, Amy Gospel2, Andrew Griffiths3 and Jeremy Henning4
(1)
Intensive Care Unit, James Cook University Hospital, Middlesbrough, UK
(2)
Tyne and Wear, UK
(3)
The York Hospital, Middlesbrough, UK
(4)
James Cook University Hospital, Middlesbrough, UK
By the end of this chapter you will be able to:
List the six P’s of PRSI
Discuss each of the P’s in turn
Appreciate the importance of a plan B
3.1 The Six P’s (Fig. 3.1)

Figure 3.1
The 6 P’s
PRSI can be separated into six phases:
1.
Preoxygenation
2.
Preparation
3.
Premedication
4.
Paralysis and Sedation
5.
Passage of the Endotracheal tube
6.
Post intubation care
It is important that each member of the team is familiar with all phases of PRSI. This allows preparation to take place concurrently with ongoing patient management.
3.2 Preoxygenation
This is an essential phase in the conduct of safe PRSI. It provides an oxygen safety buffer to prevent precipitous desaturation occurring in the period of hypoventilation and apnoea, prior to and during laryngoscopy. Desaturation below an oxygen saturation (SpO2) of 70 %, places patients at risk of cardiovascular instability, hypoxic organ damage and death (Mort 2004).
Desaturation is a significant complication of PRSI. The Scottish Emergency Medical Retrieval Service found that 15.4 % (32 of 208 patients) had a desaturation episode during the procedure (below 90 % or a reduction in >10 % from initial SpO2) (Wimalasena et al. 2014).
The aim of preoxygenation is to replace the air in the alveoli with an enriched oxygen mixture, particularly in the functional residual capacity of the lungs. This will act as a reservoir for the body during apnoea. In ideal conditions this offers several minutes before desaturation occurs (Fig. 3.2, Box 3.1). Unfortunately, conditions for PRSI are rarely ideal, and critically ill patients with increased metabolic demand, hypovolaemia, reduced cardiac output and possible lung injury will desaturate far quicker than this.


Figure 3.2
Desaturation curves after full pre-oxygenation (Fraction Alveolar Oxygen = 0.87) (Benumof et al. 1997)
Box 3.1: Approximate Time for Desaturation to 90 % After Full Preoxygenation (Benumof et al. 1997)
Normal 70 kg adult = 8 min
Moderately ill 70 kg adult = 4.8 min
Normal 10 kg child = 3.4 min
Obese 127 kg adult = 2.6 min
3.2.1 Preoxygenation Strategies
Preoxygenation should be performed using a tight-fitting face mask with a reservoir and ≥15 L/min of oxygen in order to provide a high (>0.9) fraction of inspired oxygen (FiO2) during inspiration. In hospital this is done using either a standard anaesthetic circuit or a disposable Mapleson C (sometimes called a ‘Waters’ circuit) with adjustable pressure limiting (APL) valve (Fig. 3.3). This also allows an element of continuous positive airway pressure (CPAP) to be applied, as long as the face mask is tight-fitting. These systems require a constant high flow of oxygen and a tight seal to allow manual ventilation, neither of which can be guaranteed in the pre-hospital setting. The default system in the emergency situation is a self-inflating bag-valve-mask (BVM), of which there are many varieties, including many single-use versions. Some older versions of BVMs did not have one-way exhalation valves near the mask and therefore allowed entrainment of air into the face mask at lower oxygen flow rates. These would deliver relatively low FiO2 (<0.4) during spontaneous ventilation (Mills et al. 1991; Nimmagadda et al. 2000). This is not the case with the majority of BVM systems currently in use, however, it is always important to check the type of BVM being used.


Figure 3.3
Mapleson C circuit With adjustable pressure limiting (APL) valve being used to apply CPAP
Even when a BVM has a one-way exhalation valve, there is variation in the FiO2 delivered by different BVM systems in common use. As an example, two single-use BVMs with exactly 15 L/min of oxygen attached, were compared for both ease of breathing and delivered FiO2 (using a calibrated oxygen analyser in a t-piece behind the face mask).
The Ambu Single Patient Use Resuscitator (SPUR) II (Fig. 3.4a) has an extremely low resistance inspiratory/expiratory single-shutter (one-way) valve at the patient end (Fig. 3.4b) which provided only minimal resistance to breathing. The valve prevents entrainment of air during inhalation, so only oxygen from the bag is inhaled if the mask is correctly sealed on the patient’s face. Even during large inspiratory breaths, the FiO2 remained above 0.9 at all times and at normal tidal volumes was 0.95. The large safety inspiratory valve at the reservoir bag end of the BVM assembly (Fig. 3.4c) is designed to allow air to be entrained if the reservoir bag is completely empty, to prevent asphyxiation. This only opened a little even during large tidal volumes; hence the FiO2 only dropped slightly due to indrawn air mixing with the oxygen.


Figure 3.4
(a) Ambu SPUR II, (b) Single Shutter inspiratory/expiratory valve. (c) Inspiratory safety valve
The Marshall Classic Manual Resuscitator (Fig. 3.5a) has a Laerdal type ‘duck-billed’ inspiratory valve and a flat silicone ring one-way expiratory valve at the patient end (Fig. 3.5b). The ‘duck-billed’ valve is subjectively more difficult to breathe through. The additional negative pressure generated, along with the small size and lightweight safety inspiratory valve (Fig. 3.5c), means that air is entrained through the valve even during relatively small volume breaths. This reduced the measured FiO2 from 0.9 at normal tidal volume down to 0.75 during larger inspiratory breaths. It is useful to know that both the ease of breathing and the inspired FiO2 can be improved significantly by gently squeezing the bag in time with the patient’s inspiration.


Figure 3.5
(a) Marshall Classic Resuscitator, (b) ‘Duck-billed’ inspiratory valve. (c) Inspiratory safety valve
Even more important than the design of the valves is a good seal between the mask and the patient’s face. Gas will always take the path of least resistance. During inspiration with a poor seal, gas will be entrained around the edges of the mask rather than drawing from the oxygen in the bag and reservoir via the one-way inspiratory valve. The requirement for a good face seal also applies to disposable non-rebreathe reservoir masks; the path of least resistance is also around the edge of the mask rather than through the one-way valve (although the resistance through the valve does tend to be less than in a BVM).
With a good seal and a patient with reasonable inspiratory effort, a BVM with a reservoir bag and >15 L/min will provide a high FiO2 (>0.9) and avoids the requirement to change face mask during the RSI (Nimmagadda et al. 2000). It also means that ventilation can be easily assisted if inspiratory breaths are shallow or if SpO2 drops whilst waiting for the muscle relaxant to work.
BVM preoxygenation does, however, require a competent additional person to maintain the tight face seal whilst the team is preparing for RSI. It may be more practical (and also possibly less daunting for the patient), to use a well-fitting ‘non-rebreathe’ face mask with reservoir bag for preoxygenation. This is held in place with an elastic strap placed behind the head. Basic models are not ideal as they have a thin plastic edge that may not create a good seal, and an open hole in one side of the mask that allows air to be entrained if the oxygen supply fails (Fig. 3.6). Both of these factors can result in a relatively low FiO2 (0.6–0.7), even at very high oxygen flow rates. Newer rebreathe masks have softer cushioned edges, which improve the seal, and have no open holes in the sides of the mask, thereby increasing the chance of delivering an FiO2 closer to 0.9 whilst freeing up an extra person (Fig. 3.7). With additional nasal cannula oxygen, as described below, this can increase the FiO2 further (Fig. 3.8). Clearly if the patient requires assistance to maintain airway patency or needs assisted ventilation, this benefit of freeing a person is lost.




Figure 3.6
Basic non-rebreathe mask

Figure 3.7
Newer non-rebreathe mask

Figure 3.8
Non-rebreathe oxygen mask with additional nasal cannula in place for apnoeic oxygenation during PRSI
Three minutes of normal tidal volume breaths is acceptable preoxygenation for most patients. This preoxygenation can be improved by asking the patient to fully exhale and inhale (vital capacity breaths), although most patients in the pre-hospital setting are unable to co-operate with such a request. Analgesia prior to preoxygenation may improve the effectiveness by reducing pain from chest trauma and increasing inspiratory volumes.
In agitated patients it may be necessary to use sedation to facilitate preoxygenation. Small doses of Midazolam (1–2 mg) or Ketamine (10–40 mg) should be titrated to effect. Weingart et al (2015) reported the successful use of Ketamine 1 mg/kg (+/− further 0.5 mg/kg aliquots) to facilitate preoxygenation in agitated patients. This then allowed 3 min of pre-oxygenation, before full sedation and a muscle relaxant were given. Although based on a relatively small convenience sample of patients unable to tolerate preoxygenation due to delirium, they demonstrated the ability to increase mean pre-laryngoscopy SpO2 from 89.9 to 98.8 %, and reported no complications such as pre-muscle relaxant apnoea, peri-intubation emesis, cardiac arrest or death, from doing so. Preoxygenation was achieved with a high flow non-rebreathe oxygen mask, or non-invasive CPAP if SpO2 <95 %. They commented that facilitating preoxygenation in this manner avoids the risks of proceeding to RSI without an adequate oxygen reserve in the patient, and the potential risks of peri-intubation BVM ventilation that may be required to prevent desaturation; such as gastric insufflation and aspiration. They termed this ‘delayed sequence intubation’ although it is essentially just sedation to facilitate preoxygenation. This concept has been advocated on the PHA course for several years.
SpO2 will fall precipitously during the 45–60 s of apnoea whilst awaiting onset of muscle relaxation, if starting SpO2 is already low. In these patients, if already receiving oxygen through a BVM, it may be advisable to gently augment the patient’s own respiratory effort prior to induction in order to maintain a safe oxygen saturation throughout the procedure. CPAP can also be applied to improve oxygenation if it is possible to fit an external positive end expiratory pressure (PEEP) valve to the BVM.
If ventilation is continued following induction and apnoea, cricoid pressure will help to avoid the associated gastric insufflation and reduce the risk of aspiration, although this may make ventilation more difficult in some cases. BVM ventilations should aim to be delivered slowly with both low volume (6–7 mL/kg) and low rate, in order to avoid inspiratory pressures sufficient to overcome the lower oesophageal sphincter pressure.
There is evidence that head-up positioning delays the time to desaturation during apnoea (Lane et al. 2005; Ramkumar et al. 2011). It may also reduce the risk of passive regurgitation. This may be achieved by using a semi-recumbent position on a stretcher trolley or, in trauma patients requiring spinal immobilisation, a reverse-trendelenburg position, with the head of the spinal board/scoop stretcher/trolley raised 30° above the feet.
3.2.2 Apnoeic Oxygenation
Although described in the medical literature for over a century, the application of apnoeic oxygenation to pre-hospital airway management procedures is relatively recent (Weingart et al. 2011). It utilises the physiological principle that even without respiratory effort, approximately 250 mL/min oxygen is taken from the alveoli into the bloodstream. If oxygen is supplied to a patent upper airway, a mass flow of gas from the pharynx to alveoli maintains this diffusion of oxygen into the bloodstream, and in doing so, maintains oxygenation without ventilation. The Greater Sydney Helicopter Emergency Medical Service (HEMS) has included apnoeic oxygenation in their PRSI standard operating procedure (SOP) since 2011. During the preoxygenation phase, whilst a non-rebreather mask or BVM set at 15 L/min oxygen is used to preoxygenate, nasal cannulae are applied and set to 5 L/min oxygen, as tolerated (Fig. 3.8). After induction drugs are administered, the flow rate of the nasal cannulae is increased to 15 L/min until the endotracheal tube is secured (Wimalasena et al. 2015). During the 22-month period prior to apnoeic oxygenation, 22.6 % of patients had a desaturation episode below 93 % during RSI, compared to 16.5 % of patients in the 22-month period after introduction of apnoeic oxygenation to the SOP. They suggest this simple and inexpensive technique is of clear clinical benefit to critically ill and injured patients undergoing PRSI.
3.3 Preparation
Performance of a rapid sequence intubation is not immediate and requires time for preparation. The time spent in the preparation phase is never wasted and will increase the chances of a swift and safe intubation. There are four elements to preparation for PRSI (Box 3.2).
Box 3.2: Preparation
Preassessment
Prepare equipment and drugs
Position patient and team
Protect (c-spine and cricoid pressure)
3.3.1 Preassessment
Preassessment should be easily remembered with a familiar <C>ABCDE approach. It is a similar, but much more rapid assessment, to that which occurs before any anaesthetic in the hospital environment.
3.3.1.1 Catastrophic Haemorrhage
Catastrophic haemorrhage should be controlled at the earliest opportunity during pre-hospital management. This may involve use of wound packing and direct pressure, haemostatic agents and tourniquets. Reducing blood loss and promoting clotting at this earliest stage, not only is beneficial to the patient overall, but also promotes haemodynamic optimisation in preparation for PRSI. Splinting fractures of the pelvis and long bones (particularly femur) is important and will also minimise blood loss, but this occurs after assessment of the Circulation (Sect. 3.3.1.4).
3.3.1.2 Airway
The main concerns are to achieve maximum airway patency to allow preoxygenation prior to RSI, and to be able to predict the likelihood of a “difficult airway.”
Difficult Airway
The definition of a difficult airway is that a trained clinician experiences difficulties with mask ventilation, endotracheal intubation or both (American Society of Anesthesiologists (ASA) 2013). Difficult mask ventilation is defined as a situation when it is impossible to maintain SpO2 above 90 % or it is not possible to reverse signs of inadequate ventilation with a BVM and 100 % oxygen. The incidence of difficulty in airway maintenance in trauma patients can be up to 18 % (Smith and Dejoy 2001). The incidence of a difficult airway is likely to be higher in the pre-hospital environment, for reasons mentioned earlier. Airway assessment should therefore include a judgment of the likely ease of airway maintenance with a BVM, as well as the potential for difficult intubation. The anticipation of difficulty may require a change of the initial management plan (Box 3.3).
Box 3.3: Reasons for Difficulty in Maintaining an Airway
Obesity
The presence of a beard
The edentulous patient
Facial injury
Difficult Intubation
Difficult intubation is defined in many different ways in various studies, making comparative reporting difficult. The 2013 guidelines of the ASA divide difficult intubation into two parts: difficult laryngoscopy and difficult intubation. Difficult laryngoscopy is defined as being unable to visualise any portion of the vocal cords after multiple attempts at conventional laryngoscopy (i.e. Cormack-Lehane grade ≥3 (Fig. 3.20)), and difficult tracheal intubation as the requirement for multiple attempts. (This was previously described by the same group as more than 3 attempts or requiring more than 10 min with conventional laryngoscopy (ASA 1993)). Failed intubation is then described as failure to place the endotracheal tube after multiple attempts (ASA 2013). The widespread use of alternative devices that provide indirect views of the larynx (e.g. Airtraq™ etc.) adds further complexity to these definitions.
The Whatcom Medic One service reported a pre-hospital failed intubation rate of 3.4 % (2978 patients), although some intubated patients required three attempts (Wang et al. 2004). This is identical to the failure rate reported by Fakhry et al. (Fakhry et al. 2006), again a paramedic service allowing up to three attempts at intubation (175 patients). In 1993 London HEMS, a physician led service, reported a requirement for surgical cricothyroidotomy in 7.7 % of 143 patients requiring advanced airway management on scene (Xeropotamos et al. 1993). Since then PRSI training has much improved. The London HEMS data 1991–2012 presented by Lockey et al. (2014) included 7256 patients requiring advanced airway management. An immediate surgical airway was performed in 46 patients (0.6 %), and just 52 of 7210 patients (0.7 %) required a rescue technique for failed intubation.
Breckwoldt et al. (2011) looked to characterise factors contributing to difficult pre-hospital intubation (defined as more than 3 attempts or grade 3 or 4 view) in their physician led teams (2004–2005). In this series of 276 intubation attempts, 3 patients (1.1 %) required a fourth intubation attempt, and 4 patients (1.4 %) required rescue techniques for failed intubation. Difficult intubation (36 patients (13 %)), was significantly associated with short neck, body mass index (BMI) >30, anatomical abnormalities (micrognathia (small lower jaw), ankylosing spondylitis), mouth opening (<3 cm), neck/face trauma, laryngeal oedema, and limited space on scene. Unexpected difficult intubation occurred in 5 % of patients in whom physicians had predicted no difficulties.
In a series of 400 pre-hospital intubations by the physician-led trauma team in London (2007–2008), initial view at laryngoscopy was grade 3 in 12 % of patients and grade 4 in 5 % of patients, although it is not commented upon as to whether an improved view was subsequently achieved (Lockey et al. 2013). Physicians were asked to predict whether laryngoscopy was likely to be difficult, and this ‘quick look’ assessment generated sensitivity of 0.597 and specificity of 0.763, with positive predictive value of 0.336 and negative predictive value of 0.904, demonstrating some utility of this method, although not in isolation.
The Modified Mallampati classification of airway assessment (Fig. 3.9) is a commonly used tool in the hospital environment to predict difficult intubation (likelihood of difficulty increases from Class 1 to Class 4). Unfortunately it requires an awake and cooperative patient, so it is usually not useful in patients requiring pre-hospital RSI. Other predictors of difficult intubation may be present (Box 3.4). The ‘LEMON’ assessment tool, is one system commonly taught for emergency airway assessment which combines most of these factors into a mnemonic (Box 3.5).


Figure 3.9
Airway assessment – modified Mallampati classification. The view of the pharyngeal structures is observed, with the mouth open and tongue protruded maximally (Reproduced by kind permission Anaesthesia UK Website)
Box 3.4: Reasons for Difficult Intubation
Large/prominent upper incisors
Limited mouth opening
Reduced range of movement of the head/neck (MILS)
Reduced sterno-mental distance (“no-neck”), thyro-mental distance (“chinless”)
Swollen/oedematous tongue or laryngeal/pharyngeal tissue
Congenital abnormalities
Previous maxillo-facial surgery or radiotherapy
Box 3.5: LEMON Airway Assessment
Look externally (obesity, short wide neck, small mouth, beard, lower facial trauma, high arched palate, large prominent teeth)
Evaluate the 3:3:2 rule (3 cm mouth opening, 3 cm distance tip of chin to hyoid bone, 2 cm between hyoid bone and thyroid notch)
Mallampati score
Obstruction (stridor, foreign objects, pooling secretions, neck mass/swelling)
Neck mobility (immobilisation (MILS)/fixation, degenerative disease)
Clinical Signs of Potential Difficult Intubation or Ventilation
Obese Patients
Obese patients or those with short muscular necks can be difficult to intubate and ventilate. Excess soft tissue around the airway may hinder displacement during laryngoscopy. This may affect the view. These tissues may also collapse over laryngeal structures making manual ventilation difficult. Two-handed mask ventilation with an oropharyngeal airway may be required.
Edentulous Patients
Edentulous patients are often easy to intubate but can be difficult to manually ventilate. The lack of teeth leaves less support for the cheeks, and as a result the face mask seal may be poor. This can be remedied with a two-handed technique bunching the facial tissue up into the mask to achieve a seal. Again an oropharyngeal airway may make ventilation easier. Early intubation or use of a laryngeal mask airway (LMA) may be required if ventilation is inadequate.
“Chinless” Patients
Patients with micrognathia (receding chin) usually have an anterior larynx. There is a reduced thyromental distance: tip of chin to thyroid cartilage (Adam’s apple) of <6.5 cm with the neck extended. This measurement is therefore not practical when cervical spine injury is a concern. An impression of micrognathia should still be a visual clue. These patients do not have enough space to displace tissue forward to allow intubation. Laryngeal manipulation such as backward upward rightward pressure (BURP) and a bougie will often be required. (Note: Male patients often grow a beard to hide a small chin).
“No-Neck” Patients
A reduced sternomental distance (tip of chin to sternum <12.5 cm) is predictive of a difficult intubation. Again, this measurement is based on the head in an extended position and is therefore not usually practical. Subjective appearance may add to your assessment of difficulty.
Bearded Patients
As well as sometimes hiding micrognathia, a beard makes it difficult to achieve an adequate seal with a face mask to allow ventilation. Applying lubricating jelly to the beard and using a two-person technique will improve the situation. An LMA may be required to ventilate if intubation is not possible.
“Goofy” Patients
A prominent overbite (protruding upper teeth) can impede laryngoscopy. It may be difficult to manoeuvre the laryngoscope without levering on the upper teeth. External pressure on the larynx (above the cricoid cartilage) i.e. BURP, and use of a Macintosh No. 3 blade, inserted fully inside the mouth (past the top teeth), may help.
“Stiff-Neck” Patients
In patients with poor neck mobility, the larynx will, in effect, be more anterior. In patients with no risk of neck instability, elevating the head further to compensate for reduced extension of the head along with thyroid pressure and a bougie, gives the best chance of success. If there is a chance of underlying instability and risk of spinal cord injury (e.g. severe rheumatoid arthritis), no head or neck movement should take place. The only movement that is appropriate is jaw distraction.
Bleeding (Oral) Patients
Management of patients with blood in the oropharynx can present a particular challenge to the intubator. These patients are best managed in a slightly head-down position. This allows blood to pool in the upper pharynx away from the vocal cords. Adequate suction is the key to success; two suction devices may be required.
Remember
A predicted difficult intubation may actually be easy but conversely some “easy” intubations turn out to be difficult. This necessitates the need for a fallback plan or “Plan B” (see text later).
3.3.1.3 Breathing
The initial assessment will have determined the urgency for PRSI. A hypoxic patient with a poor respiratory effort will prompt a more rapid response than one with adequate oxygenation and ventilation but who has a reduced GCS.
If a pneumothorax has been diagnosed, this should have been treated at least with needle thoracocentesis (Appendix “Needle Thoracocentesis”) prior to intubation. Positive pressure ventilation can rapidly change a pneumothorax into a tension pneumothorax, resulting in profound cardiovascular collapse. A thoracostomy ± drain (Appendix “Thoracostomy & Chest Drain Insertion”) must be performed immediately after PRSI.
(Note: Thoracostomy without a drain is only permissible in a ventilated patient. If the patient is breathing spontaneously, a thoracostomy will create an open pneumothorax, which will not improve ventilation. A valve system to prevent air entrainment during inspiration is therefore required i.e., a chest drain. (A needle/cannula thoracostomy is acceptable before RSI as this allows the release of a “tension” but is too small to allow any significant entrainment of air during inspiration)).
3.3.1.4 Circulation
Intravenous (IV) access is required and should be checked with a 10 mL flush to ensure patency. This must be adequately secured (IV sites are particularly vulnerable in the pre-hospital environment.) Ideally two sites of IV access are obtained prior to RSI in major trauma. This allows rapid infusion of blood products/fluids in one whilst leaving another available for drugs, including those for PHA. It is, however, not always practical or possible due to limb injuries or patient agitation.
In the critically ill patient intraosseous (IO) access may be appropriate if two attempts at IV access have failed or venous access is clearly very poor. Although originally only used in children, adult IO access for emergency resuscitation has become an accepted technique over the last few years, partly due to successes reported in military use (Cooper et al. 2007). IV access is still preferable, as fluids will flow more easily, whereas IO access requires pressurisation of fluid bags or use of a 50 ml syringe and 3-way tap to achieve adequate flow rates for fluid resuscitation. All RSI drugs can be administered via IO access, with intubation success rates (first pass intubation and grade of view) that match those of IV induction (Barnard et al. 2014).
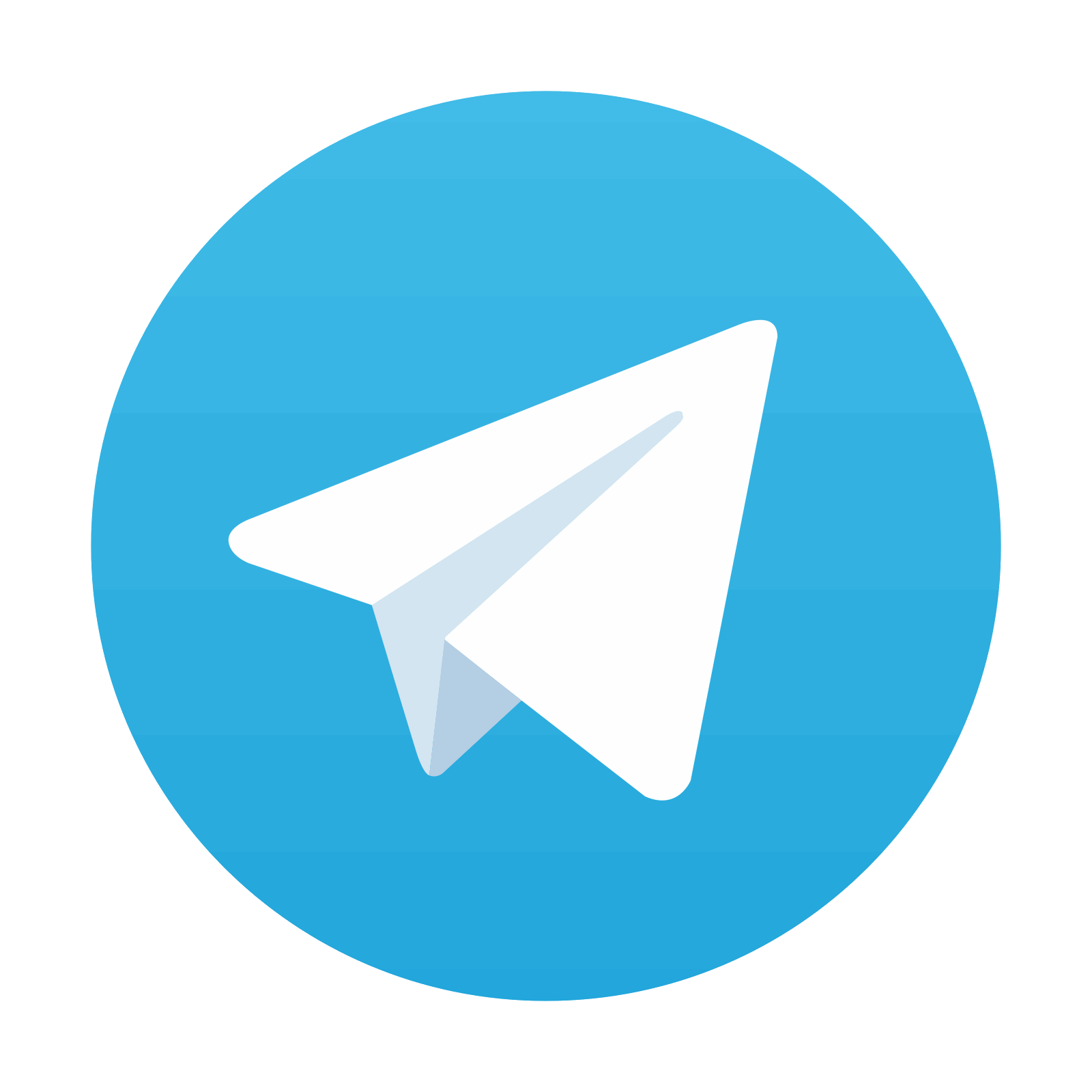
Stay updated, free articles. Join our Telegram channel

Full access? Get Clinical Tree
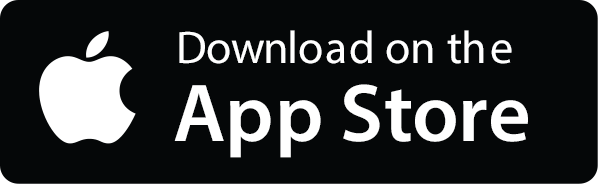
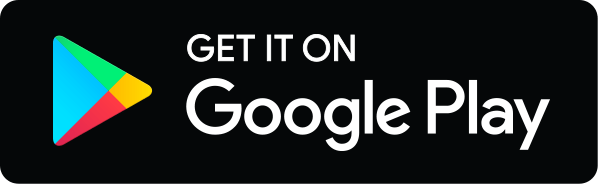
