallampati score of IV.
This patient has predictors of difficult facemask ventilation, difficult direct laryngoscopy, difficult video-laryngoscopy (VL), difficult supraglottic device, and difficult surgical airway. An awake flexible bronchoscopic intubation is an appropriate airway management plan. Alternative techniques include awake intubation using a video-laryngoscope or an optical stylet.
Awake bronchoscopic intubation can be performed rapidly with minimal patient discomfort. The technique requires familiarity with the airway anatomy, adequate regional anesthesia of the airway, and bronchoscopic dexterity. Optimal regional anesthesia of the airway requires a thorough knowledge of the pharmacology of the local anesthetics employed and the techniques of administration. The primary requirement for successful awake intubation is effective regional anesthesia of the airway.1
Knowledge of the structure, function, and pathophysiology of the upper airway permits the practitioner to anticipate potential life-threatening problems and better utilize the full spectrum of airway management techniques.2 Functionally, the upper airway can be considered to consist of the nasal cavities, pharynx, larynx, and trachea (see Figure 3–1).3 The oral cavity provides an alternate access route to the pharynx.
Anatomically, the nose can be divided into an external component and the nasal cavity.4 The external nose consists of a bony vault posteriorly and superiorly, a cartilaginous vault anteriorly, and the lobule at its inferior-anterior aspect (see Figure 3–2).3 The cavity of the nose is divided into bilateral compartments by the nasal septum and continues about 3 inches (7.6 cm)5 posteriorly from the nostrils (nares), to communicate with the nasopharynx at the posterior aspect of the septum (the choanae) (see Figure 3–3).3 The nasal lobule includes the tip of the nose, the U-shaped lower lateral cartilages, the fibrofatty alae, the vestibule, and the columella.6 The vestibule is the small dilatation located immediately inside the nostrils,3,4 and the columella is the soft tissue anterior termination of the septum. Each nasal cavity is bounded by a floor, a roof, and medial and lateral walls.3,4,7 The roof of the nasal cavity extends posteriorly from the bridge of the nose, and consists of the lateral nasal cartilages, the nasal bones and spine of the frontal bone, the cribriform plate of the ethmoid, and the inferior aspect of the sphenoid (see Figure 3–2).3–5 The medial wall is the nasal septum and is formed by the quadrilateral cartilage, the perpendicular plate of the ethmoid, and the vomer (see Figure 3–3).3 The posterior border of the vomer is free and forms the medial aspect of the choanae. The lateral wall of the nasal cavity is formed anterior-inferiorly by nasal cartilages and the frontal process of the maxilla, anterior-superiorly by the nasal bones, superiorly by the nasal aspect of the ethmoid, and posteriorly by the perpendicular plate of the palatine and medial pterygoid plate.3,8 A series of three horizontal scroll-like ridges (conchae or turbinates) project medially from the lateral walls of the nasal cavities, each of which overhangs a corresponding groove or meatus (see Figures 3–2, 3–4, and 3–5).3,9 Septal deviation is common, may be associated with compensatory hypertrophy of the turbinates, and can produce nasal obstruction (see Figure 3–4).3,4 The paranasal sinuses and the nasolacrimal duct empty into the nasal cavity through ostia in the lateral wall.3 Obstruction of the ostia of the paranasal sinuses can occur with prolonged nasal intubation and can cause sinusitis.2,3 The floor of each nasal cavity is concave and is formed by the palatine process of the maxilla and the horizontal plate of the palatine bone.3,4 The floor extends posteriorly in a transverse plane from the vestibule.
The major nasal airway is located below the inferior turbinate and declines slightly front to back (approximately 20 degrees). For this reason a nasotracheal tube or flexible endoscope should be directed backward along the floor of the nose and not upward.2–4 Occasionally, the posterior aspect of the inferior turbinate may be hypertrophied and resistance to the passage of a nasotracheal tube may be encountered at this location.4 Alternating counterclockwise/clockwise rotation of the tube changes the orientation of the bevel and may facilitate negotiation of the nasal cavity (see Chapter 12).
The anterior and posterior ethmoidal branches of the internal carotid artery supply the anterior-superior aspect of the nasal cavity and the sphenopalatine branch of the external carotid supplies the posterior-inferior aspect.3,6 The sphenopalatine is the usual source of posterior epistaxis.10 The vestibule receives blood supply from both the anterior ethmoidal and sphenopalatine arteries, as well as from nasal branches of the superior labial branch of the facial artery (see Figures 3–6A and B).3,6 The anastomotic site at the anterior-inferior aspect of the septum (Little’s area or Kiesselbach’s plexus) is a common site of anterior epistaxis.3,4,6,10 Tintinalli and Claffey11 reported moderate to severe epistaxis in 7% of 71 attempted emergency nasotracheal intubations. The single case of severe epistaxis in this series occurred in a patient with cirrhosis. Minimal epistaxis has been reported in 11% to 40% of nasal intubations.11,12 In a series of 99 patients undergoing nasotracheal intubation for oromaxillofacial surgery, epistaxis occurred in 6 patients but was sufficient to result in a visible accumulation of blood in the pharynx in only 1 patient.13 Of 175 anesthetists who underwent nasotracheal intubation at a training course, minor nasal bleeding was seen in 20 during endoscopy or after extubation.14 None of the 20 subjects required suction to control bleeding or clear the airway, and the bleeding did not interfere with endoscopy. During nasal intubation passing the tube with the bevel facing the septum directs the leading edge away from the vascular septum. However, the optimum orientation of the tube is controversial (see section “Blind Nasal Intubation” in Chapter 12).15 Perforation into the submucosal space can occur and lead to hematoma and abscess formation.2,3 Excessive force must be avoided.3
Common sensation to the nasal cavities is supplied by the ophthalmic and maxillary divisions of the trigeminal nerve.2,3 The posterior aspect of the septum is innervated by the short and long sphenopalatine branches of the maxillary nerve.3,4 Anteriorly the septum and the lateral wall is supplied by the anterior ethmoidal branch of the ophthalmic nerve (see Figures 3–7A and B).8 The posterior-superior aspect of the lateral wall is innervated by the short sphenopalatine nerve and the inferior aspect by the posterolateral nasal branches of the sphenopalatine nerve.3,4,6 Anteriorly, the floor of the nose is supplied by the anterior-superior dental branch of the infraorbital nerve and posteriorly by the greater palatine.3,4 Rootlets of the olfactory nerve located in the roof of the nose adjacent to the cribriform plate transmit the sense of smell.3
In addition to being a respiratory pathway, the nose humidifies and warms inspired air, houses the olfactory receptors, removes bacteria, dust, and other particles from inspired air, and acts as a voice resonator.3,4,9
Anatomically, the mouth consists of (1) the vestibule which is bounded externally by the lips and cheeks and internally by the gums and teeth, and (2) the mouth cavity.3,4 The mouth cavity is bounded by the alveolar arches and the teeth anteriorly and laterally, the hard palate and the anterior aspect of the soft palate above, and the anterior two-thirds of the tongue and the reflection of its mucosa onto the floor of the mouth and mandible below.3,4 Posteriorly, the oral cavity opens into the oropharynx at the oropharyngeal isthmus.3,4,7 The anterior two-thirds of the palate (hard palate) is composed of the palatine plates of the maxillae and the horizontal plates of the palatine bones (see Figure 3–2).3,4,16 Posteriorly the hard palate is continuous with the soft palate, a tough fibrous sheath, which extends to a free posterior border. In the midline, the soft palate ends in the uvula3,4,16 and then curves laterally to blend into the lateral pharyngeal wall at the palatoglossal and palatopharyngeal folds (anterior and posterior tonsillar pillars), respectively.3,4,16 The anterior and inferior aspect of the soft palate faces the mouth cavity and oropharynx, whereas the posterior and superior aspect is part of the nasopharynx.3,4,16 The uvula is a valuable midline landmark during bronchoscopic intubation through the mouth. Movement of the soft palate is controlled by five paired muscles including palatoglossus and palatopharyngeus, which descend in their respective folds to blend with the side of the tongue (palatoglossus) and the side wall of the pharynx (palatopharyngeus) and serve to approximate the folds.3,4 These folds can be used as landmarks for transmucosal glossopharyngeal nerve blocks. The palatine muscles help to isolate the nasopharynx from the mouth during swallowing and phonation.3,4 Paralysis permits regurgitation of food into the nasopharynx and results in nasal speech.3,4 Sensation to the palate is primarily supplied by the trigeminal nerve; however the glossopharyngeal supplies the most posterior aspect (see Figure 3–8).3,4 The anterior two-thirds of the body of the tongue occupies most of the floor of the mouth.2,4,5 The posterior third of the tongue lies in the oropharynx and is separated from the anterior two-thirds by a V-shaped groove on the dorsal aspect of the tongue, the sulcus terminalis.3 The posterior third of the tongue has abundant lymphoid nodules, the lingual tonsil,5 and hypertrophy of this lymphoid tissue can make intubation by direct laryngoscopy difficult or impossible.17 The tongue is also subdivided by a median vertical fibrous septum represented on the dorsum of the tongue by a shallow midline groove,16 another useful landmark during bronchoscopic intubation (see Figure 3–9). The tongue musculature is divided into intrinsic muscles that alter the shape of the tongue,15 and extrinsic muscles that move the tongue as a whole (see Figure 3–10).2,3,16 The extrinsic muscles connect the tongue to the symphysis of the mandible (genioglossus), hyoid (hyoglossus), styloid process (styloglossus), and the soft palate (palatoglossus).16,18 In the supine unconscious individual, a decrease in genioglossal tone allows the tongue to move posteriorly and airway obstruction can occur, although the primary site of obstruction has been shown to be at the level of the soft palate.19–21 Sensation to the anterior two-thirds of the tongue is supplied by the lingual branch of the mandibular nerve,3,16 whereas sensation to the posterior third is supplied by the glossopharyngeal nerve and the superior laryngeal branch of the vagus.5,16 Stimulation of the posterior third of the tongue (as well as other mucosa innervated by the glossopharyngeal nerve) during awake intubation typically provokes the gag reflex and reflex secretions, and can be particularly problematic during bronchoscopic intubation. The tongue receives its blood supply from the lingual branch of the external carotid,16 and is a very vascular structure.5 At the lateral aspect of the tongue, the mucous membrane is reflected onto the floor of the mouth at the “lingual sulcus” and extends laterally to reach the gingiva.3 Deep to the mucous membrane in the floor of the mouth on either side of the tongue anteriorly lie the sublingual glands, and deep to these structures lies the mylohyoid muscle which forms a sling to support the floor of the mouth (see Figure 3–11).3,5,8,16 The submandibular gland straddles the mylohyoid muscle posteriorly.3 Both the lingual and the hypoglossal nerves travel in the floor of the mouth lateral to the tongue in the lingual sulcus.3 Lingual branches of the glossopharyngeal nerve lie deep to the mucosa of the palatoglossal arch (anterior tonsillar pillar) at the lateral aspect of the tongue and can be blocked in this location (see Figure 3–12). The mylohyoid muscle divides the floor of the mouth into two potential spaces: the submandibular space below the muscle and the sublingual space above.2 Both spaces have also been referred to as the submandibular space with the component above the mylohyoid being termed the sublingual space and the component below the mylohyoid the submaxillary space.22 The submaxillary space can be further subdivided into submental, lateral, and posterior divisions.22 Hematoma formation or infection in either of these spaces can displace the tongue superiorly and posteriorly to produce airway compromise and can make intubation difficult (e.g., Ludwig’s angina).
The mandible consists of a horseshoe-shaped body anteriorly and two rami posteriorly, which extend superiorly to end in a condylar head and a coronoid process with an intervening mandibular notch (see Figure 3–13).3 The condylar head articulates with the mandibular fossa of the temporal bone at the temporomandibular joint (TMJ).3 Two types of movement occur at the TMJ—rotation and forward gliding or forward translation—thereby opening the mouth (see Figure 3–14).3,5 Normal mandibular opening in the adult is about 4 cm, or at least 2 finger breadths, between the upper and lower incisors.3,9 Decreased mandibular mobility and anatomic variants, in particular micrognathia, can make intubation under direct laryngoscopy difficult or impossible.
The pharynx is a U-shaped fibromuscular tube which extends from the base of the skull to the lower border of the cricoid cartilage where at the level of the sixth cervical vertebrae in the adult it is continuous with the esophagus (see Figure 3–1).2,3,5 Posteriorly, it rests against the fascia covering the prevertebral muscles. Anteriorly, it communicates with the nasal cavity, mouth, and the larynx at the naso-, oro-, and laryngopharynx, respectively (see Figure 3–15).3,4 From the pharyngeal lumen outward, the wall of the pharynx consists of mucosa, submucosa (pharyngobasillar fascia), muscle, and a loose areolar sheath (the buccopharyngeal fascia).5 This buccopharyngeal fascia is the thin fibrous capsule of the pharynx, contains the plexi of pharyngeal veins and nerves, and is continuous with the areolar sheath of the buccinator muscles and the adventitia of esophagus.2,3,5 Superiorly, it is attached to the base of the skull.2 Edema associated with infection in the floor of the mouth, such as Ludwig’s angina, is limited by the buccopharyngeal fascia, can spread into the pharynx and larynx, and can lead to airway obstruction.3,4 The muscular layer of the pharynx is made up primarily of three paired constrictor muscles that curve around the pharyngeal lumen and telescope into one another (see Figure 3–16). The inferior constrictor consists of an upper oblique part and a lower transverse part (the cricopharyngeus) that is continuous with the esophagus and functions as an upper esophageal sphincter.3,5 The junction of the pharynx with the esophagus is the narrowest part of the gastrointestinal tract and is a common place for foreign bodies to impact.3,5
The nasopharynx extends from the posterior choanae to the tip of the uvula3,6 and forms a backward extension of the nasal cavities (see Figure 3–17).3,5 It is bounded inferiorly by the soft palate.3–5 It communicates with the oropharynx at the pharyngeal isthmus, which is closed during swallowing by the soft palate, the palatopharyngeus, and a ridge of the superior pharyngeal constrictor at the level of the second cervical vertebra, the ridge of Passavant.3–5 The roof of the nasopharynx is formed by the sphenoid bone and curves into the posterior pharyngeal wall at the level of the atlas and axis.3,5,6 The nasopharyngeal tonsils (adenoids) are located in the roof of the nasopharynx and can extend laterally.3,5 The Eustachian tube enters through the lateral wall.3,4 A prominent arch of the atlas vertebra (C1) may protrude anteriorly into the nasopharynx and during nasal intubation, the endotracheal tube can impact the mucosa and resist advancement at this level.23 Rotation of the tube may facilitate passage around this prominence. However on occasion digital manipulation of the tip of the tube by the index and middle fingers inserted through the mouth may be required and this also may not succeed. If these maneuvers fail, or as an alternative, a soft nasal trumpet can be passed first through the nose into the pharynx beyond the anterior tubercle of the atlas. A nasogastric (NG) tube cut off at its proximal end can then be passed into the pharynx through the trumpet and the trumpet removed. A nasal endotracheal tube can then be passed over the NG tube beyond the tubercle of the atlas. The NG tube can then be removed and the endotracheal tube passed into the trachea. The airway caliber in OSA has been shown to be reduced at all levels of the pharynx, with the narrowest portion posterior to the soft palate.19
The oropharynx extends from the soft palate to the tip of the epiglottis3,4,9 and lies behind the mouth cavity and posterior third of the tongue. The palatoglossal folds arch downward from the soft palate to the junction of the anterior two-thirds and posterior third of the tongue and provide the dividing line between the mouth and oropharynx (see Figure 3–9).3,5 This oropharyngeal isthmus is completed by the soft palate and the sulcus terminalis of the tongue.3,5 The palatine tonsils lie on either side of the oropharynx in the triangle formed by the tongue and the palatoglossal and palatopharyngeal arches.3–5 These two palatine arches and the triangular area between them are called the fauces.5 A fold of mucus membrane, the median glossoepiglottic fold, overlying the hyoepiglottic ligament, connects the epiglottis to the tongue in the midline and the lateral glossoepiglottic folds (pharyngo-epiglottic folds)5 connect it to the lateral pharyngeal walls.3,5 The depressions formed between these folds are termed the valleculae5,6 and are considered to be within the oropharynx.6 During direct laryngoscopy, the Macintosh blade is inserted into the base of the vallecula to engage the hyoepiglottic ligament and thereby move the epiglottis anteriorly to expose the glottis.
The laryngopharynx (also referred to as the hypopharynx) extends from the epiglottis to the lower border of the cricoid cartilage, and is continuous with the esophagus (see Figures 3–15 and 3–17).3,4,16 The upper border of the laryngopharynx has been located at the tip of the epiglottis by some authors2,3,9,16,24 and the base of the epiglottis by others.6,25 The oblique inlet (aditus) of the larynx is bounded by the epiglottis, aryepiglottic folds, arytenoid cartilages, and the posterior commissure.3,5,24 The cylindrical larynx itself bulges posteriorly into the center of the laryngopharynx creating a deep recess, the piriform fossa (or sinus), on either side leading into the esophagus.3,4 As seen during direct laryngoscopy, the larynx can be conceptualized to be a smaller cylinder eccentrically placed within and at the anterior aspect of the larger cylindrical pharynx. The piriform fossae are bounded medially by the free upper end of the larynx5 formed primarily by the quadrangular membrane6 and laterally by the thyroid cartilage and the thyrohyoid membrane.3,5 Superiorly the piriform fossae are bounded by the lateral glossoepiglottic folds.2
Sensation to the nasopharynx and oropharynx is supplied primarily by the glossopharyngeal nerve.3,5 The glossopharyngeal nerve enters the neck in company with the internal carotid artery and the internal jugular vein.26 At the level of the styloid process, it leaves this position and winds anteriorly and inferiorly lateral to stylopharyngeus which it supplies (see Figure 3–18).8 The nerve then passes forward between the superior and middle constrictors and gives off pharyngeal branches as well as lingual branches to the posterior third of the tongue (see Figure 3–12).8 Glossopharyngeal nerve blocks can be performed posterior to the midpoint of the palatopharyngeal fold or at the base of the palatoglossal fold in the mouth. The maxillary branch of the trigeminal nerve supplies sensation to the roof of the nasopharynx and contributes to the sensory supply of the soft palate and the adjacent part of the tonsil.3,5 The laryngopharynx receives sensory innervation from the internal branch of the superior laryngeal branch of the vagus nerve, which pierces the thyrohyoid membrane and runs in the submucosa of the piriform fossae.3 This nerve also supplies sensation to the larynx above the level of the false or true cords.5,8,27–29 Cotton pledgets soaked in local anesthetic can be held against the mucosa of the piriform fossa using Kraus or Jackson forceps to produce a block of the internal branch of the superior laryngeal, or the nerve can be approached percutaneously. The glossopharyngeal nerve also supplies sensation to the upper portion of the laryngopharynx.6 Some authors state that the superior aspect (pharyngeal surface) of the epiglottis is innervated by the glossopharyngeal nerve, whereas the inferior aspect (laryngeal surface) receives sensory innervation from the superior laryngeal nerve (SLN).28,30 Others have stated that both surfaces of the epiglottis are innervated by the superior laryngeal branch of the vagus.27
The larynx is a complex structure made up of a framework of cartilages and fibroelastic membranes covered by a layer of muscles and lined by mucous membrane.3,4 It functions as an open valve during respiration, a partially closed valve during phonation, and as a closed value during swallowing and when increased intrathoracic pressure is required (Valsalva maneuver).2,3,6,16 The larynx extends from its oblique entrance to the lower border of the cricoid cartilage and bulges posteriorly into the laryngopharynx (see Figure 3–15).3,5 It is suspended from the hyoid bone which is itself attached to the mandible, tongue, and the base of the skull.16
The laryngeal cartilages include the thyroid, cricoid, epiglottic and the paired arytenoid, corniculate, and cuneiform cartilages (see Figure 3–19). The quadrilateral laminae of the thyroid cartilage meet in the midline anteriorly to form the laryngeal prominence (Adam’s apple) and thyroid notch. Posteriorly, the border of the thyroid cartilage is free and prolonged into paired upper and lower horns.5,6 Superiorly, the thyroid cartilage is attached to the hyoid by the thyrohyoid membrane.3 Posteriorly the lower horns of the thyroid cartilage articulate with the posteriorly oriented signet ring shaped cricoid cartilage. Anteriorly the thyroid cartilage is attached to the cricoid by the CTM, a suitable site for emergency surgical airway access in the adult. The cricoid cartilage is the only complete skeletal ring of the airway and can be used to provide cricoid pressure (Sellick’s Maneuver) during rapid sequence induction/intubation. The paired arytenoid cartilages articulate with the superior aspect of the cricoid cartilage posteriorly. The corniculate cartilages in turn articulate with the apices of the pyramidal-shaped arytenoids and are located within the aryepiglottic fold.3,6 The shallow depression between the two corniculate cartilages (the posterior commissure) is a useful landmark during laryngoscopy.3,6 The cuneiform cartilages are located lateral to the corniculate cartilages and also lie within the aryepiglottic folds. The leaf-shaped epiglottis is attached to the thyroid cartilage inferiorly by the thyroepiglottic ligament, and to the hyoid bone superiorly by the hyoepiglottic ligament.3 The remaining framework of the larynx consists of two paired fibroelastic membranes, the quadrangular and triangular membranes (see Figure 3–20).3,6 The quadrangular membrane spans the space between the lateral border of the epiglottis and the arytenoid cartilages.3,6 Its slightly thickened free upper edge forms the aryepiglottic ligament at the laryngeal aditus, and its markedly thickened lower border forms the vestibular ligament (false vocal cord).3,5 The triangular ligament is attached in the midline anteriorly to the thyroid and cricoid cartilages and extends posteriorly to attach to the arytenoid.5,6,19 The inferior border of the triangular ligament is attached obliquely to the cricoid cartilage, whereas the upper border is free and thickened to form the vocal ligament (true vocal cord).3,6 In coronal section, the relationship of the true vocal cords to the false vocal cords and the laryngeal ventricle or sinus (between the true and false cords) can be readily appreciated (see Figure 3–21).3,6 The aryepiglottic, the vestibular (false cords), and the vocal folds (true cords) form a trilevel sphincter mechanism that regulates and protects the airway.3,6 The folds also divide the larynx into three parts: the supraglottic compartment or vestibule above the false cords, the glottic compartment between the false and true cords, and the infraglottic compartment between the true cords and the lower border of the cricoid.3,5,16 The absence of a submucosal layer at the vocal ligament causes the cords to appear white and limits the extent to which they can swell in edematous conditions.16 In the adult the distance from the “teeth” to the vocal cords has been quoted to be 12 to 15 cm,19,31 or from the incisors, an average of 16 cm.32
A complex arrangement of intrinsic muscles alters the configuration of the laryngeal folds. The cricothyroid muscle is classified by itself as the only extrinsic muscle of the larynx and muscles that elevate or depress the larynx as a whole (e.g., during swallowing) are considered to be accessory laryngeal muscles.3,6
The larynx receives its nerve supply from the superior and recurrent laryngeal branches of the vagus nerves.3,4 The SLN arises from the vagus just below the pharyngeal plexus, passes medial to both the internal and external carotids, and then divides into a large sensory internal and a small motor external branch which supplies the cricothyroid muscle (see Figure 3–22).3,4,8 The internal branch pierces the thyrohyoid membrane to provide sensation to the laryngeal mucosa above the level of the false3,6,28,29 or true cords.5,8,27
On the right side, the recurrent laryngeal nerve leaves the vagus as it crosses the subclavian artery, loops posteriorly under the artery, and ascends to the larynx in the groove between the esophagus and trachea. On the left, the nerve leaves the vagus as it crosses the aortic arch and similarly loops posteriorly under the arch and then runs superiorly between the esophagus and the trachea to reach the larynx.3,4 The recurrent laryngeal nerves supply all the intrinsic muscles of the larynx and provide sensation below the level of the false3,6,28,29 or true cords.5,8,27 Hoarseness produced by damage to a single superior laryngeal nerve may be temporary as the contralateral cord exerts a compensatory action.3,4 Damage to the recurrent laryngeal nerve also produces hoarseness and if both nerves are affected, severe airway obstruction can occur.3,16
The CTM or ligament can be identified in the anterior neck as a concavity between the convex inferior border of the thyroid cartilage and the superior portion of the cricoid cartilage (see Figures 3–19 and 3–23). It is trapezoidal in shape with a cross-sectional area of 2.9 cm2 and a mean height of 9 mm (range 5–12 mm).33,34 The average vertical distance between the true cords and the midpoint of the CTM is 13 mm in the adult.33 The vertical distance from the lower border of the thyroid cartilage to the vocal cords is 5 to 11 mm.34,35 The cricothyroid branches of the superior thyroid arteries run transversely across the membrane, usually the upper third,34,35 and tributaries of the anterior jugular veins occasionally run anterior to the membrane, although considerable variation exists in the vascular pattern.34 During cricothyrotomy or membrane puncture, the CTM should be traversed at its inferior third to minimize vascular injury.34,35
The trachea extends inferiorly from its junction with the larynx at the lower border of the cricoid cartilage to the carina (see Figure 3–24).3,4,36 It is approximately 10 to 15 cm in length in the adult and about 12 to 23 mm in diameter.3–5,29,31 The inferior half of the trachea lies within the superior mediastinum.5 The cervical trachea is in the midline; however, the intrathoracic portion is deviated to the right by the aortic arch (see Figure 3–25).4 The patency of the trachea is maintained by 16 to 20 U-shaped rings of hyaline cartilage5 joined by fibroelastic tissue and closed posteriorly by the trachealis muscle.3,4 Longitudinal mucosal markings can be seen posteriorly when the trachea is viewed through the bronchoscope, and these can be used for spatial orientation (see Figure 3–26). The average distance from the central incisors to the carina is 27 cm in the adult male and 23 cm in the adult female.23 A teeth to carina distance of 22 to 30 cm in the adult has also been quoted.19,37 The distance from the nostrils to the carina is an additional 2 to 4 cm.23,31 Tracheotomy is usually performed between the second and third or third and fourth tracheal rings.3,4,34
Awake intubation is most often performed in the adult population, and this chapter is directed to this age group. Management of the pediatric airway does require consideration of the anatomic differences in children. The reader is directed to the pediatric sections of this book for further information (see Chapter 45).
What Drugs Are Useful for Airway Anesthesia? What Are Their Toxicities and Associated Complications?
Lidocaine, tetracaine, cocaine, benzocaine, and dyclonine have all been used to produce topical anesthesia of the airway, and lidocaine is commonly used to perform glossopharyngeal and SLN blocks.
Introduced in 1948, lidocaine, an aminoethylamide, is the prototypical member of the amide class of local anesthetics and is metabolized in the liver by mixed function oxidases.38 The metabolites and 2% to 3% of unchanged drug are excreted by the kidneys.39 The main metabolites are monoethylglycinexylidide (MEGX), glycinexylidide (GX), 2,6 dimethylaniline, and 4-hydroxy-2,6-dimethylaniline.39 The local anesthetic and toxic profiles of MEGX and GX are similar to but less potent than those of lidocaine.39,40 Hepatic metabolism appears to be limited by liver perfusion41 as well as parenchymal disease such as cirrhosis,42 and the clearance of lidocaine is reduced in the presence of cardiac and hepatic insufficiency.40 Orally ingested lidocaine undergoes first pass metabolism in the liver43 and about 35% of an oral dose reaches the systemic circulation.44 Lidocaine is extensively bound to plasma proteins, particularly α1-acid glycoptotein.38 Lidocaine is probably the local anesthetic most commonly used for regional anesthesia of the airway and has also been used extensively in the past for the treatment of ventricular arrhythmias.45,46
Following application to the mucous membranes of the airway, lidocaine produces an anesthetic effect which is limited to the mucous membrane in 1 to 2 minutes.31,47 The peak anesthetic effect occurs within 2 to 5 minutes,45 and the duration of the airway anesthesia is said to be 30 to 40 minutes,45 20 to 40 minutes,48 10 to 15 minutes,49 or 15 to 30 minutes.50 Watanabe et al.51 found the duration of anesthesia following the application of lidocaine to the oral mucosa to be 40 minutes with glycopyrrolate pretreatment, and 20 minutes without it. Schonemann et al.52 sprayed 10% (100 mg·mL−1) lidocaine onto the oral mucosa of the lower lip and demonstrated a hypoalgesic effect that lasted 14 mintues.52 Maximum hypoalgesia was observed after 4 to 5 minutes. Adriani et al.47 found that the maximum effective concentration of topical lidocaine applied to the tongue was 4% (40 mg·mL−1), and that this concentration had a latent period of 2 minutes, and a duration of effect of 15.2 minutes. The latent period has a profound significance when bronchoscopic intubation is performed using a “spray-as-you-go” technique and suggests that a significant risk of insufficient anesthesia may exist.52 Concentrations of lidocaine of 1% (10 mg·mL−1) to 10% (100 mg·mL−1) have been used for topical anesthesia of the airway. Excellent topical anesthesia of the airway in the adult can be produced by 4% lidocaine, but at 2% (20 mg·mL−1) concentration, topical anesthesia may be inadequate, and 1% lidocaine has been found to be insufficient for airway instrumentation.53 Increasing the concentration beyond the optimum level does not affect the latent period or duration of action,47 and increasing the dose to a given area of mucosa beyond 15 mg of lidocaine per square centimeter does not increase the anesthetic effect.52 Importantly, topical epinephrine penetrates mucous membranes poorly, has no significant local effect, does not prolong the duration of topical local anesthesia,45 and will not slow the rate of anesthetic absorption.54
Local anesthetics applied to the mucous membranes of the airway are rapidly absorbed into the circulation.45 The extent of systemic absorption depends on the site and technique of application, tissue vascularity, the total dose administered,55,56 the state of the mucosa, the concomitant use of drying agents,51,57 the amount of mucous present, the rate and depth of respiration, the state of the circulation, the patient’s disease state,58,59 and individual variation.58,59 Absorption is particularly rapid when local anesthetics are applied to the tracheobronchial tree,45 whereas decreased rates of absorption occur in the upper airway secondary to decreased vascularity and surface area.56 Absorption from alveoli may approximate IV administration60 due to osmotic relationships in the pulmonary vascular bed designed to prevent the collection of fluid in the alveolar spaces.47,61 The therapeutic serum concentration of lidocaine when the drug is used as an antiarrhythmic is usually considered to be 1.5 to 4.0 µg·mL−1,58 or 1.5 to 5 µg·mL−1.62 As serum lidocaine concentrations increase, however, systemic toxicity is produced. The commonly accepted toxic plasma concentration of lidocaine has been said to be 5 µg·mL−1.63 At the upper limit of the antiarrhythmic therapeutic range, lightheadedness, tinnitus, and circumoral and tongue numbness can occur.64 As serum concentrations continue to rise, visual disturbances and muscle twitching occur and can be followed by generalized seizure activity.64 Seizures are most frequently seen at plasma concentrations more than 8 to 10 µg·mL−1,60,65 although they have occurred at plasma concentrations as low as 6 µg·mL−1.56 Nystagmus is an early sign of toxicity.38 However, excitatory manifestations of toxicity may be brief or not occur at all39 and lidocaine can produce loss of consciousness preceded only by sedation.38 Coma and respiratory arrest can occur at plasma concentrations of 15 to 25 µg·mL−1,66 and cardiorespiratory arrest at 20 to 25 µg·mL−1.56,64 Levels in arterial blood have been shown to be 20% to 30% higher than those in venous samples57,65,67 and more closely correlate with CNS effects.68 However, most clinicians consider venous blood levels to be reliable indicators of clinical toxicity.56 Acidosis increases lidocaine toxicity.39 Hypersensitivity reactions to lidocaine, although exceedingly rare, can also occur and can be catastrophic.48 Methylparaben, used as a preservative, can also be responsible for some allergic reactions.39 Clinically significant methemoglobinemia has been reported in association with lidocaine administration, although these occurrences have been extremely rare.69–73 A 2009 review of the literature found 12 episodes of methemoglobinemia related to lidocaine without associated prilocaine or benzocaine use.74 In seven of these cases an oxidative drug had been administered concomitantly. In one case the episode occurred within 24 hours of exposure to benzocaine, and one case occurred after the chronic abuse of lidocaine gel. Of the remaining three cases, one developed cyanosis about 21 hours after lidocaine injection.70 Methemoglobin levels were not reported but the patient responded to methylene blue.70
The maximum safe dose of topical lidocaine has been stated to be 4 mg·kg−1,75–80 3 to 4 mg·kg−1,50 and 6 mg·kg−1.23 However, when topically applied to the mucous membranes of the airway, these maximal doses can only be interpreted when the method of topical administration is known. The exact dose of lidocaine delivered to the airway when an inhalational technique is used is difficult to measure,68 and the dose administered may bear little relation to the dose actually absorbed.81 Lidocaine administered to the airway by nebulization has been reported to produce low peak plasma concentrations as compared to other techniques.57,68,82,83 This has been attributed to the loss of up to 50% of the nebulized solution to the environment with continuous nebulization.68,84 It has been shown that only about 7% to 12% of the nebulized dose of a drug actually reaches the lung.85,86 Any anesthetic expectorated after administration must also be subtracted from the total dose administered.
When the use of lidocaine is discussed in the context of topical anesthesia of the airway it is important to understand what the terms “aerosolization,” “atomization,” and “nebulization” mean. “Atomization” and “nebulization” are often used interchangeably and the terminology can be confusing. An aerosol can be defined as a suspension of particulate matter (such as droplets) in a gaseous medium (such as air or oxygen).87,88 Aerosolization is the process of generating an aerosol. Atomizers use a driving gas and a Venturi to generate droplets from a solution that are typically 15 to 500 µm in diameter.89 Jet and ultrasonic nebulizers incorporate baffles which selectively remove large droplets and deliver particles mostly 1 to 5 µm in diameter.89 Vibrating mesh nebulizers use micropump technology to similarly generate small particle size.88,90
Deposition of the aerosol particles in the airway depends to a large extent on the particle or droplet size.89 The peak deposition of aerosol droplets occurs in the peripheral airway for droplets of about 2 µm, in bronchioles for droplets of about 8 µm, in bronchi for droplets of about 15 µm, and in the upper airway for droplets larger than 40 µm.91 Higher oxygen flow rates through nebulizers create smaller droplets (less than 30 µm) that travel further distally into the bronchial tree and increase the rate of absorption.50 A droplet diameter between 1 and 5 µm is preferable for central and deep lung penetration92 and a diameter <2 µm may be ideal for penetration to the most distal parts of the lung.93 Particles <0.5 µm may avoid deposition altogether and be exhaled.89
Droplets larger than 60 µm are preferred for airway anesthesia during awake intubation because they “rain out” in the proximal airway where the topical anesthesia is required.50 The droplet size produced by manually squeezing the bulb of the hand-held DeVilbiss #40 nebulizer tends to be much larger than that produced by conventional aerosol delivery systems and is dependent on the pressure generated in the nebulizer.94 The mean bulb pressure produced by a firm squeeze is 250 to 340 mm Hg (4.86–6.01 psi). The mass median diameter (MMD) of the droplets thus produced was found to be 6.2 to 12.0 µm with 28% to 50% of the particles being less than 6.2 µm in diameter, small enough to penetrate the tracheobronchial tree.94 With increasing firmness of the manual squeeze and increased bulb pressure, these nebulizers increase output and decrease MMD. When an oxygen flow rate through the RD 15 DeVilbiss atomizer of 5.0 L·min−1 was utilized, pressures of 3.8 to 4.0 psi were generated at the inlet of the device, and at 8 L·min−1 oxygen flow, the measured pressure was 11.2 to 11.4 psi. The use of higher low rates may produce smaller droplets and increase systemic absorption. Based on extensive clinical experience, 8 L·min−1 seems to be an optimal flow rate when an atomizer is used to produce topical anesthesia of the airway. Deposition of droplets in the upper airway is also a function of velocity, turbulent flow, spray cone angle, and administration angle.88,95,96 When used for topical anesthesia of the airway, lidocaine is best delivered by atomizers (as opposed to nebulizers) as rain out of the droplets occurs in the upper airway where the therapeutic effect is required.
Many investigators have endeavored to link the method of administration and dosage of lidocaine used for airway anesthesia to plasma levels and toxicity:
In a study performed by Melby et al.,97 1.5 mg·kg−1 of 4% lidocaine was injected into the endotracheal tube of six patients under general anesthesia. Peak serum lidocaine concentrations of 1.4 to 3.3 µg·mL−1 occurred at 11.7 ± 5.2 minutes following administration. Three of the six patients experienced almost instantaneous absorption.
Chu et al.91 compared plasma lidocaine concentrations produced by tracheal spraying of 3.3 mg·kg−1 of 4% lidocaine with those produced by IV injection of 1 mg kg−1 of 2% lidocaine. After tracheal spraying, maximum plasma lidocaine levels of 2.0 to 5.6 µg·mL−1 were recorded at 15 to 20 minutes. Following IV administration, peak concentrations of 5.0 to 6.85 µg·mL−1 were reached within 2 minutes.
Curran et al.98 measured the concentration of lidocaine in venous blood following tracheal as compared to laryngeal spraying in 10 patients under general anesthesia. Each group received 3 mL of 10% lidocaine. The peak lidocaine concentration in the tracheal group ranged from 1.9 to 8.2 µg·mL−1, whereas in the laryngeal group the range was 0.4 to 2.5 µg·mL−1. Lidocaine levels in the tracheal group tended to rise more rapidly and reach a peak earlier than in the laryngeal group.
Eyres et al.99 administered 4 mg·kg−1 of 4% lidocaine into the larynx and immediate subglottic area of 96 children under general anesthesia and measured plasma lidocaine levels at 2, 4, 6, 10, 15, 20, and 30 minutes after administration. Mean peak lidocaine levels measured were 4.3 ± 1.9 µg·mL−1 for those less than 1 year of age, 5.7 ± 2.0 for those 1 to 3 years of age, 5.3 ± 1.4 for those 3 to 5 years of age, and 5.3 ± 2.0 for those more than 5 years of age. Plasma lidocaine levels exceeded 8.0 µg·mL−1 in 13 patients. The time to peak concentration varied from 8.5 ± 2.5 minutes in those less than 1 year of age to 11.7 ± 4.3 minutes in those more than 5 years of age.
Parkes et al.57 administered 6 mg·kg−1 of 10% lidocaine to 10 ASA I volunteers via a nebulizer connected to a face mask powered by an oxygen flow of 6 L·min−1. The mean peak serum concentration of lidocaine produced was 0.29 µg·mL−1, and the highest measurement was 0.45 µg·mL−1. The peak concentration occurred 30 minutes after nebulization was commenced.
Chinn et al.84 administered 10 mL of 4% lidocaine to five healthy subjects using a DeVilbiss 35B ultrasonic nebulizer connected to a Hudson oxygen mask. Plasma lidocaine levels were measured at the start of nebulization, at 5-minute intervals for 30 minutes, and then at 45, 60, 90, and 120 minutes after the start of nebulization. The mean peak plasma level occurred at 10 minutes and, as measured from the published graph, was 0.95 µg·mL−1.
Mostafa et al.86 similarly administered 6 mg·kg−1 of 10% lidocaine to 14 ASA I and II patients scheduled for head and neck surgery using a nebulizer system with a mouthpiece and an oxygen flow of 7 L·min−1. The mean plasma lidocaine level 10 minutes after nebulization was 0.95 ± 0.62 µg·mL−1, and at 20 minutes it was 0.68 ± 0.32 µg·mL−1.
Sutherland and Williams100 administered 5 mL of 4% lidocaine to 20 adult patients using a standard nebulizer connected to a mouthpiece and an oxygen flow of 8 L·min−1. The mouthpiece was connected to an oral airway intubator that was advanced into the oropharynx during the latter part of the nebulization process. The subjects also gargled 5 mL of 2% lidocaine gel for 1 minute, and 2 mL of 2% lidocaine was injected through the bronchoscope onto the vocal cords, as well as 2 mL into the trachea. The dosage range was 2.5 to 11 mg·kg−1 (mean dose, 5.3 ± 2.1 mg·kg−1). The mean peak plasma concentration was 0.7 ± 0.4 µg·mL−1, and the highest plasma concentration was 1.6 µg·mL−1. The mean peak concentration occurred 23 minutes following intubation.
Kirkpatrick et al.101 administered 10 mL of nebulized 4% lidocaine to 10 normal volunteers using a DeVilbiss 646 nebulizer, an oxygen flow of 6 L min−1, and a mouthpiece during tidal breathing. Blood was sampled at 10, 20, 30, 40, and 50 minutes after the start of nebulization. In three subjects additional samples were obtained at 60, 80, and 120 minutes. The mean peak serum lidocaine level was 0.52 µg·mL−1 and occurred 20 minutes after beginning nebulization. The highest single measurement was 1.05 µg·mL−1.
Wieczorek et al.102 administered topical lidocaine to a group of 27 obese patients prior to bronchoscopic intubation. Forty milliliters of either 2% lidocaine (14 patients) or 4% lidocaine (13 patients) was administered using a DV-15-RD atomizer and an oxygen flow of 10 L·min−1. Plasma lidocaine concentrations were measured at intervals from before atomization to 120 minutes following the completion of atomization. Peak plasma concentrations were recorded in the 10-minute samples in both groups. The mean peak level in the 4% group was 6.5 µg·mL−1 (standard error of the mean, 1.0 µg·mL−1). In the 2% group, the peak level was 2.8 (0.8) µg·mL−1. No clinical signs of lidocaine toxicity were detected.
Woodruff et al.103 subsequently reported a prospective randomized blinded study from the same center which compared 1% lidocaine with 2% lidocaine using the same atomization technique. Forty milliliters of either 1% or 2% lidocaine was administered. Mean peak plasma lidocaine levels were measured 5 minutes after completion of topicalization and were 3.8 (0.5) µg·mL−1 in the 2% group and 1.4 (0.3) µg·mL−1 in the 1% group. No signs of lidocaine toxicity were detected.
Gomez et al.104 measured serum lidocaine levels in 29 patients who underwent bronchoscopy and who had between 180 and 400 mg of lidocaine instilled onto the tracheobroncheal muscosa. Blood was sampled at intervals between 10 minutes and 2 hours after administration in 9 patients, and from 45 minutes to 2 hours in 20 patients. In the group who had the whole serum concentration curve determined, the average maximum serum concentration was 1.21 ± 0.64 µg·mL−1. The peak concentration occurred at 34.02 ± 10.74 minutes. The average serum level 2 hours after administration was 0.69 ± 0.29 µg·mL−1 and the average serum half-life of lidocaine was 1.35 ± 0.41 hours. The percentage of lidocaine absorbed was calculated to be between 60.88% and 20.89% with an average value of 36.55 ± 13.95%.
Patterson et al.105 reported the administration of up to 380 mg of lidocaine almost entirely delivered as a 1% solution through the bronchoscope to 21 adult patients. With the exception of one patient, the maximum blood levels of lidocaine recorded were <2.48 µg·mL−1. The peak concentration occurred between 5 and 75 minutes following lidocaine administration, usually between 5 and 30 minutes. Considerable individual variation in the maximum concentration measured was noted. One patient with abnormal liver function was found to have a plasma concentration of 18.2 µg·mL−1 but developed no signs of toxicity.
Xue et al.43 performed a randomized double-blind study which compared 2% and 4% lidocaine administered via the bronchoscope using a spray-as-you-go technique in a group of 52 sedated patients prior to bronchoscopic intubation. The mean dose of lidocaine administered was 3.4 ± 0.6 mg·kg−1 (range 3.2–4 mg·kg−1) in the 2% group, and 7.1 ± 2.1 mg·kg−1 in the 4% group (range 6.1–8.1 mg·kg−1). The highest lidocaine concentration measured in the 2% group was 2.0 µg·mL−1, and in the 4% group the highest concentration measured was 3.6 µg·mL−1. Concentrations above 3 µg·mL−1 occurred in only three patients, all in the 4% group. In most patients the peak lidocaine concentration was observed at 20 to 30 minutes after lidocaine administration. Again, significant individual variation in plasma levels was noted.
Boye and Bredesen106 administered about 7 mL of 4% lidocaine to nine adult patients prior to bronchoscopy utilizing nebulization, a topical applicator, instillation, and injection through the bronchoscope. Blood was sampled about 8 minutes after the start of anesthesia and after bronchoscopy, about 38 minutes after the start of anesthesia. The mean maximum plasma concentration of lidocaine was 2.7 µg·mL−1. No clinical signs of lidocaine toxicity occurred.
Bigeleisen et al.107 administered a total of 7 mg·kg−1 of lidocaine to a group of 20 patients who underwent bronchoscopic intubation. Four mg·kg−1 of atomized 4% lidocaine was applied to the nose and nasopharynx and percutaneous SLN blocks were performed using 0.5 mg·kg−1 of 1% lidocaine. The patients were then randomized into two groups. Group 1 underwent transtracheal injection of 2 mg·kg−1 of 4% lidocaine, whereas group 2 received an additional equivalent dose of 4% lidocaine applied to the nasopharynx. Plasma lidocaine levels were measured every 2.5 minutes for 10 minutes after the last dose of lidocaine and then every 5 minutes for an additional 20 minutes. The mean peak lidocaine level in the transtracheal group was 4.06 µg·mL−1 and occurred at 10 minutes. The highest level recorded was 6.04 µg·mL−1. In the nasotracheal group, the mean peak level was 3.16 µg·mL−1 and occurred at 7.5 minutes. The highest level was 6.57 µg·mL−1. No signs of systemic toxicity were detected.
Loukides et al.62 measured plasma lidocaine levels for up to 2 hours after the start of topicalization in 12 patients who underwent flexible bronchoscopy. The lidocaine was administered as a 2% gel to the nose and a 2% solution injected through a laryngeal syringe or the bronchoscope. The mean total dose administered was 622±20 mg (range 500–720 mg). Peak plasma concentrations were observed at 20 minutes in eight patients, at 30 minutes in three patients, and at 60 minutes in one patient. The highest value measured was 2.25 µg·mL−1.
Efthimiou et al.55 measured plasma concentrations of lidocaine in a group of 41 patients who underwent topical airway anesthesia before flexible bronchoscopy. In 32 patients, 14 sprays of 10% lidocaine were administered to the nose and oropharynx followed by 8 mL of 4% lidocaine solution administered via the bronchoscope to the pharynx and vocal cords, and 14 mL of 1% lidocaine to the bronchial tree. In nine patients, 8 mL of 2% lidocaine gel was applied to the nose and the use of the 10% spray was omitted. The average dose of lidocaine administered was 9.3 ± 0.5 mg·kg−1. The average peak plasma concentration was 2.9 ± 0.5 µg·mL−1 and correlated with dose per unit body weight. Two patients had plasma levels above 5 µg·mL−1 but demonstrated no clinical evidence of toxicity. The average time to peak concentration was 42.6 minutes in the aerosol group and 48.4 minutes in the gel group. In a second study of 10 volunteers, plasma concentrations following 4% lidocaine gargle and swallow was compared with 10% oropharyngeal spray. The dose in each group was 6.8 mg·kg−1. The gargle produced a peak concentration of 2.4 µg·mL−1, whereas the spray produced a peak concentration of 1.9 µg·mL−1 at 50 minutes.
Reasoner et al.108 randomized 40 adult patients undergoing awake bronchoscopic intubation and surgery for cervical spine instability into topical and nerve block groups. Up to 20 mL of 4% lidocaine was administered to the topical group via a nebulizer attached to a face mask using a flow rate of 10 L·min−1. Nebulization required about 10 minutes and was followed by cricothyroid puncture and injection of an additional 3 mL of 4% lidocaine. In the nerve block group, airway anesthesia was achieved with 50 mg of 10% lidocaine spray applied to the tongue, bilateral glossopharyngeal nerve block at the palatoglossal fold using 0.5 to 1.0 mL 2% lidocaine, SLN block using 1 to 2 mL 2% lidocaine, and 3 mL 4% lidocaine injected through the CTM. Arterial blood was sampled for the plasma lidocaine level following administration of local anesthesia, 2 minutes prior to intubation (time zero), and again 10 minutes later. The topical group received 815 ± 208 mg of lidocaine whereas the nerve block group received 349 ± 44 mg. Mean plasma lidocaine levels at time zero were 2.16 ± 1.48 µg·mL−1 in the topical group, and 4.23 ± 1.12 µg·mL−1 in the nerve block group. Ten minutes later, the levels were 3.34 ± 1.87 µg·mL−1 and 4.02 ± 1.02 µg·mL−1, respectively. No plasma sampling was performed after the 10-minute recording. The quality of anesthesia achieved was similar with both techniques, and intubation was achieved in 3.2 minutes on average. No complications were identified.
Langmack et al.109 administered topical lidocaine to 51 asthmatic volunteers who underwent research bronchoscopy. Atomized 4% lidocaine was sprayed into the nose and throat, 2 mL of viscous lidocaine was applied to the nose, and 1% lidocaine was administered to the tracheaobronchial tree via the bronchoscope. The 2% viscous was excluded from the total dose calculation and the authors considered absorption of intranasal lidocaine to be less than 50%. The mean calculated total dose administered was 8.2 ± 2.0 mg·kg−1 (range 4.3–14.3 mg·kg−1). The venous serum lidocaine concentration was measured at 30 minutes after topical anesthesia was completed (T1) and 30 minutes after the bronchoscopy was completed (T2). The serum lidocaine concentration ranged between 0.1 and 2.90 µg·mL−1 at T1 and between 0.5 and 3.2 µg·mL−1 at T2. The serum lidocaine concentration correlated with the total dose administered although considerable individual variability among subjects who received the same dose was noted. No signs or symptoms of lidocaine toxicity were detected.
Williams et al.63 measured serum concentrations of lidocaine in 25 participants in a bronchoscopy training course. Five milliliters of 4% lidocaine was administered by nebulizer, 2 mL of 5% lidocaine with 0.5% phenylephrine was sprayed into the nose, and 4 mL of 10% lidocaine was sprayed into the oropharynx. Four percent lido was also administered via the endoscope. Seventy-five percent of the nebulizer dose was excluded from the total dose calculation. The average calculated dose administered was 8.8 mg·kg−1 (range 7.3–9.2 mg·kg−1). Serum lidocaine concentrations were measured before topicalization (T0), 20 minutes after topicalization (T20), and then at 10 minutes intervals until 60 minutes after the last dose of lidocaine. The duration of sampling ranged from 100 to 120 minutes. The highest recorded lidocaine concentration was 4.5 µg·mL−1 and only two subjects experienced peak levels above 3 µg·mL−1. In 8 of the 25 subjects, the highest lidocaine concentration was observed in the final sample, 60 minutes after the last dose of lidocaine was administered. Multiple symptoms of lidocaine toxicity were reported by the subjects including lightheadedness, dysphoria, nausea, and shivering.
Berger et al.110 performed transnasal flexible bronchoscopy on 21 normal volunteers and 18 patients under topical lidocaine anesthesia administered using a combination of gargle, atomization (spraying), nebulization, gel, and in the 18 patients, endobronchial instillation. The total mean dose of lidocaine administered to the 18 patients was 2086 mg and to the volunteers 1534 mg. Plasma lidocaine levels were measured in eight patients and six volunteers after gargling, after spraying, after nebulization, and then at 5, 10, 15, 30, and 60 minutes. The mean peak level in the patients was about 3.1 µg·mL−1 and in the volunteers about 1.0 µg·mL−1 as measured from the published graphs. In the patient group the peak concentration occurred at 30 minutes. In the volunteers, the peak occurred after nebulization and this level was sustained until the 30-minute sample.
Ameer et al.111 administered topical lidocaine for bronchoscopy to 19 adults using a combination of lidocaine gargle, atomized lidocaine, lidocaine jelly, and lidocaine solution injected through the bronchoscope. The time over which lidocaine was administered was 0.79 ± 0.31 hours in 5 young patients and 0.69 ± 0.22 hours in 14 elderly subjects. The total dosage administered was 19.01 ± 1.67 mg·kg−1 in the young adults, and 17.15 ± 2.28 mg·kg−1 in the elderly. The mean maximum plasma concentrations achieved were 3.04 ± 1.27 µg·mL−1 in the young and 2.40 ± 0.92 µg·mL−1 in the elderly. The times required to reach peak levels were 0.77 ± 0.28 hours in the young and 1.21 ± 0.55 hours in the elderly. No serious drug toxicity occurred.
Sucena et al.112 measured plasma lidocaine levels in 30 patients undergoing flexible bronchoscopy. The mean total dose of lidocaine administered as a 2% gel, 10% spray, and 2% solution was 11.6 ± 3.1 mg·kg−1. Plasma lidocaine levels were 3.2 ± 1.7 µg·mL−1 at 20 minutes, 3.3 ± 1.7 at 30 minutes, and 3.0 ± 1.5 at 40 minutes after the start of anesthesia. The plasma lidocaine concentration exceeded 5 µg·mL−1 in six patients but no signs of toxicity were exhibited.
Reference has also been made to peak blood levels not occurring until 90 minutes after completion of bronchoscopy113 and occurring 30 to 90 minutes from the start of airway local anesthesia.62
Toxicity associated with lidocaine topically applied to the airway can however occur.
Martin et al.114 administered 7.14 to 14.77 mg·kg−1 of topical lidocaine (median dose 9.6 mg·kg−1) to 39 volunteers participating in a bronchoscopy training study. The airway topicalization included 2% viscous lidocaine gargle, 10% spray, and 2% lidocaine administered through the bronchoscope. Thirty-six of the 39 subjects reported side effects associated with lidocaine topicalization including drowsiness, disorientation, hyperacusis, disinhibition, lightheadedness, visual disturbance, and dysphoria. Tremulousness was clinically evident in three subjects who received 9.7, 10.2, and 13.4 mg·kg−1 respectively, two of whom had a single involuntary limb movement. No serum lidocaine concentrations were measured. No major adverse events occurred.
Wu et al.56 reported a grand mal seizure which occurred following the topical application of 300 to 320 mg of lidocaine applied as a 4% spray to the larynx and 10 to 12 mL of 1% viscous lidocaine to the oropharynx and trachea of a 30-year-old, 48-kg female with renal failure, congestive heart failure, cardiomyopathy, and abnormal liver function tests. The plasma lidocaine level shortly after the seizure was 12 µg·mL−1. They also reviewed seven cases of seizure after the administration of topical lidocaine to mucous membranes of the airway. In each of these seven cases, the topical lidocaine was administered as 2% viscous or 2% to 4% solution.
Kotaki et al.65 in 1996 reported a seizure following the application of up to 800 mg of lidocaine as a 2% viscous preparation and 4% solution to the oropharynx. The serum lidocaine concentrations were found to be 11.6 µg·mL−1 and 9.0 µg·mL−1, 30 and 150 minutes postseizure, respectively. The authors reviewed three cases of seizure in addition to those reviewed by Wu et al. associated with topical application of lidocaine to the mucous membranes of the oral cavity and pharynx. In each of these three cases, the lidocaine had been administered as a 4% solution or 2% viscous preparation.
In 2014, Giordano et al.115 reported a grand mal seizure following the administration of about 200 mg of topical lidocaine as a 1% solution to a patient with tongue base abscess and airway compromise prior to planned awake flexible bronchoscopic intubation and after midazolam 4 mg IM. Twenty minutes after starting this procedure, the patient developed psychomotor agitation followed by generalized tonic clonic seizures and loss of consciousness. The serum lidocaine level was 5.1 µg·mL−1 20 minutes after the onset of the seizure. The authors postulated that hyperemia of the pharyngolaryngeal mucosa may have enhanced absorption of the lidocaine and that a preexisting respiratory acidosis may have lowered the seizure threshold.
In 1996, a healthy19-year-old female volunteer died as a result of lidocaine toxicity following a bronchoscopy performed as part of a research project.113 The amount of lidocaine administered was not documented in the record of the procedure. Details of the technique of administration, as determined from the references reviewed, were limited to “as a spray into the throat,” and that 4% lidocaine was administered in the upper airway and 2% in the lower airway. No reference to the use of atomized or nebulized lidocaine or documentation of the subject’s body weight was found in the references reviewed.113,116 The subject was left alone after release from the Medical Center and then found apparently having a seizure about an hour later. Emergency 911 was called and mouth to mouth breathing was initiated for apparent apnea. On arrival in the emergency department the subject was in cardiac arrest. The blood lidocaine level measured about 3 hours after the research procedure was 12.9 µg·mL−1. Based on this blood level of lidocaine it was reported that it was “likely that she had received in excess of 1200 mg of lidocaine.” The Medical Examiner’s Office found no evidence of any impairment in the subject’s ability to metabolize or detoxify lidocaine.113
In 2001 the British Thoracic Society recommended that the dose of lidocaine used for bronchoscopy be limited to 8.2 mg·kg−1 and that extra care be used in the elderly or those with liver or cardiac impairment.117 This maximum dosage recommendation appears to apply to all techniques, and the method of topicalization does not seem to have been taken into consideration. The Society guidelines note that in 1998 Milman et al.40 recommended that in patients with cardiac and hepatic insufficiency, the dose of lidocaine not exceed 5 mg·kg−1. The Thoracic Society of Australia and New Zealand, also in 2001, recommended that the total dose of lidocaine used for bronchoscopy not exceed 4 to 5 mg·kg−1.118
In summary, the maximum safe dose of lidocaine that can be topically applied to the mucous membrane of the airway is difficult to determine and must take into account the method of topicalization employed as well as the time course of administration. Traditional dosage guidelines may be excessively conservative when some or the entire dose is administered by aerosol, based on the available evidence with respect to serum levels and toxicity occurrences. Caution must be exercised however and a precalculated dose should not be exceeded. In clinical practice, the smallest amount of anesthetic sufficient to achieve the desired effect should be used110 and in general, for awake bronchoscopic intubation, the use of large doses is unnecessary. As always, clinical judgment is required and meticulous attention to detail should be employed when lidocaine is applied to the airway such that effective anesthesia is achieved without producing toxicity.
Several studies have looked at this issue:
Gal119 administered 4% lidocaine by ultrasonic aerosol to seven normal subjects and measured airway responses. After the inhalation of lidocaine, the subjects noted an impaired ability to swallow, and a husky voice suggesting vocal cord paresis. Three of the subjects described a sensation of obstruction during deep inspiration, although this was not reflected by significant changes in peak inspiratory flow (PIF) as recorded in maximum effort flow volume loops. Peak expiratory flow rates were also unchanged. Interestingly, statistically significant increases in maximum inspiratory flow were observed at 60%, 50%, and 40% of forced vital capacity (FVC) following the lidocaine aerosol. The author concluded that the administration of 4% lidocaine by ultrasonic nebulization produced mild bronchodilation and did not adversely affect airway function in normal subjects.
Gove et al.120 administered 10 mL of 4% lidocaine by means of an ultrasonic nebulizer attached to a mouthpiece to 33 patients prior to bronchoscopy. Five of the 33 patients required additional boluses of lidocaine to the bronchial tree during the procedure. Spirometry was recorded in 32 patients. A wide variation in individual response to the nebulized lidocaine was observed. Forced expiratory volume in 1 second (FEV1) varied between −18% and +45%, FVC between −27 and +18%, and peak expiratory flow rate (PEFR) between −41 and +34%. However, no overall effect on airflow was demonstrated. The bronchoconstriction that did occur was not clinically significant and bronchodilator therapy was not required.
Kuna et al.121 performed pulmonary function tests (PFTs) on 11 normal subjects before and after topical anesthesia of the airway. The topical anesthesia was achieved using 4% lidocaine spray to the soft palate and posterior oropharynx, an internal approach SLN block using cotton pledgets soaked in 4% lidocaine, and 1.5 mL of 10% cocaine applied to the epiglottis and vocal cords by means of a cannula under direct laryngoscopy. The PFTs consisted of flow volume loops, body box determinations of functional residual capacity (FRC), and airway resistance. The area under the inspiratory curve, PIF, and forced inspiratory flow (FIF) at 25%, 50%, and 75% of FVC were decreased after airway anesthesia, as was peak expiratory flow. However, the area under the expiratory curve and forced expiratory flow at 25%, 50%, and 75% FVC were unchanged. The authors noted that the configuration of the flow volume envelope following topical anesthesia in most subjects demonstrated a plateau or sudden reversible reduction in airflow on inspiration but a relative preservation of gas flow on expiration, and concluded that laryngeal anesthesia can compromise upper airway patency.
Liistro et al.122 measured specific airway conductance and maximum inspiratory and expiratory flow rates before and 15, 35, and 45 minutes after topical anesthesia of the upper airway. Anesthesia was achieved using four 10% lidocaine sprays to the oropharynx and hypopharynx, and 2 mL of 4% lidocaine solution instilled twice onto the vocal cords using a laryngeal syringe. Average values of maximum inspiratory flow rate (MIFR) decreased 15 minutes after upper airway anesthesia, but returned to control levels, or nearly so, at 45 minutes. Transient decreases in flow rates reaching zero flow in some subjects were observed in 13 of 16 subjects during forced inspiratory vital capacity (FIVC) and in 7 of 16 during forced expiratory vital capacity (FEVC) maneuvers. The site of obstruction to air flow was determined in 13 patients using simultaneous measurements of extraglottic pressure, flow rates, and lung volume. In 12 of these 13 patients, the site of obstruction was localized to the glottis, and in one, both supraglottic and glottic obstruction occurred. However, upper airway anesthesia in the absence of maximum forced respiratory maneuvers did not result in a decrease in flow rates. The authors concluded that topical anesthesia of the upper airway induces a glottic obstruction that produces a profound but transient decrease in maximum inspiratory and expiratory gas flow consistent with reflex regulation of upper airway caliber.
Beydon et al.123 measured airway flow resistance in nine healthy volunteers using a random noise forced oscillation technique before and after the application of topical anesthesia to the upper airway. On two separate occasions, either 100 mg of 5% lidocaine liquid was sprayed into one nostril and onto the mucosa of the pharynx and larynx and then gargled, or 100 mg of lidocaine paste was gargled. Airway flow resistance increased in all but one subject. On average the increase was 81% after lidocaine spray and 68% after lidocaine paste. The increased resistance lasted for 13 ± 3 minutes in the spray group and 12 ± 3 minutes in the paste group. At a separate session no change in resistance was detected 3 minutes after spraying normal saline into the upper airway., The two volunteers who experienced the greatest increase in airway flow resistance subsequently underwent transnasal flexible bronchoscopic laryngoscopy under topical lidocaine anesthesia during another study session. Dramatic changes were observed at the larynx. The vocal cords appeared slack and remained in a semi-closed position. During quiet inspiration the vocal cords moved medially producing incomplete obstruction at the glottis. During maximal inspiratory efforts the epiglottis moved toward the vocal cords to produce complete airway obstruction similar to that which occurs during swallowing. The authors concluded that topical lidocaine produces an increase in airway resistance in most normal subjects and that the larynx and epiglottis appeared to be main site of obstruction.
Weiss and Patwardhan124 administered lidocaine aerosols to 22 patients with stable asthma and demonstrated an initial decrease of expiratory gas flow of approximately 20% within 5 minutes of aerosol administration. Following this initial response, 12 of 22 patients continued to demonstrate a reduction in measured expiratory gas flow that persisted up to 60 minutes, whereas the remaining 10 patients revealed a significant improvement in expiratory gas flow above baseline.
McAlpine and Thomson125 similarly measured FEV1 in 20 asthmatic patients following the administration of 6 mL of 4% nebulized lidocaine. The maximum percentage change in FEV1 following lidocaine inhalation varied from −42.1% to +28.2%, with a mean of −8.2%. Five of the 20 patients experienced a decrease in FEV1 greater than 15%.
Groeben et al.126 measured changes in FEV1 in 10 volunteers with mild asthma after topical airway anesthesia with either lidocaine or dyclonine and awake bronchoscopic intubation on four separate study days. The local anesthetic was initially administered by nebulizer and supplemented with a gargle and administration of the anesthetic solution onto the epiglottis via the bronchoscope. In random order and double-blind fashion, each subject inhaled nebulized lidocaine or dyclonine preceded by salbutamol or saline. Following baseline measurements, FEV1 was measured after saline or salbutamol inhalation, local anesthetic inhalation, intubation, and extubation. Salbutamol inhalation significantly increased FEV1 as compared to saline. No significant change was noted after saline inhalation. No significant difference was found in FEV1 following lidocaine or dyclonine inhalation. Following awake bronchoscopic intubation after lidocaine anesthesia, FEV1 decreased 35%, and after dyclonine 51%. This decrease in FEV1 was significantly attenuated by salbutamol pretreatment in both groups. Two to 5 minutes after extubation, FEV1 returned to values close to those obtained following saline or salbutamol administration. No significant difference was found between FEV1 values after extubation as compared to the respective FEV1 baseline.
In 2006, Ho et al.127 reported a prospective observational study of the effect of upper airway topical anesthesia on dynamic airflow. Six healthy volunteers, all authors of the study, underwent a series of spirometric measurements before and after topical anesthesia of the upper airway produced by topical lidocaine using an aspiration technique (see section “How Is the Local Anesthetic Aspiration Performed? How Well Does It Work?” in this chapter). Peak inspiratory flow rate (PIFR), FIF between 25% and 75% of maximum inhaled volume, FEV1, and FVC were measured before the administration of lidocaine, immediately afterward, and at 10, 20, and 30 minutes afterward. A significant reduction in PIFR and FIF25–75 was demonstrated at all time points following the administration of lidocaine. Expiratory flow parameters were not affected. The authors concluded that maximum inspiratory flow is impeded by topical anesthesia of the upper airway and they suggested that caution be exercised in the setting of preexisting airway obstruction.
Thomson128 also reported a fall in specific conductance of the airway following bupivacaine aerosol administration to asthmatics.
The absence of control groups in most of these studies confounds interpretation to some extent, although the study by Groben et al. did not demonstrate a change in airway resistance after administration of saline aerosol. The effect of the temperature of the inhaled anesthetics is also uncertain. However, based on the available evidence it does appear that lidocaine anesthesia of the upper airway can cause airway obstruction.
Case reports of airway obstruction following topical anesthesia and instrumentation of the airway have also been published:
Shaw et al.129 reported a case of respiratory distress following the administration of 10% lidocaine spray to the tongue and oropharynx in the presence of a compromised airway associated with goiter. Air entry could not be maintained despite repositioning the patient onto her left side, jaw thrust, chin lift, and placement of an oral airway. Ventilation was restored via an LMA and a surgical airway performed. The authors speculated that a combination of laryngospasm due to irritation caused by the lidocaine spray, and loss of muscle tone as a result of the local anesthetic action, contributed to the airway obstruction.
McGuire and El-Beheiry130 reported complete airway obstruction during attempted awake bronchoscopic intubation under local anesthesia in two patients with unstable cervical spine fractures who were immobilized in a halo with traction. Both required surgical airways. Both patients also received sedative agents. One patient developed stridor then complete airway obstruction following introduction of the endoscope after topicalization using 1% lidocaine spray and cricothyroid puncture. The second patient was topicalized using swabs soaked in 4% lidocaine. Insertion of the endoscope was associated with gagging and coughing followed by complete airway obstruction.
Ho et al.131 reported a case of complete airway obstruction following instillation of 2% lidocaine onto the tongue and pharynx and suctioning prior to a planned awake intubation in a patient scheduled for excision of recurrent carcinoma of the neck. The patient was hoarse and had stridor preoperatively. He had previously undergone radical neck dissection, tracheostomy, and radiotherapy. Face-mask-ventilation was difficult and an attempted percutaneous cricothyrotomy failed. Flexible bronchoscopic intubation was successful and a tracheostomy was performed after the surgery was completed.
In 2015 White et al.132 reported the occurrence of airway obstruction immediately following topicalization in preparation for awake bronchoscopic intubation in a patient with a cervical spine fracture who was in halo immobilization. The patient lost consciousness, face-mask-ventilation was inadequate, and attempted flexible bronchoscopic intubation failed. Following release of the halo immobilization face-mask-ventilation was successful and intubation by VL was performed. The authors felt that cervical spine flexion in halo fixation, topical anesthesia, and sedation contributed to the airway obstruction.
In summary, extensive clinical experience with lidocaine has shown it to be an effective topical agent for airway anesthesia and to have a wide margin of safety.86,105 However, in the presence of preexisting airway compromise, topical anesthesia and instrumentation of the airway can be associated with complete airway obstruction, and in this setting due consideration must be given to the performance of an awake tracheotomy under local anesthesia.131,133 If awake intubation is undertaken in the setting of acute upper airway obstruction, then the practitioner must be prepared to perform a surgical airway should total obstruction occur (i.e., a “double set up”) in which case the CTM and anticipated incision line are marked, the necessary equipment made ready, and the area prepared in a sterile fashion. Communication of this plan to the assembled health care team is vitally important (see section “What Technique Works Well for Awake Intubation?” in this chapter).
Tetracaine (pontocaine), a long-acting amino ester derivative of para-aminobenzoic acid (PABA), was introduced in 1932 and is still used for spinal anesthesia and topical anesthesia of the eye.45,54 It is hydrolyzed by plasma pseudocholinesterase to p-aminobenzoic acid134 and diethylaminoethanol135 and has the slowest rate of hydrolysis of the ester type local anesthetics.134 The presence of an atypical pseudocholinesterase is associated with decreased metabolism.42 Although once widely used for topical anesthesia of the airway,136 its use for this indication fell into disfavor after reports of toxic reactions including fatalities were published in the 1950s.61,136 Of the local anesthetics possessing topical action, dibucaine and tetracaine are the most potent as well as the most toxic,61 tetracaine being about six times more toxic than cocaine.54 The maximal effective concentration of topical tetracaine is 1%.47 This concentration has a latent period of 0.6 to 1.1 minutes and a duration of 50.2 to 55.5 minutes.47 When applied to the tongue, 0.5% tetracaine has a latent period of 1.6 minutes and a duration of action of 18.1 minutes.47 The latent period for 0.4% tetracaine is 3.8 minutes and the duration of action is 35.8 minutes.47 The duration of action on the conjunctiva is approximately twice than that at the tip of the tongue, whereas the duration of action on the lip and palate is intermediate. Tetracaine appears to be superior to other topical anesthetics and this may be due to its ability to anesthetize structures deep to the mucous membrane.61,137
Tetracaine applied to the mucous membranes of the pharynx and trachea is rapidly absorbed into the circulation such that blood levels are almost comparable to those obtained after IV injection.137,138 Absorption has been shown to be a function of the dose of the drug administered and not the concentration of the solution utilized.138 Epinephrine added to the tetracaine does not retard its absorption.137,138 In 1951, Weisel and Tella136 reported a series of 1000 bronchoscopies performed with topical tetracaine. There were 12 minor and 7 severe toxic reactions including 6 seizures and 1 severe bronchospasm. Loss of consciousness preceded convulsions in two of the cases. Cotton pledgets were dipped into a solution of 2% tetracaine and placed successively between the faucial pillars and in each piriform fossa for about 1 minute at each location. Then 1 mL of 2% tetracaine was injected into the trachea using a syringe and a laryngeal cannula.136 The dose administered was estimated to be ≤40 mg in most cases although measurement was inexact. The toxic reaction occurred following application of the fourth pledget in two patients, and tracheal instillation in five patients, and was heralded by syncope or presyncope.136 Adriani and Campbell61 noted 10 fatalities at their institution over a 15-year period caused by topical tetracaine and were aware of 5 others “under similar circumstances.” In 1989, Patel et al.139 reported three cases of toxicity associated with the use of topical tetracaine for esophagogastroduodenoscopy, one of which was fatal. Two patients gargled approximately 4 mL and the other 4 to 5 mL of 0.5% solution. Within minutes one patient complained of dizziness, then had a grand mal seizure followed by cardiac arrest with no ventricular activity. The second patient complained of dizziness within 2 to 3 minutes of gargling 4 to 5 mL, developed slurred speech and diplopia, and then became unresponsive to voice and experienced facial weakness and right hand tonic clonic movements. The episode resolved within 15 to 20 minutes. The third patient complained of dizziness after gargling and cough, developed slurred speech, and then became unresponsive to voice. The episode resolved within 30 minutes. Although the manifestations of systemic toxicity with tetracaine may be similar to those of other local anesthetics, excitement may be absent and lethargy can rapidly progress to coma and cardiorespiratory arrest.139 The maximum safe dosage of tetracaine has never been clearly defined.137 However, maximum safe doses cited in the literature are said to be 50 mg31,45 or 100 mg140,141 in the adult. A maximum dose of 20 mg of tetracaine hydrochloride has also been recommended.61,139,142 Again, when maximum safe doses are considered, the method of administration must also be taken into account. In a 1995 review, topical tetracaine was said to be no longer recommended for topical airway anesthesia because of its narrow margin of safety.143 In 2000 Colt and Matsuo144 reported a series of 1009 flexible bronchoscopies performed “for the most part” using no more than 42 mL of 0.45% topical tetracaine with epinephrine. There were 50 adverse events including two seizures. In 2009, Davis and Channick145 reported a series of 537 flexible bronchoscopies performed on 431 patients. Four hundred and thirty four bronchoscopies were performed using only topical anesthesia with no sedation. A 0.45% tetracaine solution administered by atomization and by injection via the bronchoscope was used in 99.6% of the procedures. The mean dose of tetracaine administered was 120 mg (range 18–158 mg) and 91% of the procedures were performed with more than 100 mg of tetracaine. There were 23 adverse reactions but no neurologic events occurred. There were four arrhythmias, three of which were sinus tachycardia and one case of bigeminy all of which resolved spontaneously. The authors noted that tetracaine was used by 3% of bronchoscopists in North America.145
Allergic reaction, although rare, is more likely with the ester group of local anesthetics as compared to the amides. As of 2007, a single case of methemoglobinemia had been reported associated with tetracaine.74
In summary, tetracaine (0.45%) administered by atomizer produces excellent intubating conditions, however because of the potential for severe toxicity its use cannot be recommended particularly when a much safer and equally effective alternative (lidocaine) is available.
Cocaine (benzoylmethylecgonine) is an ester of benzoic acid and methylecgonine which is extracted from the leaves of the coca shrub, Erythroxylum coca.38,146–148 It was first isolated in 1860, serendipitously discovered to have anesthetic properties, and introduced into clinical medicine as a topical anesthetic in 1884.45,54,147,149 It is the only local anesthetic that inhibits reuptake of norepinephrine and thereby produces vasoconstriction, hence its widespread use in the past for nasal procedures.54,146,150–152 Cocaine also blocks the reuptake of dopamine, serotonin, and norepinephrine in central nervous system neurons producing psychostimulant effects.38 In addition, it causes the release of norepinephrine and epinephrine from the adrenal medulla.147 Cocaine is thus a powerful sympathomimetic.147 Antagonism of voltage sodium channels in cardiomyocytes can also occur.38 Cocaine is well absorbed through all mucous membranes.147,153 Peak plasma concentrations occur 30 to 60 minutes after nasal application.146 Blood levels after topical application to the piriform fossae were similar to levels produced after IV injection,54,138 and toxic systemic levels (>0.9 mg·L−1) have been attained following intratracheal injection of 5 mL 2.5% cocaine solution.154 Cocaine is metabolized by plasma and liver cholinesterases to water-soluble metabolites which are excreted in the urine.153 The serum half-life is 25 to 90 minutes.153
The maximum effective concentration of topical cocaine is 20%, and this solution produces an anesthetic effect within 0.3 minutes and has a duration of action of 54.5 minutes.47 Topical anesthesia produced with 10% cocaine has a latent period of 2 minutes and a duration of action of 31.5 minutes, whereas 4% cocaine has a latent period of 4 minutes and a duration of action of 10.2 minutes.47 The same degree of blockade is produced with 20% cocaine as with 1% tetracaine.47 Typically 1% to 10% cocaine is used clinically.45,79 The vasoconstriction produced by cocaine occurs after a latent period of 5 to 10 minutes.75 The maximum recommended dose for topical nasal application has been said to be from 1.5 mg·kg−1 155 to 3.0 mg·kg−1 50 and 1 to 3 mg·kg−1;156,157 however, toxic reactions have occurred after administration of as little as 10 to 30 mg,148,151,156,158 and adverse reactions are not clearly dose related.148,151 The addition of epinephrine has been shown to reduce cocaine absorption150 but its effect is not consistent.146,159 Cocaine has been associated with hypertension,155 coronary artery spasm, angina, myocardial infarction, arrhythmias, sudden cardiac death, and myocardial contraction bands.154 The first report of myocardial infarction temporally related to the recreational use of cocaine appeared in 1982 and subsequently multiple cases of cocaine-induced myocardial infarction have been identified.160 There have also been at least 10 case reports of significant cardiotoxicity associated with the clinical use of cocaine.154,159,161–168 The principal cardiac effects of cocaine include increased myocardial oxygen demand, coronary vasoconstriction, and endothelial dysfunction which predisposes to vasoconstriction and thrombosis.154 Platelet activation can also occur.169 Doses as small as 0.4 mg·kg−1 may cause ventricular fibrillation.54,155 A survey of otolaryngoscopists published in 2004 reported 14 deaths associated with the administration of cocaine,151 and this was compared to a similar survey published in 1977 which had reported 15 deaths.152 The use of topical cocaine without complications was reported in a series of 92 bronchoscopies in 2010.149 In 1956 Tella and Weisel170 reported a series of 1000 diagnostic bronchoscopies in which topical cocaine was used. In this series there were eight cases of hypotension associated with altered or loss or consciousness. One of these patients also had clonic convulsions. One additional patient experienced loss of consciousness and clonic convulsions but no hypotension.170
It has been stated that cocaine should be avoided or used cautiously in the presence of hypertension, hyperthyroidism, angina, or in patients taking monoamineoxidase inhibitors (MAOIs).50
Oxymetazoline has been shown to be as effective as cocaine in the prevention of epistaxis caused by nasotracheal intubation,171,172 as has normal saline,172 phenylephrine/lidocaine,13,158 and phenylephrine alone.158 A 1995 study by Tarver et al.173 which compared cocaine and lidocaine with oxymetazoline for nasal procedures found no difference in local anesthetic efficacy. Cocaine is listed as a schedule II controlled substance by the United States Drug Enforcement Agency because of its abuse potential.38
Based on the available evidence, the disadvantages associated with the use of cocaine to produce nasal anesthesia for awake intubation appear to far outweigh any potential advantage.
Benzocaine (ethyl aminobenzoate), an ethyl ester of PABA,38,142 is a poorly water-soluble ester type local anesthetic that has been widely used for topicalization of the airway.38,141 Following topical application most of the benzocaine is acetylated by N-acetyltransferases with a small amount being converted to PABA.174 Genetic polymorphism accounts for some slow acetylators.174 Despite its lipophilicity and rapid acetylation, measurable amounts of benzocaine are absorbed into the circulation174 where a portion undergoes ester hydrolysis by serum pseudocholinesterase to PABA and ethanol.174,175 Some benzocaine is available for hepatic biotransformation metabolism.174 The metabolite N-hydroxy PABA (N-OH-PABA) has been identified and is capable of oxidizing hemoglobin to methemoglobin.174 Polymorphism may affect benzocaine metabolism, producing some rapid metabolizers and it seems highly likely that a genetic susceptibility to the generation of N-OH-PABA exists.174
Benzocaine is available as a 20% spray which can deliver between 60 mg140 and 200 to 295 mg per 1-second spray.142 It is also a component of Cetacaine® which consists of 14% benzocaine, 2% tetracaine, and 2% butyl aminobenzoate (butamben).176 The maximum effective concentration of topical benzocaine is 20%, has a latent period of 0.17 minutes, and a duration of action of 4.3 minutes.47 An onset time of 15 to 30 seconds and a duration of action of 5 to 10 minutes have also been cited.143 A double-blind placebo-controlled study which compared the clinical effectiveness of topical lidocaine and benzocaine determined that the two drugs were equally effective in reducing pain produced by needle insertion into the palate.177 The maximum dose recommended for upper airway anesthesia has been quoted to be 1.5 mg·kg−1,50 although a dose of 100 mg in the adult has also been cited to be toxic.141
Benzocaine can produce methemoglobinemia following the administration of as little as 150 to 300 mg in the adult.178 Methemoglobinemia associated with the use of benzocaine has been reported since the 1950s.179,180 A literature review up to April 2007 identified 159 episodes of methemoglobinemia associated with the use of benzocaine.74 Of these, benzocaine had been used alone in 105 episodes. Multiple additional series and cases have been reported since that time.174,175,181–188 The incidence of benzocaine-induced methemoglobinemia has been estimated to be 0.115% (maximum 95% confidence interval for incidence 1/370 exposures and 1/28 for repeat exposure within 1 week),189 0.025%,183 0.45%,175 and 1 per 7000 exposures.190 The occurrence of benzocaine-induced methemoglobinemia appears to be unpredictable, idiosyncratic, and not directly related to dose,183,184,191 although those with active systemic infection175 and primary relatives of those who have experienced this reaction may be at increased risk.174 A letter of warning has been issued by the Federal Drug Administration (FDA) in the United States192 and the Veterans Administration in the United States has removed benzocaine from its formulary.175
Methemoglobinemia is potentially fatal176,193 and treatment with a 1% solution of methylene blue 1 to 2 mg·kg−1 has been recommended for methemoglobin levels greater than or equal to 30% or at lower levels if symptoms of hypoxia are present (e.g., dyspnea, angina, confusion, etc.).194 However, methylene blue is not the drug of choice in patients with glucose-6-phosphate dehydrogenase (G6PD) deficiency in whom it would not work or elicit a poor response.74,189 Methylene blue is reduced to leukomethylene blue by nicotine adenine dinucleotide phosphate diaphorase and adenine dinucleotide phosphate is dependent on G6PD activity.74,195 Leukomethylene blue reduces methemoglobinemia to deoxyhemoglobin.74 Ascorbic acid is the preferred treatment in patients with G6PD deficiency.74 Normal levels of methemoglobin (about 1% of total hemoglobin concentration,175 <1.5% to 2%,189 1% to 2%74) should be achieved within 20 minutes to 1 hour following treatment with methylene blue.196 However, repeat doses of methylene blue may be necessary.196 The maximum total dose recommended is 4 mg·kg−1 197 to 7 mg·kg−1.196 Methylene blue can induce hemolysis at higher doses183,187 and at clinical doses in patients with G6PD deficiency.74 Rebound methemoglobinemia has been reported 2.5 to 20 hours after methylene blue administration.74,198 If there is a poor response to methylene blue, or if methemoglobinemia is severe (levels >70%) exchange transfusion or dialysis may be required.189 Although methemoglobinemia has been reported with the use of topical lidocaine it is rare74,183; one large series reported that benzocaine containing topical anesthetics were 3.7 times more likely to be associated with methemoglobinemia.183 The benzocaine metabolite, PABA, is a highly allergenic molecule79 and allergic reactions to benzocaine can occur.
In her 2009 review of methemoglobinemia related to local anesthetics, Dr. Guay74 recommended that benzocaine should no longer be used. Other authors have also recommended that the use of benzocaine be avoided.175
Given its short duration of action, and its potential for severe toxicity, the use of benzocaine as a topical anesthetic for airway management is difficult to justify, especially when a much safer and equally effective alternative (lidocaine) is available.
Cetacaine® is a topical anesthetic spray that contains benzocaine 14%, butamben 2%, and tetracaine hydrochloride 2%. According to the manufacturer, the onset time of local anesthesia is 30 to 60 seconds and the duration is typically 30 to 60 minutes (Cetylite Industries, Inc. Cetacaine Spray product Sheet. Cited December 15, 2009. Available at: http://www.cetylite.com/cetacaine spray.html). The manufacturer recommends that cetacaine be applied for approximately 1 second or less for normal anesthesia. A spray in excess of 2 seconds is considered to be contraindicated. The manufacturer states that “dosages should be reduced in the debilitated elderly, acutely ill, and very young patients.” The average expulsion rate from the spray is 200 mg s−1 at normal temperatures. Each 200 mg of cetacaine contains 28 mg of benzocaine, 4 mg of butamben, and 4 mg of tetracaine. Hypersensitivity reactions can occur and there have been multiple case reports of methemoglobinemia associated with the use of cetacaine.183,186,190,193,196,199–206
As with benzocaine and tetracaine, the use of Cetacaine® for airway anesthesia cannot be recommended by this author.
Dyclonine, a ketone, is a unique local anesthetic agent that was introduced in 1952 and is structurally distinct from the aminoesters and aminoamides.207 It can be used as a 0.5% to 1% solution for topical airway anesthesia.45,207 When applied to mucous membranes, the onset time is 2 to 10 minutes and the duration of action is 20 to 30 minutes. Adriani et al.47 noted that dyclonine had limited systemic toxicity but a saturated solution may cause residual numbness that persists for many hours suggesting local neural injury. One percent dyclonine administered by aerosol has been shown to produce topical airway anesthesia as effective as, and longer lasting than, 4% lidocaine.126 In a study of 10 volunteers with mild asthma, 4 of the 10 subjects reported much more intense topical anesthesia following 1% dyclonine inhalation as compared to 4% lidocaine.126 However, FEV1 decreased to a greater extent in the dyclonine group and the authors concluded that dyclonine must be considered relatively contraindicated in the setting of bronchial hyperreactivity.
Bacon et al.207 reported the use of dyclonine for awake bronchoscopic intubation in a patient with apparent allergy to local anesthetics. The patient gargled and then swallowed 25 mL of 1% dyclonine solution, and 5 mL of 1% dyclonine was then administered by nebulizer. Adequate anesthesia was achieved.207 Dyclonine has not been widely used for airway anesthesia and is no longer marketed for this purpose in the United States or Canada.
Ropivacaine, an amino ethylamide, is a long acting S-enantiomer amide local anesthetic with an efficacy similar to bupivacaine.208 It appears to be less cardiotoxic than equieffective doses of bupivacaine and is slightly less potent.38 When applied topically to the oral mucosa, 1% ropivacaine produced anesthesia similar to benzocaine and EMLA209,210 and topical ropivacaine has been shown to be effective in cataract surgery.211 When sprayed onto the larynx, epiglottic area, and trachea after general anesthesia induction, topical ropivacaine has been shown to effectively reduce the hemodynamic response to intubation.212 Topical 0.5% ropivacaine was found to be less effective than 10% lidocaine in attenuating the discomfort associated with transnasal flexible nasopharygolaryngoscopy.213 In a study of histamine-induced bronchospasm by Groben et al.,214 1% ropivacaine administered by nebulizer produced anesthesia similar to that produced by 4% lidocaine as tested by touching the uvula and posterior pharyngeal wall with a cotton swab. The dose of ropivacaine administered was 0.5 mg·kg−1 and the peak plasma concentration was 0.32 ± 0.09 µg·mL−1 (toxic concentration 0.9 µg·mL−1). The duration of the topical anesthesia was similar to that of 4% lidocaine. Initial bronchoconstriction with a significant decrease in FEV1 was noted after inhalation of ropivacaine but not with lidocaine. Both drugs attenuated histamine-induced bronchospasm.214 No reports of the use of topical ropivacaine for awake intubation were found in the literature search on this topic.
Of the topical anesthetics available for awake intubation lidocaine would seem to have the most favorable risk benefit ratio.
Awake flexible bronchoscopic intubation requires profound regional anesthesia of the pharynx, larynx, and trachea if it is to be performed rapidly with minimal patient discomfort. If the nasal route is chosen, then regional anesthesia of the nasal cavity is required as well. Awake video-laryngoscopic or optical stylet intubation similarly requires profound anesthesia of the pharynx, larynx, and trachea if it is to be performed smoothly with minimal patient discomfort. Stimulation of pressure receptors at the base of the tongue by the video-laryngoscope may be difficult to avoid and without profound anesthesia of the larynx, endotracheal tube contact with the laryngeal mucosa will provoke reflex glottic closure. The choice between these awake intubation techniques is largely a matter of personal preference and experience.
A wide variety of techniques for regional anesthesia of the airway have been described as summarized below. Topical anesthesia is currently more widely used than injection techniques. The technique which the author recommends for airway topicalization is summarized in the section “What Technique Works Well for Awake Intubation?” of this chapter.
Awake intubation by direct laryngoscopy requires less profound regional anesthesia of the airway. The curved blade should be used for awake direct laryngoscopic intubation as it can be advanced into the vallecula with minimal patient discomfort and avoids contact with the larynx. The tube is passed through the glottis under direct vision and contact with the laryngeal mucosa (at the vocal cords) is brief. One of the great advantages of awake intubation by direct laryngoscopy is that regional anesthesia of the larynx and trachea is not required. However, anesthesia of the posterior third of the tongue, the free edge of the soft palate, the tonsillar pillars, and the adjacent oropharynx (innervated by the glossopharyngeal nerve) will attenuate the gag reflex and facilitate awake intubation by direct laryngoscopy. This limited topical anesthesia technique can also be used to perform an “awake look.” In general awake intubation by direct laryngoscopy is restricted to the emergency setting with favorable airway anatomy. The most important prerequisite for a successful awake intubation is adequate regional anesthesia of the airway.
The posterior third of the tongue, the free edge of the soft palate, the tonsillar pillars, and the adjacent oropharynx can be sequentially anesthetized using commercially available 10% lidocaine spray (Lidodan™ Endotracheal),215–217 simply by directing the spray onto the relevant structures. A tongue depressor can be used to gently retract the tongue. This device is fitted with a metered valve that delivers 10 mg of aerosolized lidocaine with each depression of the pump mechanism,60 which uses manually compressed air as the driving force. Adequate regional anesthesia for awake intubation by direct laryngoscopy can readily be achieved in this manner, and in the emergency setting, time may not permit additional regional techniques. The 10% lidocaine aerosol is marketed in Canada by Odan Laboratories Limited, Montreal, Quebec, Canada, but is not currently available in the United States (see Figure 3–27). A search of the FDA website revealed that 10% lidocaine was listed as a discontinued product but no warning was found. Alternatively, 4% lidocaine administered using a mucosal atomization device (MAD) can be used (Wolf Tory Medical Incorporated, Salt Lake City, Utah). A curved metal cannula can also be used to inject lidocaine solution into the laryngopharynx and larynx as time and circumstances permit; however this is not necessary for awake intubation by direct laryngoscopy, may provoke laryngospasm and cough, and blood levels of lidocaine produced with this technique will probably be higher than those produced by aerosol techniques.57
Cooperative patients can also gargle 2% to 4% aqueous lidocaine to produce some topical anesthesia of the posterior tongue and adjacent oropharynx, although the author has not used this technique in over a decade due to the availability of better preparations and techniques such as 5% lidocaine ointment and atomized 4% lidocaine as described below. Residual anesthetic should be expectorated to avoid excessive drug exposure and potential nausea and vomiting, as with any technique.216–218
Lidocaine ointment (5%) is an extremely effective preparation with which to achieve anesthesia of the posterior third of the tongue. It is very useful as the first step in producing profound topical anesthesia for awake bronchoscopic intubation and can be used to facilitate awake intubation by direct laryngoscopy as time and circumstances permit. With any of these techniques, time (at least 131 to 2 minutes)47 and perhaps as long as 5 minutes45 must be allowed for the anesthetic effect to occur.
EMLA cream, a 1:1 eutectic mixture of 2.5% lidocaine and 2.5% prilocaine, has also been used to produce airway anesthesia. Larijani et al.219 applied up to 4 g of EMLA cream to the tongue and pharynx in a series of 20 patients who underwent awake bronchoscopic intubation. The intubation was performed via a Williams airway. The mean time from the application of the EMLA cream to placement of the oral airway was 11 ± 6 minutes. All patients were successfully intubated but all coughed when the scope was passed into the trachea. No toxic plasma levels of lidocaine or prilocaine occurred. A statistically significant increase in methemoglobin levels occurred within 6 hours; however, these levels did not exceed normal values (1.5%).219 Sohmer et al.220 used 4 mL of EMLA cream applied to the tongue and gargled before performing awake bronchoscopy in 57 patients. In addition, 79.05±14.39 mg of lidocaine was administered through the flexible bronchoscope (FB) for laryngeal anesthesia. Fifty-six of the cases did not require supplemental anesthesia. Bronchoscopic conditions were excellent in 55 cases and good in the remaining 2 cases.220 The mean time from EMLA application to insertion of the bronchoscope was 5.10±0.45 minutes. EMLA cream applied to the nostril prior to the passage of an FB provoked rhinorrhea and sneezing that persisted for several hours in 21 of 31 individuals, although the endoscopy was well tolerated.221
EMLA cream may be an alternative for oropharyngeal topical anesthesia although experience is limited and the author does not recommend it at this time.
Excellent anesthesia of the airway can be produced by the administration of aerosolized local anesthetic delivered by an atomizer, such as the DeVilbiss RD 15 (see Figure 3–28). The device consists of a glass reservoir, which holds the anesthetic solution, and a nozzle assembly, which can be connected to a high-flow oxygen source by means of standard oxygen tubing. A small bleed hole is cut in the oxygen tubing at a convenient location near its connection to the atomizer. When oxygen flow is delivered into the tubing at about 8 L·min−1 occlusion of the bleed hole with a fingertip produces a fine spray of local anesthetic from the atomizer nozzle. At the author’s institution, the DeVilbiss atomizer has been used routinely for awake intubation for more than three decades with excellent results and no toxic reactions. An excellent block can be achieved with 10 to 12 mL of 3% or 4% lidocaine. For more than a year, the author and many of the faculty of the Difficult Airway Course: Anesthesia have been using the disposable EZ-Spray (Figure 3–29, EZ-100, Alcove Medical Corporation, Houston, Texas) and found the intensity of the block to be at least equivalent and perhaps even superior to that produced by the DeVilbiss. When using the Alcove EZ-Sprayer the volume of lidocaine used is also somewhat less, about 10 mL. At the author’s institution, the disposable Alcove has largely replaced the nondisposable DeVilbiss.
FIGURE 3–28.
DeVilbiss atomizer: The device consists of a glass reservoir (R), which holds the anesthetic solution, and a nozzle assembly, which can be connected to standard oxygen tubing. A small bleed hole (H) is cut in the oxygen tubing. When oxygen flow is delivered into the tubing at about 6.0 to 8.0 L·min−1, occlusion of the bleed hole with a finger produces a fine spray of local anesthetic from the atomizer nozzle (N).

The atomized lidocaine can be delivered to the pharynx, larynx, and trachea through the nasal cavity or through the mouth and both approaches are effective. Superior gas flow characteristics through the nose may deliver the anesthetic more efficiently to the pharynx, larynx, and trachea where the effect is required and sniffing through the nose reflexly abducts the vocal vords.46,217 The nozzle is held at the nostril and the spray directed posteriorly along the floor of the nose during a deep inspiration or during a sniff through the nose. The patient is instructed to breathe (or sniff) in through the nose and out through the mouth. Of course nasal obstruction precludes nasal administration of the atomized lidocaine. Alternatively, the atomized lidocaine can be delivered through the mouth. The tongue can occlude the oropharyngeal isthmus and limit the delivery of the anesthetic to the pharynx, although having the patient breathe deeply will usually create an adequate airspace between the uvula and the dorsum of the tongue. Gentle tongue traction can also be used to open the oropharyngeal isthmus and allow the atomized lidocaine to reach the pharynx. The goal is delivery of the anesthetic to the pharynx, larynx, and trachea. Anesthesia of the mouth cavity is not necessary. When the atomized lidocaine is delivered through the mouth it can be administered continuously or during the inspiratory phase of respiration. The patient is instructed to breathe deeply through the mouth. Both of these techniques produce profound anesthesia of the airway in about 5 minutes.46,217
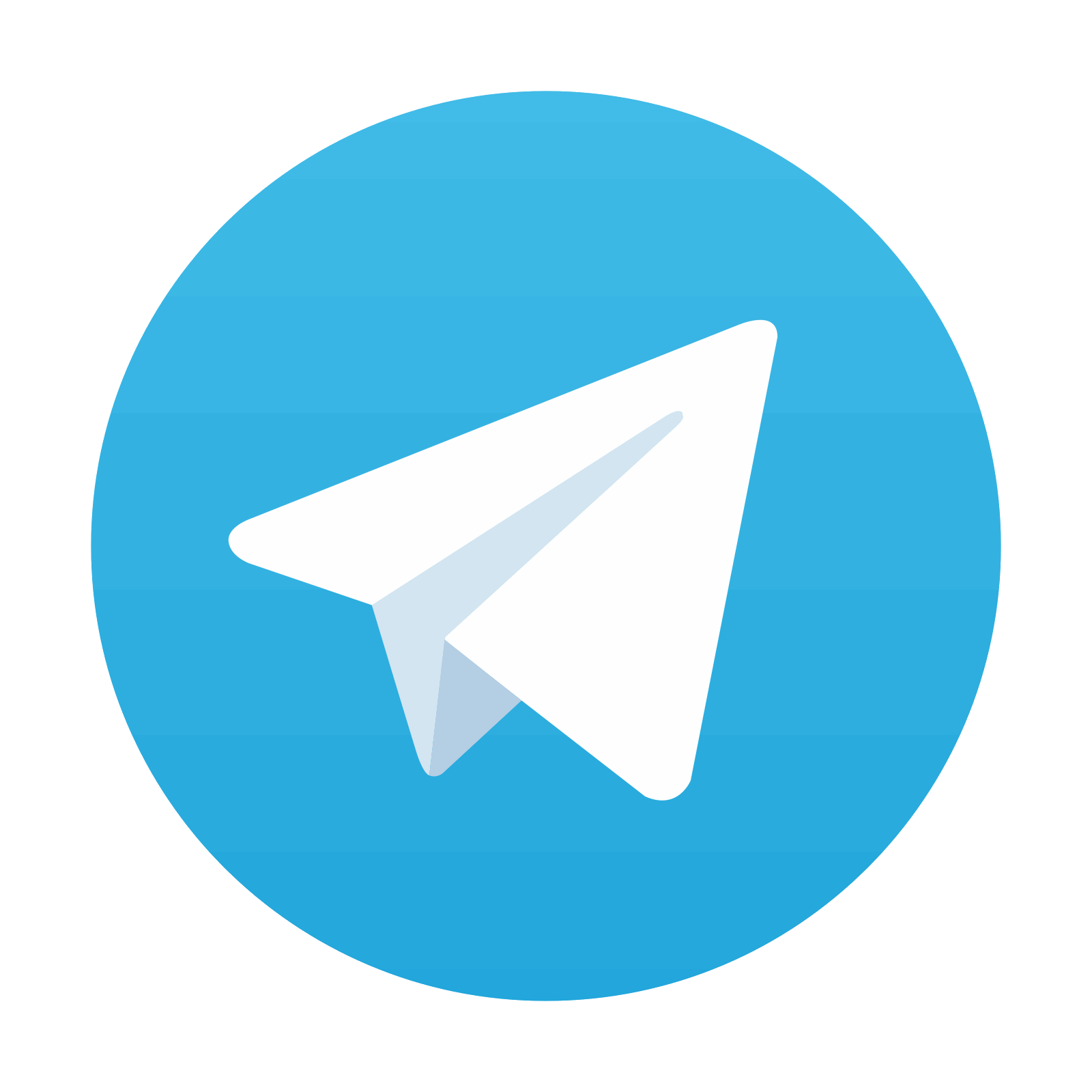
Stay updated, free articles. Join our Telegram channel

Full access? Get Clinical Tree
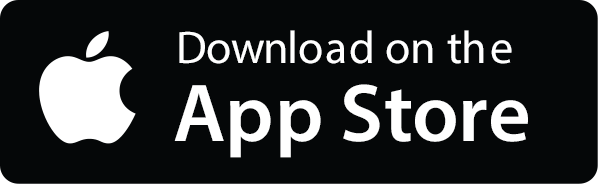
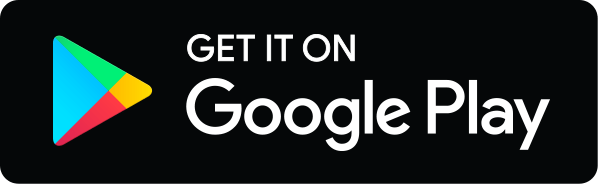