KEY POINTS
Pneumonia is one of the most common causes of ICU admission, usually because of impending respiratory failure or hemodynamic compromise.
Pneumonia on admission to the intensive care unit presents in three different forms: traditional community-acquired pneumonia (CAP), hospital-acquired pneumonia (HAP), and the controversial entity of health care–associated pneumonia (HCAP).
By far, the most important risk factor for oropharyngeal colonization with pathogenic bacteria is the use of antibiotics; the broader the antibiotic spectrum and the longer the duration of treatment, the more likely that pathogenic bacteria will colonize the oropharynx.
Despite concern about secretion clearance, intermittent noninvasive ventilation (NIV) with careful attention to increasing secretion clearance has a survival benefit in CAP and immunocompromised patients with pulmonary infiltrates.
CAP is the leading cause of infectious death around the world and a frequent cause of ICU admission.
While Streptococcus pneumoniae remains the most common cause of severe CAP, other pathogens are overrepresented in patients admitted to the ICU, including Staphylococcus aureus, Legionella, Pseudomonas, and other gram negatives.
Because of the broader spectrum of etiologies, an aggressive diagnostic approach is appropriate in patients admitted to the ICU with CAP. Blood cultures and tracheal aspirate/bronchoalveolar lavage samples through the endotracheal tube are much more likely to be positive than in non-ICU patients.
Patients initially admitted to a non-ICU setting but subsequently requiring ICU transfer have high mortality that exceeds that of patients with equivalent illness at presentation who are admitted directly to the ICU. Presence of at least three of a set of minor criteria for severity identifies patients likely needing ICU care and the probability increases with an increasing number of these minor criteria.
The HCAP designation was developed in response to the consistent finding of pneumonia acquired while outside the hospital but caused by pathogens traditionally associated with HAP, such as MRSA, Pseudomonas, and drug-resistant Enterobacteriaceae. The definition remains very controversial.
HAP precipitating respiratory failure and ICU transfer is now more common than VAP complicating respiratory failure, although both are caused by similar multidrug-resistant (MDR) pathogens.
At least one potential pathogen is isolated in up to 75% of patients with HAP who are intubated. Access to the lower respiratory tract via the endotracheal tube is the most important reason for the higher diagnostic yield.
Broad-spectrum β-lactam antibiotics are the backbone of treatment for HAP and HCAP, but emerging antibiotic resistance patterns make choice of specific agents—piperacillin/tazobactam, late generation cephalosporins, or carbapenems—difficult. The use of combination therapy and the routine need for MRSA coverage remain controversial.
De-escalation of antibiotic therapy once the results of cultures are known is critical for management of ventilated ICU patients with HCAP and HAP.
INTRODUCTION
Pneumonia is one of the most common precipitating causes for ICU admission. It is a frequent cause of hemodynamic compromise and septic shock. Pneumonia is also one of the most common causes for the acute respiratory distress syndrome (ARDS).
Pneumonia on admission to the intensive care unit presents in three different forms: traditional community-acquired pneumonia (CAP), hospital-acquired pneumonia (HAP), and the controversial entity of health care–associated pneumonia (HCAP). HCAP is a community-onset pneumonia but with risk factors for pathogens more typical of HAP. In addition, presence of any number of immunocompromised states within each of these entities raises concern for a broader spectrum of potential etiologies, especially opportunistic pathogens. While ventilator-associated pneumonia (VAP) is technically a subgroup of HAP, this type of pneumonia occurs as a complication of critical illness, rather than the precipitating cause of critical illness. As such, VAP is covered in a separate chapter (Chap. 59).
While each of these types of pneumonia has some common characteristics, their differences warrant separate discussion. However, the basic pathophysiology of pneumonia in patients without an artificial airway is very similar and will therefore be discussed in more general terms initially.
PATHOGENESIS
The lung represents the greatest amount of surface area in contact with the external environment in humans. The lung is therefore exposed routinely to airborne infectious microorganisms. In addition, deposition of a liquid inoculum into the lower respiratory tract occurs on a frequent basis secondary to microaspiration. As a result, the lungs and entire respiratory tract have effective and redundant host defense mechanisms in order to respond to this infectious challenge. Despite this, lower respiratory tract infections remain the leading causes of infectious death, even in the modern world.1 Some have suggested that all humans remain immunodeficient2 and that the decrease in overall mortality from pneumonia and influenza in developed countries is likely due to salvage by antibiotic therapy.
Airways below the vocal cords and the alveolar spaces have generally been thought to be sterile. However, recent data using nonculture molecular tools have suggested that the microbiome of the lower respiratory tract may not be free of bacteria.3 This is clearly true in patients with HAP and HCAP, as well as patients with chronic airway disease including chronic bronchitis and bronchiectasis. Alterations in this microbiome are likely to predispose to subsequently culture-positive infections.
In contrast, bacteria are abundant in the upper respiratory tract, reaching concentrations as high as 1010 to 1012 colony-forming units (cfu)/mL. While most of these bacteria are generally considered nonpathogenic, normal oropharyngeal and nasal colonization includes potential pathogens such as Streptococcus pneumoniae, Staphylococcus aureus, and Neisseria meningitides.
In patients with HAP and HCAP, the bacterial milieu of the oropharynx changes dramatically, with the emergence of colonization by gram-negative Enterobacteriaceae and methicillin-resistant S aureus (MRSA). This gram-negative colonization can occur within the first 3 days of admission of moderately ill patients.4,5 Most of these gram-negative colonizers are endogenous flora from the patient’s own gastrointestinal tract. Other pathogens can be introduced via poor infection control practices, including poor hand washing by caregivers, or from the environment. However, the oropharynx is remarkably refractory to colonization simply by exposure to pathogens. Pseudomonas aeruginosa and Aspergillus species are fairly ubiquitous in the environment, even in the hospital, but pneumonia from these pathogens essentially never occurs unless patients have been exposed to prolonged antibiotic therapy.
Risk factors for altered oropharyngeal flora that are particularly pertinent to patients likely to be admitted to the ICU are listed in Table 65-1. By far, the most important risk factor is use of antibiotics; the broader the antibiotic spectrum and the longer the duration of treatment, the more likely that pathogenic bacteria will colonize the oropharynx. Colonization with bacteria that are not native to the oropharynx is the principal factor discriminating between the two community-onset pneumonias, CAP and HCAP.
For the majority of bacterial pneumonias, aspiration of oropharyngeal secretions is the dominant mechanism by which bacteria gain entrance into the lower respiratory tract and alveoli. Usually, the volume of secretions is relatively small and is termed microaspiration. Occasionally, obvious large volume aspiration can occur, leading to severe pneumonia if the bolus is infectious or markedly predisposes to pneumonia even if noninfectious, such as with enteral feedings. Microaspiration is likely the predominant cause in CAP and VAP, while macroaspiration plays a bigger role in HAP and HCAP.
The terms aspiration and aspiration pneumonia have been used for multiple clinical entities, both infectious and noninfectious (Table 65-2). As suggested above, most bacterial pneumonias result from an aspiration episode and could therefore be technically called aspiration pneumonia. However, this term often is associated with a clinical entity of anaerobic pleuropneumonia (Fig. 65-1) resulting from a past episode of aspiration associated with loss of consciousness, usually associated with acute alcohol intoxication or a seizure disorder.6 The key differentiating factor for this diagnosis is that the aspiration episode occurred days to weeks, even months, prior to presentation to the hospital. While this disorder is one of the aspiration syndromes, its frequency has markedly decreased in the last few decades.
Aspiration Syndromes
Syndrome | Infectious | Major Pathogen | Clinical Scenario |
---|---|---|---|
Anaerobic pleuropneumonia | Yes | Anaerobes | Loss of consciousness in past—alcohol abuse, seizure disorder |
Large-volume gastric aspiration | Vomiting, esophageal motility disorders | ||
(Contents of bolus determine resultant syndrome) | |||
Low pH | No | Acute lung injury/ARDS | |
Bland, enteral feedings | Yes | Aspiration pneumonia if gastric pH not low | |
No | Aspiration pneumonitis if bilious or moderately low pH | ||
No | Atelectasis, high risk of subsequent pneumonia | ||
Small bowel contents | Yes | Gram negatives, anaerobes | Small bowel obstruction, ileus |
Small volume aspiration | |||
Oropharyngeal | Yes | Anaerobes, normal flora, any colonizer | Loss of consciousness or inability to protect airway—stroke, sedation, metabolic encephalopathy, etc |
Aspiration pneumonia | |||
Gastric | No | Usually associated with gastroesophageal reflux | |
Acute—bronchospasm/asthma or cough | |||
Chronic—cough syndrome, bronchiolitis obliterans, pulmonary fibrosis |
In contrast, viral CAP and some forms of bacterial pneumonia result from droplet inhalation. Legionella pneumophila HAP from contaminated water sources is a good example of an inhalational bacterial pneumonia. However, many of these infections actually represent contiguous extension from the infected oropharynx, rather than true inhalation pneumonia. Therefore, microaspiration may still play a significant role even in these infections.
Because the lower respiratory tract is discontinuously exposed to infectious microorganisms, a wide variety of redundant host defense mechanisms are available to deal with this infectious challenge.
Airway Defense: Airway defenses are critical once an infectious bolus is aspirated into the trachea or proximal airways and for inhaled pathogens and particulate matter. The critical importance of mucociliary clearance is abundantly illustrated in the genetic defects in cystic fibrosis and primary cilial dysfunction.7 A variety of mucins are induced by pathogens but may actually dampen the inflammatory response in some cases.8 The effect of cigarette smoking and antecedent viral infection are well-known risk factors for development of pneumonia. However, the airway epithelium cells are also immunologically active.9 A number of antimicrobial peptides are secreted by these cells, including defensins, lactoferrin, lysozyme, and cathelicidins. Immunoglobulin (Ig)-A and complement components are also secreted into the airway during inflammation.
Alveolar Defense: The resident alveolar macrophage is the key component of host defense at the alveolar level.10 They are able to clear most inhaled infectious and noninfectious challenges, as well as smaller degrees of aspiration. More importantly, macrophages are the source of a host of cytokines and chemokines, which recruit neutrophils and initiate a variety of other components of innate immunity. In addition, macrophages and dendritic cells are key antigen-presenting cells, important in the initiation of humoral immunity.
The alveolar epithelial cell is not a simply passive barrier to microbial invasion but is an integral part of host defense. Critical to airway and alveolar epithelial defense, as well as that of macrophage and neutrophils, is the ability of these cells to recognize pathogen-associated molecular patterns (PAMPs). The Toll-like receptors (TLRs) and nucleotide-binding oligomerization domain (NOD) receptors are important examples. Binding of microbial markers to these receptors results in both enhanced killing and initiation of the cascade of cytokines and chemokines characteristic of innate immune response.
A variety of other molecules contribute to alveolar defense. IgG and complement components passively diffuse into the alveolar space. Their importance is illustrated by genetic deficiencies, which are associated with frequent pneumonia. In addition, surfactant protein (SP)-A and SP-D, which belong to the collectin family and are secreted by alveolar type-II cells, have the ability to bind bacterial carbohydrates. Surfactant itself increases opsonization of bacteria.
While part of the host response in many severe infections, the role of the coagulation system may be uniquely important in pneumonia.11 Alveolar fibrin deposition appears to assist in trapping bacteria, especially in the exudative phase of pneumonia, allowing macrophages and neutrophils to capture and engulf bacteria. The association of thrombocytopenia12 and elevated fibrin degradation products13 and adverse outcome from community-acquired pneumonia lend credence to the important role of coagulation.
Vascular Defense: Localizing an infection to the alveolar space is an important function of host immunity. Even the presence of bacterial DNA in peripheral blood appears to correlate with mortality.14
The factors important in prevention of the bacteremia and defending the vascular space in pneumonia are poorly understood. Clearly, preformed antibody is important, since the most incontrovertible evidence of pneumococcal vaccine efficacy is prevention of invasive disease, principally bacteremia and empyema.15 The ability to opsonize bacteria is also important since deficiencies in mannose-binding lectin and complement are also associated with increased bacteremia and invasive pneumococcal disease.16,17 The role of the spleen in clearing opsonized bacteria also appears important for pneumonia due to pneumococci and other encapsulated bacteria.
PATHOPHYSIOLOGY
Given the near constant infectious challenge, the airway and lower respiratory tract host defenses function without any clinical manifestations in the overwhelming majority of cases. However, when an infectious challenge is no longer contained by local host defense, systemic manifestations can be seen. These include fever as a result of TNF and IL-1 release by alveolar macrophages and other cells, leukocytosis in response to IL-6 and G-CSF release, and the resultant increase in secretions and change to purulence as neutrophils are recruited to the lower respiratory tract. In addition, release of these and other cytokines results in a localized alveolar-capillary leak syndrome, which manifests as a radiographic infiltrate and results in hypoxemia. The degree of localized capillary leak and local cytokine levels is equivalent to that seen more diffusely in ARDS. The inflammatory response can extend to the pleural surface, resulting in pleural effusions and pleuritic chest pain. These clinical manifestations are common in all forms of pneumonia to varying degrees.
The above manifestations are primarily a result of the host response to infection, rather than from the microorganism. Occasionally clinical manifestations may result from specific pathogen-related factors. Mild hemoptysis can result from the alveolar capillary leak syndrome but massive hemoptysis usually indicates pathogen invasion of the pulmonary vasculature. Massive hemoptysis usually results from release of exotoxins by the pathogen and is classic for S aureus18 and Aspergillus sp. These same exotoxins and microbial enzymes can also cause actual lung necrosis, leading to cavities on chest radiographs (Fig. 65-2) and even pneumothoraxes. In addition to S aureus and Aspergillus, the anaerobic pleuropneumonia syndrome (Fig. 65-1) and Pseudomonas aeruginosa are in the etiologic differential. While unusual manifestations of pneumonia, patients admitted or transferred to the ICU are more likely to have these complications.
The mechanism of hypoxemia in pneumonia results from the full range of ventilation/perfusion relationships. Lobar pneumonia is classic for causing shunt physiology. Part of the severe hypoxemia seen in these situations is a result of a block in the normal hypoxic vasoconstriction, thought to be due to mediator release from the bacteria. Some increase in dead space ventilation occurs as a result of lobar consolidation as well. However, CO2 retention is much more likely to be due to impending respiratory muscle fatigue and/or decreased central respiratory drive from septic encephalopathy, particularly when the latter is combined with either uremia or underlying hepatic disease.
Respiratory failure is the most common reason for ICU admission for pneumonia. In addition to ineffective gas exchange due to shunt and ventilation/perfusion mismatching, cytokine release associated with the systemic inflammatory response syndrome (SIRS) results in increased minute ventilation due to the resetting of central respiratory drive. In addition, pulmonary compliance is decreased by the consolidation and airway resistance may be increased by the presence of secretions. These both result in substantially increased work of breathing. Pleuritic chest pain and splinting may also contribute to the worsening gas exchange.
Septic shock is also a manifestation of severe pneumonia.19 For pneumonia acquired in the community, the overwhelming majority of patients are hypovolemic at the time of presentation. However, this may not be true for patients with HAP. The pathophysiology and mechanisms of septic shock from pneumonia probably vary somewhat by microorganism but are likely due to similar mechanisms as septic shock from other sources (see Chap. 64).
GENERAL TREATMENT STRATEGIES
Understanding the pathophysiology of pneumonia can lead to rational treatment strategies. While many principles apply to severe infections in general, several aspects are unique to the treatment of pneumonia.
Obviously, appropriate antibiotic therapy is key to adequate control of pneumonia. The time to initial antibiotic dose appears to be an important determinant of outcome if the patient is in septic shock.20 The benefit of rapid initiation of antibiotics in other clinical presentations is less clear, particularly if the patient has inadequate volume resuscitation. The Jarisch-Herxheimer reaction sometimes seen with the use of cell wall active antibiotics and highly susceptible bacteria may actually lead to hypotension if antibiotics are given prior to adequate fluid resuscitation. Appropriate empirical antibiotic therapy will be discussed below for each of the separate clinical pneumonia syndromes.
If release of exotoxins appears to be playing an important role, such as with S aureus and some streptococcal pneumonias, use of antibiotics that interfere with ribosomal protein synthesis may be of some benefit. This appears to be particularly important for community-acquired methicillin-resistant S aureus (CA-MRSA).21 The concern in this infection is that neither vancomycin nor linezolid is rapidly bactericidal, allowing viable bacteria to continue to release exotoxin. However, linezolid suppresses exotoxin production in viable bacteria, as does clindamycin. Therefore, use of linezolid or the addition of clindamycin to vancomycin may have benefit in pneumonia due to toxin-secreting strains. For MSSA, and streptococcal infections in general, the rapid killing with β-lactam antibiotics effectively eliminates exotoxin production.
The presence of cavities may decrease antibiotic penetration to the site of infection. This does not appear to be a problem with anaerobic pleuropneumonia, possibly because vascular invasion is not as prominent a feature as it is with Pseudomonas and CA-MRSA pneumonias. Aerosolized antibiotics may therefore be required, although this is much less likely than in treatment of VAP.
Several aspects of the pathophysiology of severe pneumonia provide unique challenges to ventilatory support.
Noninvasive Ventilation: Concern about noninvasive ventilation (NIV) in patients with pneumonia was raised early in its development. Patients clearly cannot adequately expectorate against continuous positive airway pressure (CPAP) delivered via a full-face mask. A very productive cough with pneumonia remains one of the relative contraindications to NIV.22 Failure of NIV in these patients has been associated with a subsequent prolonged duration of mechanical ventilation and increased risk of VAP.23 However, intermittent NIV with careful attention to increasing secretion clearance prior to restarting NIV and possibly use of a nasal-only mask appear to minimize this risk. NIV has been demonstrated to have a survival benefit in CAP24 and immunocompromised patients with pulmonary infiltrates.25
Severe Hypoxemia on Mechanical Ventilation: Occasionally, patients with unilateral pneumonia developed worsening hypoxemia after intubation and initiation of mechanical ventilation. Positive end-expiratory pressure (PEEP) may actually exacerbate the problem. The pathophysiologic mechanism is overdistention of the alveoli in the unaffected lung with the resultant increase in pulmonary capillary pressure shunting more blood from the unaffected lung to the pneumonic lung.
Several strategies may be effective in this situation. Clearly, these patients should be treated with a lower tidal volume, such as the 6 mL/kg ideal body weight used for ALI,26 to minimize overdistension of the unaffected lung. However, PEEP should be adjusted to maximize oxygenation rather than by use of the ARDSNet algorithm. Positioning the patient in the lateral decubitus position with the unaffected lung dependent both increases perfusion based on gravity as well as limiting overinflation of the good lung and is very effective in this situation. If hypoxemia is refractory, ibuprofen appears to block the bacterial-induced paralysis of hypoxic vasoconstriction and has been demonstrated to be safe in critically ill patients.27
Patients with CAP appeared to respond to drotrecogin alfa activated and tifacogin much better than patients with HAP or nonpneumonia infections,28,29 consistent with the important role that the coagulation system plays in CAP. However, a negative prospective randomized trial of tifacogin in severe CAP (SCAP)19 and of drotrecogin alfa activated in all-cause septic shock did not confirm these subgroup analyses and neither drug is now available clinically.
COMMUNITY-ACQUIRED PNEUMONIA
Community-acquired pneumonia continues to be a frequent cause of morbidity and mortality. Worldwide CAP is the leading infectious disease cause of death and the third leading cause of death overall.1 Despite continued advances in a multitude of areas in medicine, the mortality rate from CAP has changed very little in the past four decades. In addition to the deaths within the hospital, patients admitted to the hospital with pneumonia are at an increased risk of death for months to years after discharge, relative to age-matched controls.30-32
For the year 2000, over 1 million patients were hospitalized in the United States, and 65,000 deaths were attributable to CAP and influenza.33 The financial cost is substantial as well, estimated at over $9 billion per year.34
Approximately 10% of all patients hospitalized with CAP require ICU admission.35-38 Hospitalized CAP patients carry significant mortality depending on the severity of illness. Several studies have reported a mortality rate of approximately 10% in hospitalized ward patients, and 30% to 60% mortality in patients that require ICU admission.19,39 SCAP is even more burdensome to health care systems as the mean duration of hospitalization is 6 days at a cost of approximately $7500 for ward patients compared to 23 days and $21,144 for ICU patients.40
The most important determinant of hospitalization and mortality in patients with CAP is the presence of chronic comorbid conditions.38,39,41-44 The most common comorbid illnesses in patients with SCAP are chronic obstructive pulmonary disease (COPD),38,45,46 which is present in up to half, followed by alcoholism, chronic heart disease, and diabetes mellitus.38,44,47
While all CAP studies identify S pneumoniae as the leading pathogen causing CAP, the frequency of other pathogens varies regionally or with outbreaks of particular pathogens (Table 65-3). More importantly, significant differences between the etiology of milder pneumonia and severe disease exist. For example, Legionella pneumophila appears to be more common in SCAP, at least in some areas,48 while other atypical pathogens like Mycoplasma pneumoniae and Chlamydophila pneumoniae are much less common. Gram-negative pathogens such as Escherichia coli and Klebsiella pneumoniae, again with significant regional differences, are also more common in severe disease.49 While uncommon in most series, P aeruginosa may also be an important SCAP pathogen in some centers. Whether this is due to innate virulence of the pathogen or a reflection of the comorbidities in patients who acquire them is uncertain.
Microbial Etiology of Community-Acquired Pneumonia Admitted to the ICU
Common | Uncommon | Rare |
---|---|---|
S pneumoniae | Haemophilusinfluenzae | Burkholderia pseudomallei |
S aureus | Enterobacteriaceae | Francisella tularensisa |
Legionella spa | P. aeruginosa | Coronavirus (SARS) |
Other streptococci | Chlamydophila pneumoniae | Parainfluenza virus |
Influenza | Mycoplasma pneumoniae | Adenovirus |
Respiratory syncytial virusb | Acinetobacter sppa | Hantavirusa |
Human metapneumovirus | Mucormycoses | |
Aspergillus sp | Endemic fungia | |
M. tuberculosis |
Knowledge of the local etiology is particularly important in the setting of SCAP and shock as this will significantly impact on both empiric therapy and the microbiological investigations ordered. Examples of pathogens that can cause severe pneumonia and septic shock that are significant considerations in some areas and nonexistent in others are Burkholderia pseudomallei, Acinetobacter spp, L longbeachae, and Francisella tularensis.
Recently there has been increasing concern over CA-MRSA as a cause of SCAP.18,21 Mortality rates from this pathogen may be quite high, particularly when associated with the virulence factor Panton-Valentine leukocidin. Although the current prevalence of MRSA is too low to influence general CAP antibiotic guidelines, this may well change in the near future.
Use of molecular diagnostic techniques demonstrate that streptococci other than S pneumoniae, including S pyogenes,50S mitis, S agalactia, or S milleri,51 may also be important pathogens for CAP. In the past, many of these have been dismissed as oral flora when cultured from sputum and they only rarely caused bacteremia. Some of these species are more resistant to penicillin than S pneumoniae and may explain some penicillin treatment failures in culture negative patients.
Viral pneumonia in adults was generally underappreciated until the SARS epidemic and the novel 2009 H1N1 influenza A pandemic. Even in CAP patients admitted to the ICU, viral pneumonia probably plays a significant role.52 This is even more likely in immunocompromised patients. The spectrum of respiratory viruses has also increased with recent recognition of human metapneumovirus, Bocavirus, and the SARS-like coronaviruses with increased use of molecular diagnostic techniques. One mechanism by which viral infections can precipitate ICU admission is the common association with increased bronchospasm in patients with obstructive lung disease. The pneumonia itself may be minimal but the associated bronchospasm will require either frequent aerosols or ventilatory support.
Patients with endemic fungal pneumonia, such as histoplasmosis, blastomycosis, or coccidiomycosis, and tuberculosis can also present to the ICU with CAP-like symptoms. While uncommon, failure to recognize these pathogens not only leads to poor patient outcome but also exposes critical care staff to possible infection. Opportunistic fungi, such as Aspergillus and Mucor, would be more common in patients with HCAP or HAP.
Clinical Criteria: Pneumonia is generally diagnosed by presence of abnormalities in three groups of clinical criteria: (1) evidence of infection—including fever, subjective chills or rigors, or hypothermia; leukocytosis, leukopenia, or immature white blood cells; and other biomarkers, (2) evidence that the infection is localized to the lung—including increased sputum production, change to purulence or hemoptysis, dyspnea, chest discomfort, rales or signs of consolidation on physical examination, and (3) an abnormal chest radiograph. In certain circumstances, variations in clinical findings are also compatible with pneumonia such as confusion in the elderly as evidence of infection53 or wheezing in a child as evidence that an infection is localized in the lung, such as viral pneumonia. While absence of any one of these three criteria should call into question the accuracy of the diagnosis of CAP, clear-cut cases of pneumonia have occurred in the absence of all of them, including radiographic infiltrates. The differential diagnosis of CAP is listed in Table 65-4.
Differential Diagnosis for Community-Acquired Pneumonia
Acute exacerbation of chronic obstructive lung disease (COPD) |
Central airway obstruction with atelectasis |
|
Atypical pulmonary edema |
Pulmonary embolism/infarction |
Hypersensitivity pneumonitis |
|
Acute exacerbation of pulmonary fibrosis |
Acute eosinophilic pneumonia |
Vasculitis |
Bronchoalveolar carcinoma |
Bronchiectasis |
Bronchogenic cyst |
The elderly are notorious for not mounting a fever in response to pneumonia53 and use of antipyretics may mask fever in other patients. Other biomarkers such as C-reactive protein and procalcitonin have been used for patients with elevated leukocyte counts. “Normal” chest radiographs on presentation may represent hypovolemia and infiltrates will blossom on subsequent radiographs54
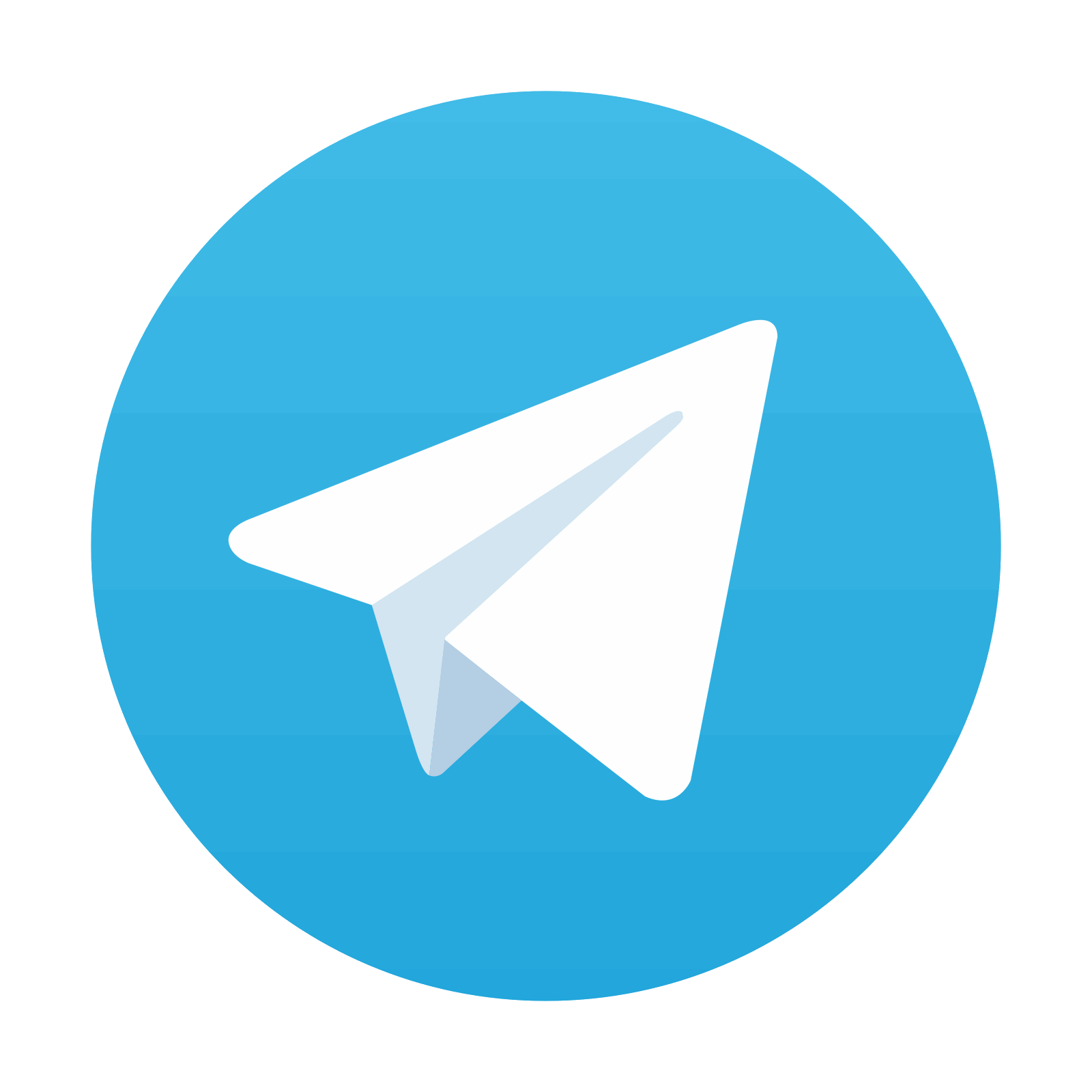
Stay updated, free articles. Join our Telegram channel

Full access? Get Clinical Tree
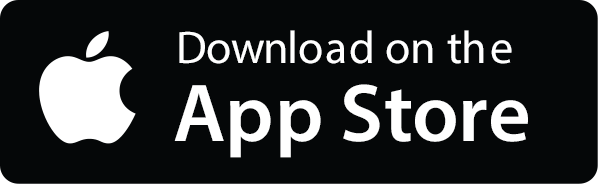
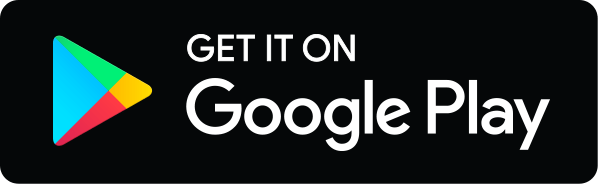
