Metabolic acidosis
Metabolic acidosis is defined as a clinical process that leads to a decreased blood pH (acidemia) and a decreased HCO 3 level. It is caused by a gain of acidic compounds and/or loss of a base, usually bicarbonate. Electrolyte movements can also play a role in acid–base balance that are sometimes manifested as an increased anion gap.
Physiologic mechanisms of metabolic acidosis ( ) :
- •
In diarrhea, the pancreatic secretions into the small intestines have a relatively high sodium to chloride content, with a relatively higher bicarbonate concentration. Loss of such GI fluids often leads to a metabolic acidosis.
- •
In renal tubular acidosis, if the excretion of sodium and potassium are in excess of chloride excretion into the urine, the anion accompanying sodium/potassium is usually bicarbonate. This can lead to a decreased serum bicarbonate and metabolic acidosis.
- •
Hyperchloremia usually indicates a metabolic acidosis. Gains of chloride by GI absorption or intravenous administration can cause hyperchloremia. This is a physiologically complex area, but here are a few principles:
- •
If the Cl − concentration increases and the Na + concentration stays the same, an anion other than Cl − must decrease. This anion is usually bicarbonate.
- •
Hyperchloremia can occur with loss of nonchloride sodium salts from GI tract or from urine.
- •
Hyperchloremia will develop with additions of fluids containing chloride salts such as sodium, potassium, calcium, or ammonium.
- •
Gains or losses of potassium can also affect the sodium concentration, which will then affect the chloride concentration.
- •
An elevated anion gap can be associated with a metabolic acidosis. The following processes are usually associated with an increased anion gap:
- •
Lactate and acid production from anaerobic metabolism. If adequate oxygen is not available to tissues (hypoxia), cellular conditions begin to favor anaerobic metabolism with the conversion of pyruvate to lactate. While clinicians often think of this process producing lactic acid, it is actually lactate that is produced ( ) . The acid (H + ) comes from the degradation of large amounts of ATP without resynthesis.
- •
Excess production of acid from ketoacidosis. The most common type of ketoacidosis is diabetic ketoacidosis, which occurs when glucose cannot enter cells to produce adequate ATP. In the setting of low cellular glucose (serum glucose is often quite elevated in the setting of diabetic ketoacidosis), fatty acids will be oxidized to produce ketoacids for energy. Ketoacidosis can also be seen with starvation where plasma glucose levels are low, and in patients who are malnourished and suffer from chronic alcoholism.
- •
Ingestion of exogenous acids or acid-producing substances: salicylates, ethanol, ethylene glycol, methanol, etc.
- •
Inadequate renal excretion of acids. In renal failure, depending on whether glomerular or tubular failure, accumulation of H + and/or loss of HCO 3 − may occur. Because renal acidosis usually has a slow onset, hyperventilation typically provides respiratory compensation to prevent acidemia in the early stages.
Use of the anion gap in metabolic acidosis . The anion gap (AG), especially when elevated, may be useful in diagnosing the type of metabolic acidosis and in indicating the possibility of a mixed acid–base disorder ( ) , as shown in Table 2.1 .
Disorder | Decreased | Gained | Effect on AG |
---|---|---|---|
Diarrhea | HCO 3 − | Cl | Little |
Renal tubular acidosis | HCO 3 − | Cl | Little |
Lactate acidosis | HCO 3 − | Lactate | Increased |
Ketoacidosis | HCO 3 − | Ketoacids | Increased |
Mixed disorder: ketoacidosis with metabolic alkalosis | HCO 3 − | Ketoacids and | Increased (with little change HCO 3 − in total CO 2 ) |
As described earlier, the AG is the difference between the commonly measured cations (Na + and sometimes K + ) and the commonly measured anions (Cl − and HCO 3 − ). The approximate reference intervals for the AG are 8–16 mmol/L (8–16 mEq/L) when the AG = Na−(Cl + CO 2 ) and 12–20 mmol/L (12–20 mEq/L) when AG = (Na + K)−(Cl + CO 2 ).
Serum albumin as an important factor affecting the anion gap calculation. Because albumin in blood constitutes a large proportion of the “unmeasured” anions, a decrease or increase (less common) in the albumin concentration will, respectively, decrease or increase the anion gap calculation. For each g/L decrease in albumin concentration, the AG is decreased by approximately 0.25 mmol/L ( , ) . If the lab reports albumin in g/dL then each g/dL decrease in albumin concentration will result in the AG decreasing by approximately 2.5 mmol/L. This could hide an increased AG due to increased lactate in a metabolic acidosis. If hypoalbuminemia is noted, which is common in critically ill patients, the AG can be “corrected” using Eq. (2.1) to better interpret the AG. The sensitivity of an increased anion gap in detecting hyperlactatemia appears to be improved by correcting the AG for the albumin concentration ( ) .
AG corr=AG meas+0.23×(45−Alb meas)
In Table 2.1 , the AG measured (really calculated) on a hypothetical sample with an elevated lactate of 5.0 mmol/L and a normal albumin of 45 g/L is elevated at 18 mmol/L, as expected. However, on a sample with the same elevated lactate of 5.0 mmol/L, but a decreased albumin of 25 g/L, the measured AG is apparently normal at 12 mmol/L. Correcting the AG in this latter sample gives a corrected AG of 18 mmol/L. Table 2.2 also shows a hypothetical sample with an elevated albumin of 70 g/L and a normal lactate that had a spuriously increased AG of 18 mmol/L. Correcting the AG for the increased albumin gave a normal AG of 12 mmol/L. A potential concern is that this correction requires using a “normal” albumin in the calculation, such as the mean of the reference interval. It would probably be better to use the patient’s baseline albumin and AG as a reference if available.
Chemistry test | Normal albumin Elevated lactate | Low albumin Elevate lactate | Elevated albumin Normal lactate |
---|---|---|---|
Na (mmol/L) | 140 | 138 | 140 |
K (mmol/L) | 4.0 | 4.0 | 4.0 |
Cl (mmol/L) | 104 | 108 | 98 |
HCO 3 (mmol/L) | 18 | 18 | 24 |
Albumin (g/L) | 45 | 25 | 70 |
Lactate (mmol/L) | 5.0 | 5.0 | 2.0 |
AG meas | 18 | 12 | 18 |
AG corr | 18 | 18 | 12 |
Monitoring AG results in the laboratory is also a common quality control technique for detecting drift in electrolyte results. Note that this is detected by a series of unusual anion gaps, not by a single result. This could be why one report claims that the most common cause of low anion gaps is laboratory error in electrolyte measurements ( ) .
Expected compensation in metabolic acidosis ( , ) ( Table 2.3 and Fig. 2.1 ). In the initial phase of metabolic acidosis (acidemia with a low plasma bicarbonate), both pH and HCO 3 − are decreased while p CO 2 remains normal. The expected respiratory compensation is hyperventilation, which will begin to lower blood p CO 2 within 30 min, have a significant effect by 2 h, and reach maximal effect in 12–24 h ( ) . A general rule is that respiratory compensation lowers p CO 2 by 1.2 mmHg for each 1.0 mmol/L decrease in HCO 3 − below 24 mmol/L ( ) . For example, in a patient who develops metabolic acidosis and has an initial p CO 2 of 40 mmHg and initial HCO 3 − of 24 mmol/L, if the HCO 3 − decreases by 8 mmol/L from 24 to 16 mmol/L, the p CO 2 after compensation should be approximately 30 mmHg (40–1.2 × 8). The equation for this relationship is:
CompensatedpCO2=InitialpCO2−1.2×[InitialHCO3−−CompensatedHCO3−]pCO2=40−1.2×[24−HCO3]=40−10=30mmHg
Primary disorder | pH | HCO 3 − | p CO 2 | Condition |
---|---|---|---|---|
Metabolic acidosis | Dec | Dec | Norm | Early phase of met acid (<2 h) |
Dec to norm | Dec | Dec | Expected Resp compensation (≥6 h) | |
Dec | Dec | Norm to Dec | Inability to compensate | |
Dec++ | Dec | Inc | Mixed met acid and Resp acid | |
Metabolic alkalosis | Inc | Inc | Norm | Early phase of met acid (<2 h) |
Inc to norm | Inc | Inc | Expected Resp compensation (≥6 h) | |
Inc | Inc | Norm to Inc | Inability to compensate | |
Inc++ | Inc | Dec | Mixed met Alk and Resp Alk | |
Respiratory acidosis | Dec | Norm | Inc | Initial phase of Resp acid |
Dec to norm | Inc | Inc | Expected met compensation (≥24 h) | |
Dec | Norm to inc | Inc | Inability to compensate | |
Dec++ | Dec | Inc | Mixed Resp acid and met acid | |
Respiratory alkalosis | Inc | Norm | Dec | Initial phase of Resp Alk |
Inc to norm | Dec | Dec | Expected met compensation (≥24 h) | |
Inc | Norm to Dec | Dec | Inability to compensate | |
Inc++ | Inc | Dec | Mixed Resp Alk and met Alk |

Thus, if the p CO 2 is more than 3 mmHg above this calculated value, the patient may not be fully capable of compensating through hyperventilating and thus may also have an underlying respiratory acidosis. If the p CO 2 is more than 3 mmHg below this calculated value, an underlying respiratory alkalosis may be present. Either of these situations would indicate that a mixed disorder is present (metabolic acidosis with either respiratory acidosis or respiratory alkalosis).
Caution: These calculations for expected compensation should be used as guidelines in the diagnosis and should not be overinterpreted as an absolute diagnosis .
Treatment . The optimal treatment for metabolic acidosis is correcting the underlying cause, such as by administering insulin for diabetic ketoacidosis, improving oxygen delivery by increasing the oxygen content of the blood and/or improving cardiac output. Losses of fluid and electrolytes should be replaced if appropriate, dialysis for renal failure or to remove certain exogenous causes of metabolic acidosis and possible administration of bicarbonate. However, administration of bicarbonate may be harmful and is not generally recommended outside of hemodynamic instability secondary to profound metabolic acidosis ( ) .
Metabolic alkalosis
Metabolic alkalosis is an acid–base disorder characterized by an elevation in bicarbonate level above 26 mmol/L and an elevation in pH. Many disease states can induce metabolic alkalosis including excess renal loss of hydrogen ions or retention of bicarbonate, intracellular shifts of hydrogen ions, GI loss of hydrogen ions, and fluid volume loss through diuretic use without proportional loss of bicarbonate, known as “contraction alkalosis” ( ) , which is frequently associated with renal impairment because a healthy kidney can excrete large amounts of HCO 3 when it is in excess ( ) .
Mechanisms of metabolic alkalosis:
- •
Increased mineralocorticoid levels. The kidney uses an exchange mechanism in which sodium is reabsorbed and hydrogen is excreted. Because aldosterone increases retention of sodium, conditions that increase aldosterone, such as primary aldosteronism (Conn syndrome) and diuretics, can lead to hypernatremia and increased loss of hydrogen ions in the urine ( ) . Because cortisol and other steroids such as corticosterone have some mineralocorticoid activity, excess cortisol, as in Cushing’s syndrome, can lead to excretion of both K + and H + in the distal renal tubules with subsequent metabolic alkalosis and hypokalemia.
- •
GI loss of acid by vomiting. The stomach fluids are highly acidic, with a pH of approximately 1.5–3.5. Thus, the loss of gastric acid causes metabolic alkalosis with an increased bicarbonate concentration in the blood.
- •
Hypochloremia. Deficiency of Cl − in blood enhances HCO 3 − reabsorption in the renal tubule. When cations such as Na + and K + are reabsorbed, an anion must follow. Because less Cl − is available, more HCO 3 − is reabsorbed.
- •
Hypokalemia. Hypokalemia stimulates distal tubular reabsorption of HCO 3 ions. In primary mineralocorticoid excess, aldosterone increases tubular Na reabsorption and promotes the loss of K and H ions. These movements lead to extracellular alkalosis with hypochloremia, hypokalemia, and expanded ECF volume.
- •
Administration of excess HCO 3 − . Although not usually required for treatment of metabolic acidosis, infusion of sodium bicarbonate solution may sometimes be used. If excessive, HCO 3 − administration may lead to alkalosis, especially if renal function is compromised.
- •
Contraction alkalosis. This occurs when a large volume of fluid with relatively more sodium than bicarbonate is lost from the body such that the plasma bicarbonate concentration increases. This can occur with diuretics, diarrhea, cystic fibrosis, and other conditions ( ) .
- •
Hypoalbuminemia. According to the strong ion difference concept ( ) , a decrease in plasma albumin (being the predominant weak acid in plasma) causes a decreased weak acid concentration that results in a metabolic alkalosis.
Expected compensation in metabolic alkalosis ( Table 2.3 and Fig. 2.1 ). Because the normally functioning kidney can excrete large amounts of bicarbonate, a moderate bicarbonate load can be readily excreted to correct a metabolic alkalosis. However, if renal bicarbonate excretion is hindered, bicarbonate can increase in the blood leading to an increase in blood pH.
Changes in blood pH are sensed by both peripheral and cerebral chemoreceptors, such that a metabolic alkalosis consistently causes hypoventilation and an increase in blood p CO 2 to compensate the increased bicarbonate. However, while hypoventilation almost invariably occurs when metabolic alkalosis is present, other factors such as pain, for example by the arterial puncture, or hypoxemia may stimulate hyperventilation and overcome the hypoventilatory stimulus of metabolic alkalosis ( , ) .
Because they may partially offset each other, the combined stimuli for hypoventilation by alkalemia and hyperventilation by hypoxemia can present both diagnostic and therapeutic challenges. Patients who present with hypercapnia from metabolic alkalosis and hypoxemia from other causes, may have the metabolic alkalosis missed. In such cases, administering oxygen should decrease the hypoxemic stimulus for hyperventilation and reveal the hypoventilation as a compensatory response to metabolic alkalosis ( ) .
Although the maximal respiratory compensation by hypoventilation was once believed to be no more than 55–60 mmHg, compensation can elevate arterial p CO 2 to over 80 mmHg in some instances ( , ) .
In the initial phase of metabolic alkalosis, both pH and HCO 3 − are increased and p CO 2 remains normal. Within 2 h, compensation by hypoventilation should be apparent, with maximal compensation occurring by 12–24 h, which increases the blood p CO 2 to match the elevated HCO 3 − and restore the HCO 3 − / p CO 2 ratio to normal. In general, respiratory compensation increases p CO 2 by 0.7 mmHg for each 1.0 mmol/L rise in HCO 3 − . For example, in a patient who develops metabolic alkalosis, after 24 h, if the HCO 3 − increases from 24 to 32 mmol/L (8 mmol/L above a normal 24 mmol/L), the p CO 2 should be approximately 46 mmHg (40 + 0.7 × 8) ( ) . The equation for this is as follows:
CompensatedpCO2=InitialpCO2+0.7×[CompensatedHCO3−−InitialHCO3−]pCO2=40+0.7×[HCO3−−24]=40+0.7×8=46
If the actual pC O 2 deviates from this expected p CO 2 by more than 4 mmHg, the patient may have a mixed disorder due to either an underlying respiratory acidosis (if more than 4 mmHg higher) or alkalosis (if more than 4 mmHg lower).
Caution: These calculations for expected compensation should be used as guidelines in the diagnosis and should not be overinterpreted as an absolute diagnosis .
Treatment . The optimal treatment for metabolic alkalosis is correcting the underlying disorder and any factors that perpetuate the disorder. In some cases, simple hydration will gradually correct metabolic alkalosis if renal function is normal. Because Cl − depletion is commonly present, Cl − may be replaced by the administration of NaCl or KCl solutions as appropriate, with consideration of the renal function. Rarely, dilute HCl may be administered intravenously if necessary, and Brandis points out that correction of metabolic alkalosis will cause a right shift in the oxygen dissociation curve, which will increase peripheral oxygen release ( , ) . In patients with anasarca or congestive heart failure who remain in an edematous state and continue to require diuresis, acetazolamide, a carbonic anhydrase inhibitor, may be given. Acetazolamide primarily inhibits proximal sodium bicarbonate reabsorption in the kidney, thereby increasing urinary bicarbonate excretion.
Respiratory acidosis
Arterial p CO 2 is a balance between cellular production and removal by alveolar ventilation. Respiratory acidosis is usually caused by inadequate ventilation that results in excess CO 2 in the blood and a concurrent decrease in blood pH below 7.35. Acute respiratory acidosis is most commonly related to decreased ventilation, but can also be caused by increased production of CO 2 by the body, or excess CO 2 in the inspired gas ( ) . Normally, respiratory centers in the pons and medulla control alveolar ventilation by chemoreceptors sensitive to changes in p CO 2 , p O 2 , and pH, such that any increased production of CO 2 promptly stimulates hyperventilation, which maintains arterial p CO 2 at normal levels ( ) . If arterial p CO 2 is increased, it usually involves a problem with ventilation.
Mechanisms of acute and chronic respiratory acidosis:
- •
Ventilation failure due to CNS depression caused by brain injury, drugs such as opioids or anesthetics, or respiratory muscle insufficiency as seen in neuromuscular disorders, such as myasthenia gravis, amyotrophic lateral sclerosis, or muscular dystrophy.
- •
Impaired pulmonary gas exchange due to a high dead space fraction [(total ventilation—alveolar ventilation)/total ventilation]. This can be seen in a number of pulmonary diseases associated with the destruction of the alveolar space or impaired blood flow to a segment of the lung. In these scenarios, effective alveolar ventilation makes up a smaller fraction of the total minute ventilation.
- •
Chronically decreased reflex responsiveness to hypoxia and hypercapnia seen in COPD, chronic restrictive lung disease, and obesity hypoventilation syndrome ( ) .
- •
Acute airway obstruction caused by aspiration of a foreign object or blockage of an endotracheal tube
- •
Circulatory failure, which causes insufficient delivery of blood to the lungs
- •
Increased metabolism or CO 2 production in a mechanically ventilated patient who is not spontaneously breathing. If CO 2 production increases while ventilation remains constant, the blood p CO 2 will rise ( ) .
Expected compensation in respiratory acidosis ( , ) ( Table 2.3 and Fig. 2.1 ). To compensate the excess CO 2 and acid in the blood, the kidneys begin to excrete more hydrogen and ammonium ions and reabsorb more bicarbonate. During the acute phase, plasma buffering of the elevated CO 2 increases the HCO 3 − slightly, by approximately 1 mmol/L for each 10 mmHg rise in p CO 2 . Over the next several hours, the kidneys increase the reabsorption of HCO 3 − , which elevates serum HCO 3 − by about 2 mmol/L. The kidneys can also increase bicarbonate retention by urinary excretion of a high chloride (and ammonium ion) concentration relative to sodium, which would lessen bicarbonate loss. As this continues into the chronic phase (over 24 h), renal compensation occurs gradually over several days to increase HCO 3 − , reaching a plateau after 2–5 days. Even after several days, compensation generally does not return pH completely back to normal ( ) . As compensation occurs in two phases, the expected compensation has two algorithms to predict the expected blood level of HCO 3 − ( ) .
Acute Resp Acid: For each 10 mmHg rise in arterial p CO 2 above 40 mmHg, HCO 3 − should increase by about 1 mmol/L and pH should decrease by about 0.07–0.08. For example, if a patient’s breathing slows for a few hours and the p CO 2 increases from 40 to 50 mmHg, the HCO 3 − should rise by about 1 mmol/L (i.e., 25 mmol/L) and the pH should decrease by 0.08. For example, pH decreases from 7.40 to about 7.32.
CompensatedHCO3−=InitialHCO3−+0.1×(CompensatedpCO2−InitialpCO2)
pHchange=0.008×(40−pCO2)=0.008×−10=−0.08
Chronic Resp Acid: The maximal HCO 3 − response occurs in about 3 days, with the HCO 3 − increasing by 3–4 mmol/L for each 10 mmHg rise in arterial p CO 2 . For example, if the p CO 2 has increased from a patient’s baseline of 40 mmHg to a steady state of 60 mmHg for several days, the HCO 3 − should have risen about 6–8 mmol/L. In our example, if the patient’s normal HCO 3 − was 24 mmol/L, it should be about 30–32 mmol/L after 3 days of metabolic compensation. Thus, for a p CO 2 increase from 40 to 60 mmHg, the expected bicarbonate during compensation may be calculated by
CompensatedHCO3−=InitialHCO3−+0.35×(CompensatedpCO2−InitialpCO2)
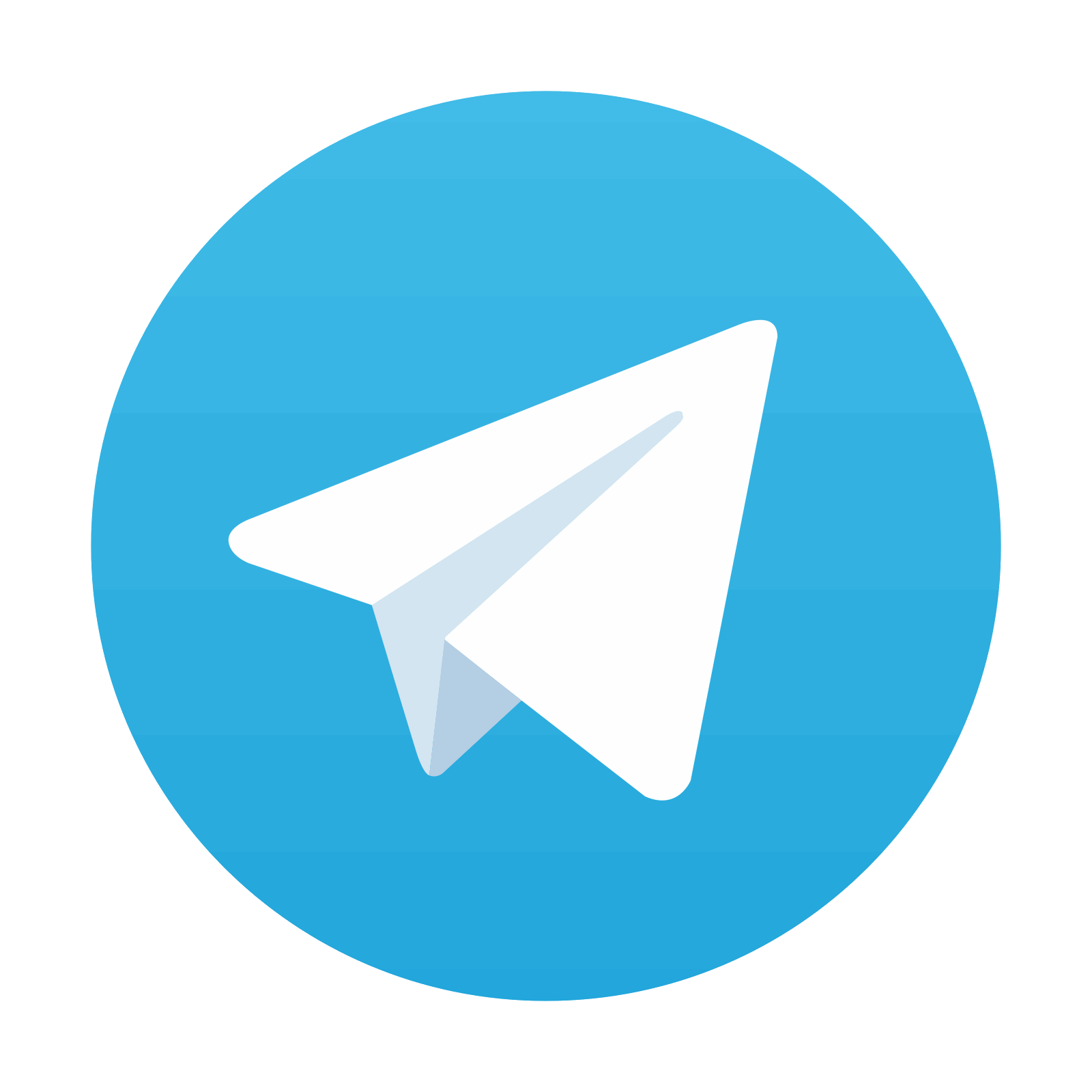
Stay updated, free articles. Join our Telegram channel

Full access? Get Clinical Tree
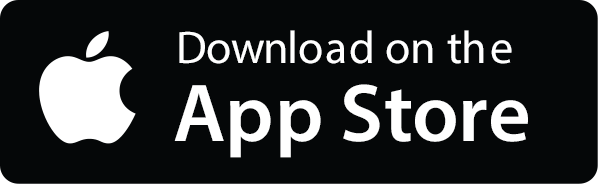
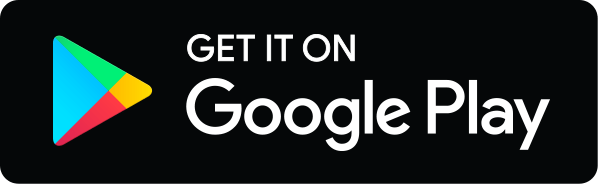
