Physiologic and Pharmacologic Substrates of Nociception after Tissue and Nerve Injury
Tony L. Yaksh
Certain classes of unconditioned stimuli that interact with visceral, somatic, or muscular sensory receptor systems give rise to a complex syndrome of behavior in the unanesthetized organism that we refer to as pain. This syndrome is frequently characterized by vocalization or efforts to escape. Stimuli able to evoke such behaviors were referred to by Sherrington as being nociceptive or painful.1 An important characteristic is that such “stimuli become adequate as excitants of pain when they are of such intensity as to threaten damage to the skin.” To generalize on this phenomenon, we note that other unconditioned stimuli, such as electrical stimuli, that do not produce evident damage will produce the same behavioral syndrome. We would thus conclude that these stimuli have activated portions of the circuitry that mimic the input produced by those stimuli that physically damage the organism. Similarly, instillation of bradykinin onto the cornea, reversible ischemia of cardiac muscle, or gallbladder sphincter contraction, although not necessarily leading to acute and immediate damage, produces similar response syndromes (e.g., vocalization, escape, guarding), which suggest that the organism’s response to the input provided by that stimulus is similar to its response to tissue-damaging stimulus. The substrate by which these classes of information gain access to the central nervous system (CNS) and are encoded is the subject of this chapter. The behavior of the organism in context thus provides corollaries that define the “painful” nature of the stimulus. In some instances, the behavioral response reflects a defined pain state (e.g., a pain report, vocalization, guarding), but the stimulus appears inadequate. Thus, a modestly intense stimulus may evoke a response normally associated with a more intense input or a light touch may result in behavior that is normally only associated with tissue injury. In the first case, we might define this behavioral state as reflecting hyperalgesia, whereas in the second case, the syndrome may be referred to as hyperalgesia or allodynia. The apparent alteration of the linkage between stimulus intensity and response constitutes an important example of the dynamic characteristics of those systems that process sensory information. As will be seen, such feedback systems, when activated by repetitive afferent input or by nerve injury, can induce a powerful alteration in the stimulus–response relationship. In some instances, this modulation serves to increase the gain of the system, leading to an exaggerated response (e.g., hyperalgesia); other systems serve to downregulate the system’s response and lead to a reduced pain response (analgesia). The appreciation of this complex relationship between stimulus and response represents one of the major advances in conceptualization of the pain response to have occurred in the past 50 years. It is now apparent that one cannot consider the afferent limb through which “pain” information travels (e.g., the pain pathway) without considering those systems that modulate excitability at every level of that very transmission.
Nociception: The Behavioral Correlates
Acute Stimulus
It is commonly appreciated that acute mechanical distortion (pressure), increases in temperature applied to the body surface, or acute distention of a hollow viscus will evoke (a) unlearned but organized escape response with decreasing latency/increasing reliability,2,3 and (b) the appearance of physiologic reflexes that are evoked by small afferent input (e.g., autonomic responses—blood pressure/heart rate)4,5 or spinal somatic reflexes (e.g., tail flick, skin twitch).6,7 In humans, experimental stimuli, such as acute thermal or electrical stimuli, will similarly evoke responses that report pain with increasing rated intensity and increasing certainty as the electrical,8,9,10,11 thermal,12,13 or mechanical14,15 stimulus intensity is increased (Fig. 32-1). These acute focal stimulus exposures, both in humans and animals, have several properties:
The focus of the behavior and the pain referral is typically limited to the site of stimulus exposure (e.g., it is somatotopically limited).
With an intense focal stimulus, it is possible that the observer will frequently report an acute sharp sensation (first pain) followed by a dull throbbing (second pain).16,17
If the stimulus is limited to a brief exposure, and if the stimulus does not itself produce a local injury, the response measure (latency, pain report) can be shown to be relatively stable over an extended sequence of exposures.
If the stimulus is extended, if it results in a persistent state of activity (as with a chemical stimulus that activates small afferents), or if it results in a local injury (e.g., tissue damage or a local burn), the pain report/behavior will show a
time-dependent enhancement, suggesting an increase in the response generated by the stimulus.17 This phenomenon will be discussed below.
In general, then, it can be seen that with an acute high-intensity stimulus there is a corresponding faithful representation of the magnitude of the stimulus and its localization on the body surface. The sensation thus induced has great teleogical merit, as it provides an alert that will evoke a tissue-protective reaction.
Persistent Stimulus
If local tissue injury occurs as a result of the stimulus, the magnitude of the response generated by a subsequent test stimulus applied to the injury site is enhanced and a previously innocuous stimulus is reported as aversive. This post-injury state is referred to as primary hyperalgesia. In this state, there is (a) a reduction in the threshold needed to produce a pain response, (b) an augmented response to a suprathreshold stimulus, and (c) an ongoing pain report. Importantly, this sensitization is observed for essentially all modalities (e.g., thermal and mechanical) and tends to be localized to the immediate locality of the injury18,19 (Fig. 32-1).
In contrast, shortly after the induction of a local injury, a region surrounding the local injury appears, substantially larger than the injury, in which low-threshold tactile (but not thermal) stimuli will evoke a significant pain report.20 Human psychophysical studies have pursued the characteristics of this event systematically by the use of intradermally injected capsaicin. This agent is known to selectively activate C primary afferents.21 In these studies, it has been shown that intradermal capsaicin will evoke a local primary hyperalgesia that dissipates within the hour and also a large, persistent, secondary hyperalgesia in which low-threshold tactile stimulation (but not thermal) will evoke a pain report.18,22,23 The mechanism of this sensitization will be considered later; however, it should be noted here that: (a) the region of secondary hyperalgesia lies considerably beyond the edge of the injury flare (e.g., the axon reflex);24 (b) the allodynia is mediated by large afferents (e.g., mechanically evoked pain is diminished by a tourniquet that blocks large afferents);18 and (c) the evolution of the secondary hyperalgesic state is prevented by a local anesthetic block of the region injected with capsaicin prior to the injection of capsaicin, but not after.25 These latter observations suggest that, in the face of a local injury, there is a locally mediated enhancement of the organism’s response to a given stimulus. This primary hyperalgesia is accompanied by the appearance of an extended area of sensitization in which low-intensity mechanical stimuli evoke a pain response. Animal models have similarly been employed in which a local injury, such as that induced by the injection of formalin or carrageenan into the skin,2,26 the generation of an acute arthritic state,27,28 a local incision,29 or a local burn (as with ultraviolet radiation)30,31 can evoke a prominent facilitation of pain behavior. These observations in humans and animals have striking significance in the treatment of pain states, as they suggest that prior interventions may reduce the magnitude of postinjury stimulus. Accordingly, as will be noted later, the systems activated by such protracted afferent input themselves have unique properties.
Nociceptive Processing: Rostrally Projecting Substrates
The following sections discuss the substrates through which information generated by high-intensity stimuli gains access to the neuraxis. Anatomically, the substrates may be broadly considered in terms of the primary afferents—the spinal cord, brainstem (medulla, mesencephalon, diencephalon), and cortex. In each case, one must consider the presumptive evidence associating activity in elements of that substrate with the
afferent and efferent connections of that substrate and the behavioral sequelae that might be predicted secondary to the physiologic manipulations (lesion, stimulation) of that substrate.
afferent and efferent connections of that substrate and the behavioral sequelae that might be predicted secondary to the physiologic manipulations (lesion, stimulation) of that substrate.
Primary Afferent Systems
Addressed here are the anatomic, physiologic, and pharmacologic characteristics of afferents as they appear to relate to nociceptive transmission.
Primary Afferent Morphology
Myelinated versus Unmyelinated Axons
Afferent fibers can be broadly classified according to whether they are myelinated or unmyelinated. Large-diameter peripheral afferents enveloped in Schwann cell sheets range in diameter from about 6 to 14 μm in the human cutaneous nerve. Nonmyelinated fibers range in diameter from 0.2 to 2.0 μm and, although not possessing the Schwann cell investment, are commonly colocated in proximity with other small fibers within a common Schwann cell sheath (bundles of Remak). Myelinated to unmyelinated fiber ratios in cutaneous nerves are about 1:3 to 1:5 in humans.32,33 Thus, while although the largest of myelinated fibers represent the largest area in a cross-section of nerve, numerically they are likely to constitute a relatively small proportion of the afferent pathways.
Peripheral Terminals
Afferent axons in the skin ramify profusely,34 losing their perineural sheath. In this process, some fibers lose their Schwann cell investment and become nonmyelinated. Conduction velocities of myelinated fibers thus decrease and approach that of unmyelinated fibers when measured near their site of termination.35,36,37 Large-diameter fibers typically develop specialized terminals with distinctly organized encapsulations constructed of non-neuronal elements.38,39,40 However, the vast majority of nerve terminals, and certainly those deriving from unmyelinated fibers, show little morphological specialization.40 Unmyelinated terminals show extensive branching in a horizontal layer within the superficial dermis. Axon collaterals enter the epidermal layer, with the basement membrane of the nerve terminal becoming contiguous with that of the epidermis. Unmyelinated fiber terminals are directed toward the stratum corneum, and there they lie between the juxtaposed epidermal cells.41,42 A similar organization is observed in different target tissues such as the cornea. In tooth pulp and knee joint, both myelinated and unmyelinated fibers appear to lose their Schwann cell sheath and show prominent local branching.20,43 Importantly, these sensory free nerve endings have been typically demonstrated to lie in close anatomic proximity to mast cells and small blood vessels (Fig. 32-2). As will be considered later, injury leads to activation of inflammatory cells and plasma extravasation, which can act upon these free nerve endings; conversely, the products released from the primary afferent terminal can degranulate mast cells and cause vasodilatation and plasma extravasation.
These nonspecialized or so-called free nerve endings (e.g. without specialized structures) commonly display agranular vesicles and numerous mitochondria (Fig. 32-3).41,43,44,45,46,47 As will be discussed in the comments on the peripheral pharmacology of afferents, these vesicle populations provide the substrate for the release of locally active agents at the distal terminals of the sensory axon. It appears likely that many “free” nerve endings are characteristically sensitive to physical stimuli that evoke pain behavior. Thus, (a) tissue damage leads to activation of small-diameter myelinated and unmyelinated fibers, and most small-diameter fibers end in unencapsulated terminals; and, (b) electrical, mechanical, thermal, or chemical stimuli, applied to certain structures such as the cornea or the tooth pulp, which possess few or no encapsulated endings, will evoke a pain report.10,48,49,50
Primary Afferent Neuron Morphology and Markers
Primary sensory neurons are pseudo-unipolar, with their soma located in the dorsal root ganglion (DRG) or, as in the fifth nerve, in the trigeminal ganglion. The DRG may be divided into two categories51: (a) large, lightly staining cells (type A), which give rise to large-diameter myelinated fibers, and (b) small, darkly staining cells (type B) from which derive the small-diameter myelinated or unmyelinated primary afferent axons.52,53
Morphologically, type A and type B DRG neurons have been differentiated on the basis of structural components such as relative content of granular and smooth endoplasmic reticulum and of ribosomes, neural filaments and microtubules, Golgi apparatus, and lysosomal bodies.54
Primary Afferent Neuron Markers
In addition to morphology, content and cell surface markers have been shown to distinguish subpopulations of afferents (Table 32-1).
Type A cell bodies associated with larger myelinated axons but not type B cells are immunopositive for the neurofilament marker RT97.
Type B DRG cells giving rise to small unmyelinated primary afferents may be subdivided into at least two relatively distinct populations by specific cell surface markers and content. In general, small afferents may contain peptides such as substance
P (SP), somatostatin (SST), and calcitonin gene-related peptide (CGRP), and display immunoreactivty for the transient receptor potential vanillin (TRPV1) receptors. Conversely, a second population is not peptidergic, binds to the lectin Griffonia simplifolia (IB4) and to the neurotrophin tropomyosin receptor kinase A (TrkA), and displays fluoride-resistant acid phosphatase (FRAP) and P2X3 receptors55,56,57,58,59,60,61,62,63,64,65.
P (SP), somatostatin (SST), and calcitonin gene-related peptide (CGRP), and display immunoreactivty for the transient receptor potential vanillin (TRPV1) receptors. Conversely, a second population is not peptidergic, binds to the lectin Griffonia simplifolia (IB4) and to the neurotrophin tropomyosin receptor kinase A (TrkA), and displays fluoride-resistant acid phosphatase (FRAP) and P2X3 receptors55,56,57,58,59,60,61,62,63,64,65.
Table 32-1 Summary of morphological markers of primary afferents | ||||||||||||||||||||||||||||||||||||||||||||||||||||
---|---|---|---|---|---|---|---|---|---|---|---|---|---|---|---|---|---|---|---|---|---|---|---|---|---|---|---|---|---|---|---|---|---|---|---|---|---|---|---|---|---|---|---|---|---|---|---|---|---|---|---|---|
|
Primary Afferent and Axon Transport
Because axon terminals do not possess ribosomes with which to manufacture peptides and proteins, such materials are synthesized in the soma66 and transported to the distal terminals of the neuron by means of an energy-dependent axon transport system.67,68,69 Differences have not been observed between myelinated and unmyelinated fibers in the velocity or character of axon transport,70,71 and the ability to conduct an action potential does not depend on the viability of axon transport systems. However, changes in the transport of materials to the distal terminals appear to play several important roles in the maintenance of the axon. Blockade of axon transport (as with certain neuropathy-inducing agents such as colchicine or vinblastine) results in trophic changes in axon structure.72 Moreover, the likely role of certain peptides in primary afferent neurotransmission and the inability of nerve terminals to synthesize peptides argue for the importance of such a transport system.
Afferent Transduction and Activation Biology
Transduction
As indicated, sensory afferents may be activated by different physical stimuli or a different range of intensities for a given physical modality. This specificity results from the transduction properties of the axon terminal. Transduction is accomplished by specialized structures or receptors at the peripheral terminal. Thus, the myelinated afferent axon displays specialized nerve endings that define the characteristics of the stimulus that initiates axon depolarization (e.g., pacinian corpuscles for rapidly adapting mechanoreceptors), whereas unmyelinated axons typically show “free nerve endings.” Although these terminals display no evident morphologic specialization, they will express specific transducer proteins. Thermal, mechanical, or chemical stimuli evoke excitation of the peripheral terminals through an interaction with transduction proteins. For small sensory afferents, a series of transducing channels (TRPs) sensitive over ranges of hot, warm, cold, have been identified. In addition several channels recognize local pH (TRPV1 and the acid-sensing ion channels [ASIC]). When activated by the appropriate stimulus range, these channels pass sodium (Na+) and/or calcium (Ca2+) ions to depolarize the membrane and activate voltage sensitive sodium (NaV) channels to generate action potentials (Fig. 32-4). In addition to these channels, the terminals of primary afferents are also decorated with a wide variety of receptors for products released from local tissue after injury, inflammation, or plasma extravasation. These are reviewed later.
Action potential frequency is a function of local depolarization and hence stimulus intensity. Some channels also have associated chemical sensitivity. Consistent with the response properties endowed to the sensory axon by the channel activation profile, TRP channels with high temperature threshold profile (such as TRPV1) and with cold threshold profiles (such as TRPM8) are activated respectively by capsaicin and menthol.73 This selective activation accounts for the psychophysical experience of heat and cold arising from local application of these two chemicals to the skin. Some transducer channel proteins (ASICs) show an enhanced activation in the presence of hydrogen ions (H+), thus accounting for the painful stinging associated with acidic solutions. Interestingly, the wound environment is often acidic. This may directly lead to activation of small afferents. In addition, the acidic environment may shift the stimulus response function of thermal transducing afferents, such that they may be activated by temperatures at or near local skin temperatures, leading to one component of post incision pain
The mechanisms of mechanical transduction are not well defined. Two mechanisms hypothesized to account for such transduction are: (a) mechanical distortion of terminal membranes may yield increased conductance in a nonspecific, large-pore, mechanically gated cation channel, leading to a depolarized membrane;74 and (b) mechanical distortion of satellite cells releases adenosine triphosphate (ATP), which could then activate purine receptors (P2X) on the afferent terminal.75
In addition to the mechanisms whereby thermal and mechanical transduction can occur, it is appreciated that a variety of chemical stimuli exist in the peri-terminal milieu, particularly
after tissue injury. These can activate small afferents. These chemical entities will be considered further in the discussion of the origins of activation after tissue injury and inflammation.
after tissue injury. These can activate small afferents. These chemical entities will be considered further in the discussion of the origins of activation after tissue injury and inflammation.
Action Potential Conduction
Based on conduction velocities, primary afferents have been subdivided into Aβ- (approximately 30–100 m/sec) and Aδ-fibers (approximately 4–30 m/sec). The C component consists of those fibers conducting at less than 2.5 m/sec.76,77 Conduction velocity of a primary afferent is depdedent upon three factors: (a) axon diameter, (b) myelination, and (c) distribution of Na+ channels. Based on classical studies, muscle afferents have been divided into the following groups on the basis of axon diameter: group 1, greater than 12 μm; group II, 6 to 12 μm; group III, 1 to 6 μm; and group IV, less than 1 μm.78 Groups I to III are considered to be myelinated. In myelinated axons, conduction occurs between the nodes formed by adjacent Schwann cells.The larger the axon, the greater is the internodal distance and the greater the rate at which depolarization will travel over a length of axon. Given the close relationship between axon diameter in myelinated/unmyelinated axons and conduction velocities, the following relationships are normally accepted: group I, no sensory homolog; group II, Aβ; group III, Aδ; and group IV, C (Table 32-2).
Table 32-2 Summary of the sensory afferents and their characteristics | |||||||||||||||||||||||||||||||||||||||||||||||||||||||||||||||||||||||||||||||||||||||||||||||||||||||||||||||||||||||||||||
---|---|---|---|---|---|---|---|---|---|---|---|---|---|---|---|---|---|---|---|---|---|---|---|---|---|---|---|---|---|---|---|---|---|---|---|---|---|---|---|---|---|---|---|---|---|---|---|---|---|---|---|---|---|---|---|---|---|---|---|---|---|---|---|---|---|---|---|---|---|---|---|---|---|---|---|---|---|---|---|---|---|---|---|---|---|---|---|---|---|---|---|---|---|---|---|---|---|---|---|---|---|---|---|---|---|---|---|---|---|---|---|---|---|---|---|---|---|---|---|---|---|---|---|---|---|
|
Action potential conduction in primary afferents is dependent upon several families of voltage-gated sodium channels (VGSC; NaV). These channels consist of tetramers constructed of α and β subunits. Multiple subunits (10 α and three β) define the gating properties of the channel, ion selectivity, and its pharmacology. All VSGC are blocked by agents such as lidocaine, but the channels may be divided into those that are tetrodotoxin-resistant (NaV 1.8 and NaV 1.9) and those that are tetrodotoxin (TTX)-sensitive (the remainder). Molecular techniques have shown that NaV1.6 and NaV1.7 are present in virtually all sensory neurons. NaV1.1 is preferentially expressed in large-diameter sensory neurons; NaV1.8 and NaV1.9, the TTX-resistant channels, are only present in small-diameter neurons. The gating properties of the channels are strongly regulated by phosphorylation at a number of consensus sites. Thus, such phosphorylation can lead to a lowering of depolarization thresholds and an increase in the duration of the channel opening. Such phosphorylation can thus lead to an increase in action potential frequency for any given depolarizing stimulus79.
Response Properties of the Primary Afferents
The functional characteristics of the primary affferents may be stated in terms of these notable properties:
Under control conditions, in which little or no stimulus is present, and no injury or inflammation occurs, little or no ongoing activity occurs. Under resting conditions, such spontaneous discharge frequencies may be on the order of 0.1 Hz or less.
In the face of a stimulus of the appropriate modality, the sensory axon will display an increasing frequency of discharge, with the rate of discharge increasing monotonically over a range of stimulus intensities.
The content of the message delivered by a specific axon will depend upon not only the frequency of its discharge, but on its connectivity as well. Thus, a high-threshold and low-threshold A∂ axon may have similar firing rates at two different temperatures, each of which is associated with distinct psychophysical experiences (Fig. 32-5). Although the axons are often functionally subdivided into low- and high-threshold responsiveness (Fig. 32-5), the transduction response profiles of different members of a population of afferents may range relatively continuously between the two extremes.
Correlation of Behavior and Sensory Afferent Activity
Direct Activation of Axons
Electrical stimulation that produced synchronous volleys in high-threshold cutaneous afferents (and therefore slowly conducting and of small diameter) evoked massive sympathetic discharges and pseudo-affective responses even in lightly anesthetized animals,77 suggesting that the activation was associated with a noxious stimulus. In humans, stimulation that evoked only fast-conducting volleys in cutaneous nerves gave rise to sensations of tickling or light pressure, whereas stimulation that evoked fast and slow components resulted in pain.80,81 High-intensity stimulation applied during selective blockade of large-diameter fibers by anoxia, leaving Aδ- and or C-fibers active, produces a short-lasting pain of a pricking nature. The sensation appears similar to that often reported as “first” pain. Activation limited to more slowly conducting fiber populations (C or group IV) gave rise to dull, diffusely localized sensations that were likened to burning or so-called “second pain”.81,82,83,84,85,86,87
Afferent activity evoked by stimuli associated with behavioral signs of pain
Although electrical activation of gross fiber populations may produce pain, it does not necessarily follow that when a high-intensity somatic stimulus is applied, specific afferent populations are activated, or that their specific activation is uniquely correlated with pain sensations. Using single-unit recording in human nerve fascicles in situ, it has been shown that stimuli that produce sensations of light touch or vibration are accompanied by the activation of rapidly conducting afferents.88,89,90,91 Needle pricks associated with verbal reports of marked discomfort evoked rapid firing in C cutaneous afferents (0.5–1.5 m/sec). Chemical (acetic acid or intradermal histamine) or thermal stimuli that produce reports of pain or itch activate populations of slowly conducting fibers.83,84,85,86,87,92 Such investigations have provided a number of insights:
Although the activation of fibers conducting at velocities corresponding to Aδ- and C-fibers is a prerequisite for evoking somatic pain in humans, activity in all slowly conducting fibers is not uniquely associated with a pain event. Populations of slowly conducting afferents communicate information on warmth, cooling, or muscle pressure,35,39 stimulus conditions that are not normally aversive. This heterogeneity is emphasized in Table 32-2, where the classes of afferents (as defined by conduction velocity) are correlated with the categories of “natural” stimuli that result in their excitation.
Electrical or mechanical stimulation sufficient to activate a slowly conducting component of a compound action potential and evoke a pain event will also evoke activity in rapidly conducting afferent fibers.
Although these observations indicate that the pain report may be obtained in the absence of large fibers, they cannot absolutely exclude them from a role in characterizing the pain event. Thus, prolonged intense mechanical stimuli will give rise to an initial burst of activity in low-threshold afferents which, unlike in the small-diameter fibers, is not sustained. High-frequency stimulation of the sural nerve, at an intensity at which the electrical stimulus was just sufficient to generate a rapidly conducting volley (Aβ), was adequate to provoke reports of pricking pain.94 Finally, in Fabry disease, in which small-diameter fibers are damaged and the largest fibers remain functional, pain sensations remain.32,95,96
Factors Influencing Afferent Activity after Tissue Injury
As noted in the preceding section, mechanical stimuli producing extreme distortion of the skin, or thermal stimuli greater than 42°C to 48°C, will evoke a stimulus-dependent activation of small sensory afferents and a correlated increase in pain behavior. Although such stimuli constitute an important element in the sensory environment of the organism, it is clear that, following tissue injury, there appears a persistent low level of discharge in these small afferents, and, not infrequently, these axons will show an exaggerated response to a subsequent mechanical or thermal stimulus. An example of such an altered stimulus–response relationship is shown in Figure 32-6.97,98 Of particular interest, systematic studies have revealed that it is often possible to identify slowly conducting afferents with thresholds that exceed the maximum possible mechanical stimulus. In the face of inflammation, however, such axons have
been shown to develop spontaneous activity and an exquisite sensitivity to subsequent mechanical stimulation. These axons, frequently found in joint afferents (see following), have been designated as “silent nociceptors.”99 These observations, in conjunction with the observed chemical sensitivity of the small afferent, suggest that the contents of the “inflammatory soup”100 that has been identified in inflamed tissue may play an important role in the afferent message generated in the post-injury pain state. There are two components to this consideration: (a) the nature of the materials that are elaborated and (b) the effect of these products on afferent activity and pain behavior.
been shown to develop spontaneous activity and an exquisite sensitivity to subsequent mechanical stimulation. These axons, frequently found in joint afferents (see following), have been designated as “silent nociceptors.”99 These observations, in conjunction with the observed chemical sensitivity of the small afferent, suggest that the contents of the “inflammatory soup”100 that has been identified in inflamed tissue may play an important role in the afferent message generated in the post-injury pain state. There are two components to this consideration: (a) the nature of the materials that are elaborated and (b) the effect of these products on afferent activity and pain behavior.
The Post-Injury State
A common result of a high-intensity thermal or mechanical stimulus is tissue damage. This state is defined by Lewis101 as a “triple response”: a flush at the site of the stimulus accompanied by a flare resulting from widespread arterial dilation and a local edema secondary to increased vascular permeability. This state of inflammation is often accompanied by a local decrease in the magnitude of the stimulus required to elicit a pain response, that is, a primary hyperalgesia,102,103 referred to by Lewis101 as “nocifensor tenderness.” The region of primary hypersensitivity is often surrounded by a much larger region of secondary hypersensitivity101 (Fig. 32-1). Evidence that at least a fraction of these phenomena were mediated by a peripheral mechanism derives from the following observations: (a) antidromic electrical activation of sensory afferent fibers produces hypersensitivity and flare in the skin region innervated by the nerve, and (b) blockade of the nerve central to the site of antidromic stimulation does not block the hypersensitivity evoked by such nerve stimulation.104 Because the antidromic volley evokes a primary hyperalgesia and vasodilation in the absence of sympathetic innervation, and the required stimulation intensities evoke discharges in C fibers, it appears likely that the effects are mediated by unmyelinated somatic afferents.104,105,106 Lewis104 presciently suggested that primary hypersensitivity was due to the release of algogenic agents from damaged tissue and from nerve terminals in the skin by means of an axon reflex activated by the proximate tissue damage. In this case, it is suggested that action potentials evoked in the terminals of a sensory fiber located in the damaged regions travel not only back to the spinal cord, but also antidromically into the surrounding vascular bed by means of axon collaterals. This is associated with the release of a vasodilator agent that increases blood flow (thereby producing the flare), increases vascular permeability (producing edema), and, as a result of either a direct effect or a subsequent release of an intermediate agent, activates or facilitates the activation of peripheral sensory afferent terminals (hypersensitivity). The local release of chemical intermediates, as just suggested, may also explain the occurrence of continued sensation after the primary stimulus (e.g., thermal or mechanical) has been removed. Thus, mild heat damage to the receptive fields has been shown to produce significant increases in the excitability of polymodal nociceptors (C-fibers, see following)107,108,109,110 and high-threshold mechanoreceptors.111 As noted earlier, the parodoxical discharges of cold receptors to noxious heat also display sensitization.112 A chemical intermediary with a prolonged half-life that alters the environs of the adjacent nerve terminals and facilitates their activity could explain one component of the accompanying hyperalgesia.
Agents Released after Injury and Their Action on Afferents and Behavior
Endogenous agents that may mediate these algogenic and facilitatory effects can be categorized according to their chemical class and their presumed origin, for example: tissue (serotonin, histamine, potassium, H+, members of the arachidonic acid [AA] cascade), plasma (kinins, adenosine), or nerve terminals (SP, CGRP). This listing is meant only to be representative and is neither inclusive nor exclusive.113 Nevertheless, it provides some sense of the complexity of the chemical milieu that occurs after a local tissue injury (Figs. 32-4 and 32-7).
Potassium/hydrogen
Tissue injury results in prominent release of intracellular potassium (K+) and a lowering of tissue pH. Following intense exercise or burns, for example, extracellular K may reach high mM concentrations.114,115 Blister base fluid and the pH of the wound environment may display pH values as low as 4 or 5 in cardiac ischemia, fracture-related hematomas, and exercising muscle.116 The mechanisms of this increase probably reflect failure of cell membrane integrity and
dysfunction of the Na/K pump function.114 A population of C-nociceptors sensitive to noxious intensities of mechanical and thermal stimuli also responds in a stimulus-related fashion to solutions of increasing proton concentration injected into their receptive fields. These receptors develop a lower threshold and enhanced response to mechanical stimuli. Similar injections in humans induce a sustained graded pain and hyperalgesia. Increasing evidence suggests that agents such as capsaicin may interact directly with peripheral terminal membranes to increase proton conductance.
dysfunction of the Na/K pump function.114 A population of C-nociceptors sensitive to noxious intensities of mechanical and thermal stimuli also responds in a stimulus-related fashion to solutions of increasing proton concentration injected into their receptive fields. These receptors develop a lower threshold and enhanced response to mechanical stimuli. Similar injections in humans induce a sustained graded pain and hyperalgesia. Increasing evidence suggests that agents such as capsaicin may interact directly with peripheral terminal membranes to increase proton conductance.
Amines
Histamine (granules of mast cells, basophils, and platelets) and serotonin (mast cells and platelets) are released by mechanical trauma, heat, radiation, and certain by-products of tissue damage, most notably neutrophil lysosomal materials, certain immunologic processes, thrombin, collagen, and epinephrine, as well as by lipid acids of the AA cascade, such as the leukotrienes; the prostaglandins (PG),117,118,119,120 histamine, and acetylcholine have all been shown to excite primary afferents. Close intra-arterial injection of serotonin excites populations of cutaneous mechanoreceptive and high-threshold afferents.121,122 Similar administration of histamine was shown to excite populations of C-fiber/group IV cutaneous mechanoreceptors and nociceptors sensitive to thermal and mechanical stimuli, but not myelinated (presumably non-noxious) mechanoreceptive afferents.123,124 Administration of the phenethylamine compound 48/80, which releases histamine from mast cells, activated small-diameter fiber nociceptors.109 A variety of agents (acetylcholine, histamine, serotonin) injected into the ear of a rabbit isolated from the body except for its nerve supply evoked a reflex depressor response indicative of the activation of small-diameter cutaneous nociceptive afferents.125 Direct application of histamine or serotonin onto a blister base induced by cantharidin, or the direct injection of these agents into the skin, induced a significant pain response in humans.126,127,128 In dogs, the intra-arterial but not the intravenous injection of serotonin and histamine induced pseudo-affective responses (barking, efforts to escape, pupil dilatation)129 (Fig. 32-1), the most potent agent being bradykinin.129
Kinins
Bradykinin is synthesized by the cascade that is triggered when factor XII is activated by agents such as kallikrein and trypsin. In the course of the cascade, even more kallikrein is produced; this is converted to bradykinin by the enzyme timenogenase.130,131 Bradykinin is released by noxious insult.2,132,133 The local injection of bradykinin has been shown to have potent stimulatory effects in small afferents.122,134,135,136 After intra-arterial injection in the dog or application in the blister base in humans,137 bradykinin produces potent pseudo-affective pain behavior. This activity in producing pain behavior and the elaboration of bradykinin during the injury state has led to considerable speculation as to its role in sustaining post-injury evoked activity. Pharmacologic investigations have emphasized the existence of two bradykinin receptors (B1/B2138). It appears that, under normal circumstances, pharmacologic studies have indicated a preponderance of B2 sites, which can activate small afferents, whereas in the face of continued inflammation, an upregulation of B1 sites appears to occur.139,140
Lipidic acids
Prostanoids are synthesized upon the release of cell membrane–derived AA by the activation of phospholipase A.141 Various agents, including norepinephrine and dopamine, stimulate the synthesis of cellular phospholipids by releasing
nonesterified free fatty acid precursors.142,143 Membrane-bound enzymes, lipoxygenase144 or cyclooxygenase (COX), act on these substrates to synthesize the leukotrienes and the prostanoids. Agents such as acetylsalicylic acid and indomethacin inhibit COX145,146,147 and prevent the synthesis of these agents. Elevated levels of PGs and leukotrienes are found following local injury and inflammation in joints and damaged skin.133,148,149 Local intra-arterial bradykinin will enhance the formation and release of PGs.150,151,152,153 Prostaglandins injected alone evoked little pain response except in high doses.154,155,156 Although these prostanoids commonly have little evocative effect, they readily facilitate the pseudo-affective and autonomic responses produced by intra-arterial administration of bradykinin in dogs and rabbits.125,155,157 Thus, in the knee joint, PGI2 will excite and facilitate the responsiveness of slowly conducting joint afferents.136 In the behaving animal, intradermal injections of PGEI augmented the pain evoked by peripheral injections of bradykinin and histamine,158 the writhing response evoked by intraperitoneal phenylbenzylquinone,159,160 and the response to pressure in the rat’s inflamed paw161,162 and on the dog’s inflamed knee joint.157 Perfusion of the receptive field of a thermal nociceptive afferent with PGE2 did not alter its resting discharge. However, PGE2 produced a dose-dependent increase in the discharge rate of the fiber in response to a stimulus.163 Similarly, the simultaneous administration of PGE1 and bradykinin, each in a dose that by itself was ineffective, produced significant activity in afferent fibers.164 That some or all of bradykinin’s algogenic effects may be mediated or potentiated by PGs is suggested by a nociceptive response to the kinin being blocked by prior treatment with a COX inhibitor.155,157,165 If the PGs produce their sensitizing effects by a common biochemical mechanism in a different species and several organ systems, a common order of potency for the lipidic acids should be observed. Where examined, the following rank order has, in fact, been typically observed: PGE1 >PGE2 >PGF2a, 2 PGF2B 2 PGA1 = PGB2 = PGI2 = 0.125,155,157,162,166
nonesterified free fatty acid precursors.142,143 Membrane-bound enzymes, lipoxygenase144 or cyclooxygenase (COX), act on these substrates to synthesize the leukotrienes and the prostanoids. Agents such as acetylsalicylic acid and indomethacin inhibit COX145,146,147 and prevent the synthesis of these agents. Elevated levels of PGs and leukotrienes are found following local injury and inflammation in joints and damaged skin.133,148,149 Local intra-arterial bradykinin will enhance the formation and release of PGs.150,151,152,153 Prostaglandins injected alone evoked little pain response except in high doses.154,155,156 Although these prostanoids commonly have little evocative effect, they readily facilitate the pseudo-affective and autonomic responses produced by intra-arterial administration of bradykinin in dogs and rabbits.125,155,157 Thus, in the knee joint, PGI2 will excite and facilitate the responsiveness of slowly conducting joint afferents.136 In the behaving animal, intradermal injections of PGEI augmented the pain evoked by peripheral injections of bradykinin and histamine,158 the writhing response evoked by intraperitoneal phenylbenzylquinone,159,160 and the response to pressure in the rat’s inflamed paw161,162 and on the dog’s inflamed knee joint.157 Perfusion of the receptive field of a thermal nociceptive afferent with PGE2 did not alter its resting discharge. However, PGE2 produced a dose-dependent increase in the discharge rate of the fiber in response to a stimulus.163 Similarly, the simultaneous administration of PGE1 and bradykinin, each in a dose that by itself was ineffective, produced significant activity in afferent fibers.164 That some or all of bradykinin’s algogenic effects may be mediated or potentiated by PGs is suggested by a nociceptive response to the kinin being blocked by prior treatment with a COX inhibitor.155,157,165 If the PGs produce their sensitizing effects by a common biochemical mechanism in a different species and several organ systems, a common order of potency for the lipidic acids should be observed. Where examined, the following rank order has, in fact, been typically observed: PGE1 >PGE2 >PGF2a, 2 PGF2B 2 PGA1 = PGB2 = PGI2 = 0.125,155,157,162,166
Adenosine
Cytokines
Injury and the evolution of the inflammatory state, or local skin injury, will lead to the release into the local extracellular fluid of a number of cytokines such as epidermal growth factor, tumor necrosis factor (TNF)-α, and a number of interleukins (IL), including IL1 and IL8.169,170,171 It appears certain that their local interactions can lead to the release of PGs, which can subsequently activate small afferents. This interaction provides mechanisms whereby general immune reactions can be affiliated with activity in small afferents (such as, perhaps, the aching sensation associated with influenza, or the reaction to foreign bodies, as in chimeric antibody therapy for cancer).
Proteinases
Thrombin or trypsin is released from macrophages and mast cells after local injury. These peptidases can cleave tethered peptide ligands that exist on the surface of small primary afferents. These tethered peptides act upon adjacent receptors (proteinase-activated receptors [PARs]) that can serve to depolarize the terminal, causing an orthodromic input and local release of SP and CGRP into the injured tissues.172,173 These receptors are present on both terminals of the primary afferents modulating the spinal as well as the peripherral sensitivity of the afferent terminal.174
Growth factors
Nerve growth factor (NGF) is upregulated in a wide variety of inflammatory conditions in inflammatory cells and Schwann cells and, when released, acts upon TrkA), which is expressed on the terminals of primary afferents. The Trk receptor activates several intracellular signaling cascades, which increase the nociceptor’s sensitivity and acts through retrograde transport to initiate delayed and persistent transcriptional processes. This is likely responsible for upregulation of the sensory neuropeptides and neuromodulators (e.g., CGRP, SP, brain-derived neurotrophic factor [BDNF]), receptors (e.g., TRPV1, P2X), and ion channels (e.g., NaV). With local inflammation, NGF leads to an upregulation of BDNF in TrkA-expressing sensory neuron. Brain-derived neurotrophic factor itself can be released and exert downstream effects.175
Afferent peptides
Substance P and CGRP are peptides found in peripheral afferent C-fiber terminals in the skin,176,177,178 cortical blood vessels,179,180 tooth pulp,181 and eye.182 Peptide levels in the peripheral tissues are typically elevated in inflamed tissues and joints.183 Antidromic sensory stimulation has been shown to release SP or CGRP in a variety of peripheral tissues, including tooth pulp,184 knee joint,185 skin,186 and trachea.187 Importantly, a stimulus that serves to activate the nerve terminal will itself yield the release of the afferent peptide.187
With regard to the effects of the afferent peptides, SP has been shown not to be algogenic when administered peripherally,188 and will not activate peripheral nociceptive afferents.189 However, it has been shown that conditioning a nerve terminal with SP will result in a facilitation of the response of the terminal to subsequent chemical mediators.190 Aside from a direct terminal interaction, locally released afferent peptides can serve to alter the local milieu. However, antidromic afferent nerve stimulation will produce flare and sensitization in the region of skin innervated by the stimulated sensory nerve.191 Exogenously administered SP has been shown to induce plasma extravasation in both normal and denervated skin,188 and because other peptides, such as vasoactive intestinal peptide (VIP), do not produce such extravasation,176 SP appears to be a likely mediator for the neurogenically evoked increase in capillary wall permeability. Administration of capsaicin interacts with the TRPV1 receptor present on the terminals of SP- and CGRP-containing C-fibers. This binding will activate and then frequently destroy these terminals. Accordingly, a subsequent depletion of SP/CGRP content occurs in skin.176 This peptide depletion is accompanied by a loss of the ability of peripheral nerve stimulation to produce extravasation.192,193 Exogenously administered SP/CGRP or antidromic stimulation of the sensory afferent evokes an increase in local blood flow and capillary permeability. The effects of either manipulation are reversed by these peptide antagonists.194,195 These observations reflect mechanisms that may be quite common. Thus, the SP/CGRP in plexuses surrounding cerebral vessels and those which originate in the trigeminal ganglion may be released and occasion the vascular component of migraine.180 Aside from their effects upon the vasculature, the locally released peptides may serve to bring into play a number of systems that may subsequently interact with the afferent terminal. Thus, antidromic stimulation and SP can degranulate mast cells and evoke kinin release.196 As noted previously, the peptidergic primary afferent terminal in the skin and joint is frequently in close apposition with both blood vessel and mast cells (Fig. 32-2).
Sensory Innervation Excited by Natural Stimuli
Table 32-2 presents a summary of the classes of afferents activated by various physical stimuli capable of evoking pain
behavior. In the following section, characteristics of these afferents as a function of their respective innervated organs are discussed.
behavior. In the following section, characteristics of these afferents as a function of their respective innervated organs are discussed.
Cutaneous Stimuli
The cutaneous input is richly innervated by afferents that transduce a variety of stimulus modalities.
Mechanosensitive afferents
High-threshold mechanoreceptive fibers responding only at pressure sufficient to produce damage to innervating glabrous tissue and conducting in the range of Aδ-fibers (15–25 m/sec) have been identified.39,122,197,198,199 These afferents tend to respond with a rate of discharge proportional to the magnitude of the pressure applied. Receptive fields for these mechanoreceptors are large in the trunk (1–8 cm200) and smaller on the face (1–2 cm200); on the limbs, distal receptive fields tend to be smaller than proximal fields. Mechanoreceptors conducting at C-fiber velocities have been shown with thresholds requiring von Frey hair stimuli greater than 2 g and with receptive fields ranging from small (5 mm200) to strips covering several square centimeters. These fibers discharge with a frequency that is monotonically proportional to the stimulus intensity.109,198
Thermoreceptive afferents
Warm receptors with small peripheral receptive fields (<0.5 mm in diameter) have been observed that respond to temperature increments of less than 1°C within the range of 30°C to 40°C.201 These fibers have also been shown to respond to thermal stimuli of noxious intensity with an increasing frequency of discharge. High-intensity stimulation within the range of 47°C to 51°C evokes a transient high-frequency discharge. These response characteristics are to be differentiated from those reported for the mechanical–thermal receptive units (see following) in that the response rate commonly is all or none in character.202,203 Certain afferent cutaneous fibers that respond to decreases in temperature on the order of less than 1°C (i.e., “cold” receptors) may show a paradoxical response to heat. As skin temperature rises to 45°C to 52°C, the fiber’s rate of discharge also increases.112,204,205,206
Mechanothermoreceptive nociceptive afferents
Afferents have been reported to respond to high-intensity mechanical and thermal stimulation, conduct within an Aδ range (10–40 m/sec), and exhibit a positively accelerating monotonic stimulus response function.39,121 The activation threshold may lie between 40°C and 60°C, and a maximum response is commonly observed at 45°C to 53°C.36,198,202,203 Receptive fields for these units are small (<5 mm200) and frequently occur as several spots, suggesting extensive collateralization of the terminals. These afferents may mediate the “first” pain of heat, for example, the pricking sensation reported immediately after the application of a strong thermal stimulus.207
Polymodal C-fiber afferents
A major portion of C-fiber afferents that respond to nociceptive stimuli are “polymodal” in character. These fibers may constitute 80% to 90% of the primate nociceptive C-fiber population.108 These axons are activated equally well by several classes of stimuli, including mechanical (>1 g), thermal (45°–53°C), and frequently chemical stimuli applied to the characteristically small (<3 mm200) receptive field. The response to such ongoing stimuli is a sustained, vigorous discharge, in which the frequency is monotonically related to stimuli intensity.107,108,109,208
Recordings in humans from single afferents conducting in the range of 0.5 to 2.0 m/sec have been made from sensory nerves innervating nonglabrous skin. These fibers are activated by strong mechanical stimuli (von Frey hairs of 0.7–13 g; needle pricks; and local compression) and produce an ongoing discharge that adapts slowly in the presence of the sustained stimulus.83,84,85,86,87,92,93 Such units are not spontaneously active at temperatures up to 40°C, but temperatures in excess of 45°C evoke activity in an increasing number of units.83,92,93 Local application of acid, histamine, or potassium chloride evokes a prolonged discharge.83,92,93 Receptive fields of polymodal C afferents tend to be larger in humans (1 mm–1 cm200) but frequently may also display several sites, suggesting extensive collateralization.83,85,87,92 These slowly conducting fibers, presumably unmyelinated in character, show clear signs of fatigue and failure of conduction upon repeated high-frequency electrical stimulation.84,86
Skeletal Muscle
It has been reported that, excluding the stretch receptors, the principal sensory innervation of the skeletal muscle derives from free nerve endings in fascia and the adventitia of blood vessels that arise from myelinated and nonmyelinated fibers.209 High-threshold groups III and IV mechanoreceptors in muscle activated by intense contraction have been reported.189,210 Intense thermal stimuli applied to muscle belly evokes monotonically increasing activity in these afferents.211 Hypertonic solutions of sodium chloride212 and close intra-arterial injections of a number of algogenic agents (histamine, bradykinin, and serotonin) evoke significant activity in afferent groups III and IV.124,135,213 However, the terminals are not functionally homogeneous. At least three groups of afferents have been identified: (a) those activated by algogenic agents but not by muscle activity, (b) those activated by muscle activity but not by algogenic agents, and (c) those activated by both sustained muscle activity and algogenic agents.214 The sensitivity to chemical agents makes it likely that these nociceptive groups III and IV afferents are activated by agents that are released locally during muscle contraction. Extracellular K levels double (5–15 mM) during isometric testing in the cat.215 Failure of nominal concentrations of phosphate and lactate to alter group IV afferent activity suggests that metabolic by-products alone do not constitute a source of muscle pain.214 The apparent ability of PGs to sensitize algogenic receptors to otherwise inactive concentrations of chemical stimulants in cardiac muscle (see following), and the likelihood of their release during intense skeletal muscle contraction, could be a significant factor in the activation of groups III and IV skeletal muscle afferents by metabolic by-products.
Cardiac Muscle
The heart receives afferents that travel with both the parasympathetic and the sympathetic tree. In the latter, the cell bodies are in the nodose ganglia, and in the former, the cell bodies are in the respective thoracic dorsal root ganglia. Pain arising from the heart appears to be mediated to a significant degree by activity in the sympathetic afferents, but vagal afferents may play an important, if undefined, role.216 Occlusion of the coronary artery leading to ischemia of ventricular muscle produces pain in humans217,218 and pseudo-affective response in animals.219 Sensory afferents in the inferior cardiac nerve projecting to the T1–T5 segments of the spinal cord mediate the transmission of such information.220,221,222,223 Recording single units from the T2–T3 ramus communicans reveals that, during coronary occlusions, activity in Aδ/C afferents increases.224,225,226 The cardiac afferents are activated by moderate- to high-intensity mechanical stimulation,225,227,228 noxious heating,229 and several algogenic agents.229,230,231 The stimulus for angina may be the release from ischemic muscle of humoral factors that sensitize or activate cardiac afferents. Serotonin and histamine stimulate cardiac afferent fibers,229,232,233 and blood levels of bradykinin in the
coronary sinus after occlusion are sufficient to evoke pseudo-affective responses when administered in dogs.129,226,234,235 In the heart, reflex cardiovascular changes suggestive of angina discomfort occur following the topical application of several algogenic agents to the wall of the left ventricle, and temporary occlusion of the coronary artery supplying the area of the ventricle under study sensitizes the heart to the algogenic effects of bradykinin.209 Importantly, coapplication of PGs (PGEI >PGE2 >PGF2a) with bradykinin has significantly potentiated the algogenic effects of bradykinin. Pretreatment with a PG synthesis inhibitor reduced the algogenic effects of bradykinin and the sensitizing effects of prior ischemia. The potentiation of bradykinin’s algogenic effects by exogenous PGs was evident for as long as 60 minutes and could not be attenuated by indomethacin. This is consistent with the observation that PGs are formed and released in the heart muscle following hypoxia or ischemia.236,237,238 Thus, angina could speculatively result, in part, from the neurohumoral activation of afferent terminals by the joint release of both PGs and bradykinin.
coronary sinus after occlusion are sufficient to evoke pseudo-affective responses when administered in dogs.129,226,234,235 In the heart, reflex cardiovascular changes suggestive of angina discomfort occur following the topical application of several algogenic agents to the wall of the left ventricle, and temporary occlusion of the coronary artery supplying the area of the ventricle under study sensitizes the heart to the algogenic effects of bradykinin.209 Importantly, coapplication of PGs (PGEI >PGE2 >PGF2a) with bradykinin has significantly potentiated the algogenic effects of bradykinin. Pretreatment with a PG synthesis inhibitor reduced the algogenic effects of bradykinin and the sensitizing effects of prior ischemia. The potentiation of bradykinin’s algogenic effects by exogenous PGs was evident for as long as 60 minutes and could not be attenuated by indomethacin. This is consistent with the observation that PGs are formed and released in the heart muscle following hypoxia or ischemia.236,237,238 Thus, angina could speculatively result, in part, from the neurohumoral activation of afferent terminals by the joint release of both PGs and bradykinin.
Teeth
Innervation of the teeth occurs both intradentally (within the tooth) and periodontally (within the surrounding connective tissue). Free nerve endings are found within the pulp and the surrounding blood vessels. Fibers that originate in afferent plexuses adjacent to the inner dentinal surface pass through the odontoblasts to the dentinal tubules that run parallel to the odontoblast processes.239,240 In tooth pulp, the unmyelinated fibers are ensheathed by Schwann cells and interlace with the odontoblast somata. Tooth pulp afferents consist of fibers having diameters and apparent conduction velocities in the Aδ- and C-fiber range.241,242,243,244 The periodontal afferents travel through the maxillary and mandibular branches of the trigeminal nerve to terminate within collagen fibers of the alveolar ligament, with specializations similar to the Meissner corpuscle. Transdentinal electrical stimulation produces a sharp pain of brief duration,10 which gives rise to jaw opening and closing reflexes and attempts to escape.245,246,247 In shock titration paradigms, primates maintain the current intensity of dental stimulation at or immediately above that intensity which, in a single escape paradigm, will support escape behavior.248 These observations correspond to studies in humans suggesting that the difference between a perceivable threshold stimulus (prepain) and a stimulus sufficient to evoke a pain report is small.48,49 Conversely, activity in intradentinal sensory nerves has been shown to correlate closely with the sensation of pain.249 Pressure, touch, or tooth movement is likely to be mediated by afferents from the periodontal and gingival structures in which the tooth is embedded.250,251
Thermal stimulation of teeth has been reported to evoke neuronal activity in dental nerves.252,253 In cats, the frequency of discharge of dental afferents rose when the dentin temperature was elevated from 34°C to 37°C within a 10-second period.254 Slow heating of the cat tooth surface to 47°C failed to elicit such activity.255 Repeated intense thermal stimulation of the tooth (60°C), however, did evoke a persistent afferent discharge. Pretreatment, but not posttreatment, with prostaglandin synthesis inhibitors reduced the discharge associated with repetitive heating.256 The generation of COX products may therefore sensitize nerve endings to stimuli originating in the local pulpal environment. Because nerve endings do not penetrate to the enamel dentin interface, the effective stimulus in tooth sensations has been proposed to be the distortion of odontoblasts or alterations in hydraulic flow produced by changes in temperature.257 Inflammation or edema associated with thermal damage, as just described, or direct pressure could increase pulpal volume and deform the mechanically sensitive nerve terminals that lie between the odontoblasts. That changes in pulpal pressure can evoke activity in tooth afferents is supported by the observations that (a) bursts of spikes occur in synchrony with the systolic pulse and (b) pulpal pressure changes as much as 5 mm Hg with the systolic pulse pressure in the normal tooth.254,258 Presumably, where inflammation has occurred, such changes may be augmented because of the increased pulpal volume in a restricted space.
Synovial Joints
Cutaneous afferents and branches of adjacent muscle afferents innervate the joints.259 Intense deformation or inflammation-induced expansion within the joint will evoke activity in the group III (Aδ) fibers.260,261,262 Urate crystals,263 carrageenan,157,264 and endotoxin265 injected into the synovial joint evokes an acute local inflammation, whereas the intradermal injection of killed bacteria suspended in Freund adjuvant results in a chronic polyarthritis.266 A marked sensitivity to stimulation of the affected limbs is observed in animals so treated. Joint pain associated with gout-induced arthritis in humans probably results from urate crystal deposition.267 Altered sensitivity to mechanical stimulation may be mediated by the generation of chemical intermediaries such as PGs. The ability of COX or phospholipase A inhibitors to reduce the sensitivity associated with the inflamed joints and to reduce the inflammation is consistent with this hypothesis.264,268 Other inhibitors of COX are effective against joint pain but exert no effect on arthritic swelling.269,270 This has led to the speculation that nonsteroidal anti-inflammatory drugs (NSAIDs) may either work by a separate mechanism271 or be associated with a central action.272,273
Visceral Organs
Information regarding the state of the visceral organs projects to the CNS by afferents that travel with both parasympathetic (essentially vagal above the diaphragm and sacral parasympathetics for the abdominal viscera) and sympathetic innervation.274 For the vagus, the cell body of the afferent lies in the nodose ganglion, whereas for the sympathetic and sacral parasympathetic, the afferent cell body lies in the respective DRG. Many of the axons are small and lightly myelinated or unmyelinated.216,275,276 Manipulation of a number of healthy visceral organs, such as the liver, kidney, and spleen, does not appear to give rise to reports of pain. However, it appears likely that three conditions associated with visceral stimuli lead to a pain report:
Distention of hollow viscus. Thus, expansion of the gastrointestinal tract (esophagus, stomach duodenum, large and small intestines), smooth muscle organs (gallbladder), and urinary tract (urethra and bladder) will yield short-latency pain behavior in animals and humans.276,277
Inflammation. Internal body organs clearly become symptomatic in the presence of mechanical distortion and particularly during inflammation. Based on the ability of some of the inflammatory products outlined earlier, such as bradykinin and PGs, to induce activity in visceral innervation and to induce pain behavior,209,278,279,280,281 such a correlation appears reasonable.
Ischemia. Occlusion of the vasculature is recognized as a potential source of visceral pain. Ischemia induces activity in visceral afferents282 and results in a septic shock state in which large quantities of active products are released locally and into the circulation (e.g., heart—coronary; viscera—splanchnic). As in the earlier comments on inflammation, the presence of these products can both facilitate and activate the transduction properties of the innervating afferent.
Origins of Abnormal Activity in Peripheral Afferents
Commonly, orthodromic discharge of a peripheral afferent originates at the distal terminals secondary to generator potentials induced by the appropriate peripheral stimulus. Pressure briskly applied mid-axon evokes only a transient neural activity (Fig. 32-8).283,284 Acute nerve compression in humans may be reported as transiently painful, if perceived at all.285,286 Simple, slow distortion of mid-axon regions, however, is ineffective in generating neural activity.200,284,287,288,289 Thus, chronic pressure alone, such as that generated by benign tumors, is often not reported as painful.286 However, mechanical insensitivity of the mid-axon region is altered after nerve injury.
Upon severance of a nerve, a barrage of activity occurs that then disappears. The injured axon undergoes retrograde chromatolysis (dying back). Over an interval of days to weeks, the injured axon initiates sprouting.290 Collections of these sprouts form a neuroma. Systematic studies in animal models have shown that this neuroma frequently gives rise to ongoing orthodromic activity in the nerve. The activity occurs within fibers having conduction velocities suggestive of large- and small-diameter myelinated and unmyelinated fibers284,291 and is characerized by patterns of spontaneous and bursting activity in the sprouting axon.292 Small distortions of the neuroma now produce long periods (30 seconds) of repetitive discharge. These observations suggested that the local injury had transformed that region of the axon and endowed it with properties of excitability similar to those in the terminal region. Importantly, it has been shown that, after such peripheral nerve injury, the DRG cell of the injured nerve also begins to display a spontaneous activity.291,293
The origin of the spontaneous activity appears to reflect several mechanisms:294
Increased expression of NaV channels in the neuroma and DRG of the injured axon. Following nerve injury, an increased expression of a variety of NaV channels occurs, particularly those that are TTX-resistant.295,296 Increased ionic conductance of the neuroma may yield the ectopic activity observed in these regenerating axons. Support that this is indeed the case is provided by the observation that systemic lidocaine at doses that do not block conduction, block ectopic activity in the neuromas and DRG.293
Potassium channel activity. An alternate possibility leading to ectopic activity is a reduction in K+ channel activity. Such a change would similarly contribute to an increased afferent excitability.297,298
Altered chemosensitivity. Following nerve injury, application of a variety of amines299,300 and proinflammatory cytokines (IL-1β, TNFα) to neuromas leads to the initiation of axon activity.301 An important component of the local milieu after nerve injury is the release from local inflammatory and Schwann cells of cytokine such as I-1β and TNFα. Support for the potential role of such cytokines in nerve injury hyperpathia is provided by the observation that local delivery of IL-1β and TNFα receptor antibodies reduces indices of hyperpathia following nerve injury.302,303 Growth factors, notably NGF, is also upregulated at the injury site after nerve injury.175 As reviewed earlier, this neurotrophin may be internalized and transported to the DRG cell body, where it initiates transcription of a wide range of transmitters, receptors, and channels.
Ectopic foci in these injured nerves have been suggested to be the source of the abnormal sensations that occur after amputation.304,305 Neurotomy in several species, including mice, rats, cats, and rabbits,284,306,307 gives rise to autotomy of the denervated region, which may result from the abnormal discharges generated by the neuroma formed. The self-mutilation, when and if it does develop, apparently is not due to the anesthetic state, but begins only after several days.308 Guanethidine, which suppresses the ectopic discharges associated with neuromas in mice, suppresses autotomy in neurectomized rats and mice.309 Selective spinal tractotomies abolish the autotomy.306
Dorsal root ganglion cells are apparent exceptions to the this discussion of nonterminal afferent spike generation. As shown in Figure 32-8, slow, minor distortion of the DRG evokes repetitive firing that lasts minutes. Similar distortion of the dorsal roots was not as effective.156 It has been proved that this
stimulation evoked a pain event by the fact that acute injury to the DRG evokes immediate tachycardia and mass flexion reflexes in the lightly anesthetized animal. These observations support the suggestion that the radicular pain of sciatica might be associated with such a focal distortion of the DRG and not the root or nerve proper. Patients suffering from tic douloureux are often reported to have an artery, small tumor, or plaque impinging upon the trigeminal root.310,311,312 Although such mechanical stimulation may not account for the unique sensory barrage associated with tic episodes, the mechanical sensitivity of the dorsal root may provide a background upon which a mechanical stimulus, such as that provided by the adjacent artery, might alter innocuous sensory input and thus generate the pain event.313,314,315
stimulation evoked a pain event by the fact that acute injury to the DRG evokes immediate tachycardia and mass flexion reflexes in the lightly anesthetized animal. These observations support the suggestion that the radicular pain of sciatica might be associated with such a focal distortion of the DRG and not the root or nerve proper. Patients suffering from tic douloureux are often reported to have an artery, small tumor, or plaque impinging upon the trigeminal root.310,311,312 Although such mechanical stimulation may not account for the unique sensory barrage associated with tic episodes, the mechanical sensitivity of the dorsal root may provide a background upon which a mechanical stimulus, such as that provided by the adjacent artery, might alter innocuous sensory input and thus generate the pain event.313,314,315
Spinal Terminals of Primary Afferents
Dorsal Root Entry Zone
As the dorsal root approaches the spinal cord, small myelinated and unmyelinated fibers tend to aggregate in the lateral aspect of the dorsal root, and larger myelinated fibers aggregate medially (Fig. 32-9).316,317,318,319,320 Entering the spinal cord, the primary afferents bifurcate into rostrally and caudally projecting branches. Large fibers proceed along the dorsal columns for varying distances; many terminate in the spinal gray matter.321,322 Large-diameter afferent collaterals exit from the dorsal column axis perpendicularly and pursue a ballistic trajectory, coursing deeply into the gray matter before turning dorsally to terminate in the upper portions of the gray matter.323 The smaller-caliber fibers project rostrally and caudally one or two segments into the medial portion of the tract of Lissauer. Small-diameter fibers enter the dorsal gray matter directly and terminate dorsally, as will be discussed. Both large- and small-diameter fibers give rise to collaterals that distribute ventrally in the spinal gray matter (Fig. 32-9).324
Although the preponderance of afferents terminate ipsilaterally, evidence suggests that a proportion of the afferents also terminate contralaterally.317,325,326,327,328 These fibers travel dorsal to the central canal to terminate in laminae III and IV of the contralateral dorsal horn, forming a longitudinally oriented plexus one to two segments in length.329 These contralateral projections occur most commonly at the cervical and sacral levels of the spinal cord, although crossing fibers have been described in the lumbar cord.317,326 Early work demonstrated the behavioral significance of the medial to lateral distribution of the large and small primary afferents by making discrete lesions in the dorsal root entry zone.319 Lateral cuts, presumably severing the smaller-caliber myelinated fiber population, produced a significant blockade of the pseudo-affective response of the animal to strong, otherwise aversive, stimuli on the side where the lesions were made. It is likely that such a section also produced local infarcts of the adjacent dorsal gray matter.330 Discrete surgical lesions directed at the dorsal horn through the dorsal root entry zone have been shown to alleviate pain, particularly that associated with root avulsions.331,332
The principal portion of the sensory afferents enters the spinal cord through the dorsal root entry zone, consistent with the “law” of Bell and Magendie. However, a significant number of unmyelinated afferent fibers, which arise from DRG cells, also exist within the ventral roots.333,334,335,336,337 After the injection of horseradish peroxidase into the spinal gray matter of cats with previously sectioned dorsal roots, reaction products appeared in small DRG cells. Failure to label large DRG neurons is consistent with the observation that the ventral root afferents are largely unmyelinated.338,339 The relevance of the ventral root afferents in pain transmission remains to be fully defined. This alternate pathway may account for the failure of dorsal rhizotomies to reliably relieve pain.340 The origin of dorsal and ventral root afferents in DRG neurons would suggest that ganglionectomy would be superior to rhizotomy if afferent input is to be abolished.341,342
Afferent Terminals in Dorsal Horn
The dorsal horn was classically recognized from the days of Cajal as being divided into the dorsal root entry zone, superfical dorsal horn (marginal layer), the substantia gelatinosa, the neck of the dorsal horn (nucleus proprius), the ventral horn, and the
region around the central canal. The concept that the spinal gray matter of the adult spinal cord may be organized according to a distinctive lamination of cell bodies and terminal regions refines this gross anatomic subdivision to delimit the anatomy of the spinal gray matter (Fig. 32-10).343,344 The laminations correspond grossly with the classical.
region around the central canal. The concept that the spinal gray matter of the adult spinal cord may be organized according to a distinctive lamination of cell bodies and terminal regions refines this gross anatomic subdivision to delimit the anatomy of the spinal gray matter (Fig. 32-10).343,344 The laminations correspond grossly with the classical.
Organization of Terminals by Fiber Type
Retrograde transport of horseradish peroxidase to the distal cut ends of primary afferent fibers after medial section of the dorsal root revealed that the horseradish peroxidase reaction product was located primarily in the most dorsal laminae of the spinal gray matter. Terminals from smaller myelinated fibers were located in the marginal zone, the ventral portion of lamina II, and throughout lamina III.317 Fine-caliber unmyelinated fibers largely terminated throughout lamina II. In contrast, the large-caliber fibers passing in the medial portion of the root entry zone terminated largely in the nucleus proprius and in more ventral regions of the dorsal gray matter. These larger fibers made few direct contacts with neurons of the substantia gelatinosa and marginal layer, terminating largely below lamina II. Similar results were obtained in studies in which the distribution of functionally defined afferents was examined after intra-axonal application of horseradish peroxidase.326 Intermediate- to fine-diameter cutaneous nociceptive mechanoreceptors terminated largely in the marginal zone. Terminals of low-threshold mechanoreceptors activated by low-threhsold mechanical stimuli are distributed in the dorsal portion of laminae IV and V. These comments are summarized schematically in Figure 32-11.345
After entry into the dorsal root entry zone, the axons typically display bifurcations or trifurcations. The large afferents send projections rostrally and caudally within the dorsal
columns and directly into the dorsal horn (Fig. 32-12). The rostrally projecting collaterals may continue as far forward as the dorsal column nuclei. However, many of the axons send collaterals off into the spinal horn at progressively distal segments. Small axons display a similar collateralization, although the rostrocaudal spread occurs in the lateral tract of Lissauer.346 Systematic mapping studies have shown that the projections of the “long-ranging afferents” may extend as far as four to 11 segments. Thus, in the rat, labeled terminals from the sural nerve were found in the gray matter up to three to four segments caudal to their root entry, and for the sciatic nerve in S4, four to six segments caudal to the segment of root entry.347 Importantly, the strength of the excitatory drive is greatest at the segment of entry and diminishes so that inputs from distal dermatomes have a progressively decreasing excitatory influence.348 The significance of this large- and small-afferent collateralization is that an excitatory drive occurs at spinal segments distal to the segment of entry. Factors that regulate the postsynaptic excitability of the distal cells receiving these projections will serve to define the size of the receptive field of the respective neuron.
columns and directly into the dorsal horn (Fig. 32-12). The rostrally projecting collaterals may continue as far forward as the dorsal column nuclei. However, many of the axons send collaterals off into the spinal horn at progressively distal segments. Small axons display a similar collateralization, although the rostrocaudal spread occurs in the lateral tract of Lissauer.346 Systematic mapping studies have shown that the projections of the “long-ranging afferents” may extend as far as four to 11 segments. Thus, in the rat, labeled terminals from the sural nerve were found in the gray matter up to three to four segments caudal to their root entry, and for the sciatic nerve in S4, four to six segments caudal to the segment of root entry.347 Importantly, the strength of the excitatory drive is greatest at the segment of entry and diminishes so that inputs from distal dermatomes have a progressively decreasing excitatory influence.348 The significance of this large- and small-afferent collateralization is that an excitatory drive occurs at spinal segments distal to the segment of entry. Factors that regulate the postsynaptic excitability of the distal cells receiving these projections will serve to define the size of the receptive field of the respective neuron.
Pharmacology of Primary Afferents
Spinal Afferent Terminals
The synapse made by primary afferents with neurons of the spinal cord represents the first-order link between the periphery and the CNS. This link has several properties. Classic electrophysiologic studies provided evidence that conducted potentials interact with the second-order neuron by an excitatory synapse. No evidence of a classic monosynaptic inhibitory influence by primary afferents has been hitherto presented.349 A second property of the neuronal response evoked by afferent excitation is that at least two populations of excitatory postsynaptic potentials are often seen, which are thought to be monosynaptic: (a) fast-onset and short-lasting and (b) delayed and of a relatively extended duration.350,351,352 These electrophysiologic findings, in concert with the presence of morphologically distinct vesicle populations in the same terminal, are interpreted as revealing the presence of at least two classes of neurotransmitters released from the same terminal.
Considerable efforts have been directed at establishing the identity of neuroactive substances in the primary afferent. Several criteria are classically accepted as minimum requirements in the establishment of the correspondence between the endogenous material and a given substance: (a) the material must be found in the terminals of the afferent population in question (and it or its precursor in the respective DRG cell), (b) the material must be present in a fraction that is released when the appropriate afferents undergo depolarization, (c) the postsynaptic effects of the exogenously applied materials must mimic the effects that result when the endogenous afferent systems are physiologically activated, and (d) those physiologic effects that result from the actions of the endogenously released and exogenously administered agents must possess an identical pharmacology (i.e., the characteristics of the receptor acted upon by the endogenous and exogenous agents must be indistinguishable) (Fig. 32-13).
Primary Afferent Neurotransmitter Phenotypes
Primary afferent neurotrasmitter phenotypes may be broadly divided according to axon size, state of myelination, and cell surface markers:
Large primary afferents arising from Type A (large) ganglion cells typically display small, clear-core synaptic vesicles that contain and release excitatory amino acids (glutamate/aspartate).
The terminals of small afferents arising from type B (small) ganglion cells may display several disitnct phenotypes:
Small clear-core vesicles (containing excitatory amino). Many of these cells display the IB4 glycoprotein (e.g., IB4+)
Small clear-core vesicles and large dense core vesicles (containing excitatory amino acids and peptides, respectively). These cells are IB4–. Thus, 90% of the small DRG neurons that are immunopositive for SP, are also glutamate immunopositive.353
Peptides identified in the type B ganglion cells and distributed in the superficial (laminae I and II) dorsal horn include SP; CGRP; VIP; SST, a VIP homologue (peptido-histidine-isoleucine [PHI]); cholecystokinin (CCK); angiotensin II; and bombesin.354,355
The levels of these peptides in the dorsal horn are significantly reduced by rhizotomy or ganglionectomy. These observations are consistent with findings discussed previously, that unmyelinated afferent fibers course through the dorsal roots but also, to a modest degree, in the ventral roots.
Immunohistochemical studies directed at the dorsal horn have revealed distinguishable differences in the discrete distribution of the several peptides in the dorsal laminae. Substance
P is predominantly located in Rexed lamina I and the outer layer of II; VIP is principally found in lamina I, whereas SST is found in the outer layer of II. Fluoride-resistant acid phosphatase, found in small ganglion cells separate from those containing SP or SST,63 is distributed primarily in the inner layer of lamina II in regions distinct from those that SP, VIP, or SST project.356
P is predominantly located in Rexed lamina I and the outer layer of II; VIP is principally found in lamina I, whereas SST is found in the outer layer of II. Fluoride-resistant acid phosphatase, found in small ganglion cells separate from those containing SP or SST,63 is distributed primarily in the inner layer of lamina II in regions distinct from those that SP, VIP, or SST project.356
Ability of Putative Afferent Neurotransmitters to Be Released
Important considerations are whether the materials present in afferent terminals exist within a releasable fraction and whether the axons from which they derive are relevant to pain transmission. From spinal cord slices, SP and SST levels in the extracellular fluid have been shown to be elevated in a Ca2+-dependent fashion by depolarization.357,358,359,360,361 In vivo studies using a variety of spinal perfusion models362 have demonstrated the release of materials from primary afferents, including SP,363,364 CGRP,365 VIP, CCK,366,367 and glutamate.368,369,370 Using internalization of the G-protein-coupled neurokinin with these peptides, release was produced by stimulation that activated Aδ/C- but not Aβ-afferents. The release of SP from the spinal cord has been shown to be antagonized by the local application of μ and δ but not κ opioid agonists and by α2 agonists.371 These observations are consistent with the presence of opioid and α-adrenergic receptors on primary afferent terminals and the effects of opiates and adrenergic agonists on afferent terminals.372,373,374,375
Postsynaptic Actions
Glutamate and aspartate applied onto dorsal horn neurons result in a powerful, reversible depolarization with rapid onset, accompanied by an increase in membrane conductance.376 Examination of the subclasses of neurons excited by glutamate or aspartate reveals no functional selectivity. Thus, motor horn cells and dorsal horn neurons excited by Aβ-, Aδ-, and C-fibers show a characteristic excitatory response to glutamate administration.376,377
Iontophoretic application onto the dorsal horn of several peptides found in primary afferents has been shown to produce excitatory effects. The focal administration of SP onto spinal neurons results in a slow progressive depolarization of the cell378,379,380 mediated in large part theough a G-protein-coupled neurokinin 1 (NK1) receptor.381 Substance P will excite cells that are naturally activated by noxious radiant heat,378 strong mechanical stimuli,382 and the intra-arterial injection of bradykinin,383,384 and it facilitate responses of cells activated by noxious cutaneous stimuli. Other peptides, such as CGRP, SST, CCK, and VIP have been shown to produce excitatory and facilitatory effects on neurons that respond to a wide variety of innocuous stimuli. Calcitonin gene-related peptide may act through two subtypes of receptors.385 Locally delivered, it facilitates synaptic transmission in dorsal horn neurons, and this effect was exaggerated in spinal slices harvested from rats with inflammatory arthritis.386 Thus, there appears to be a significant correlation between the ability of SP to evoke a progressive depolarization in a dorsal horn neuron and the existence of an afferent drive from small fibers.383 Vasoactive intestinal peptide and CCK have been shown to similarly evoke excitation of dorsal horn neurons.387,388,389 Somatostatin has been shown to produce an inhibition of the activity of dorsal horn neurons after iontophoretic administration.382,390 This finding is somewhat surprising, because a monosynaptic inhibition by primary afferents on second-order neurons has not been described, but reflects the fact that these agents may exist in several systems, not in primary afferents alone.
As noted, populations of afferents, particularly those of a small diameter, have more than one material in their terminals that can be released. Electron microscopy has long indicated the presence of several morphologically distinct vesicle populations in primary afferent terminals, notably those with dense cores and now thought to contain peptides and smaller clear vesicles that may contain amino acids.391 Intracellular recording in rat spinal cord slices has revealed that repetitive stimulation of the dorsal roots results in an initial burst of monosynaptically driven spikes followed by a prolonged, slow hypopolarization.351 The slow depolarization of dorsal horn neurons evoked by afferent stimulation or focally applied SP (but not VIP) was blocked by coadministration of a putative SP receptor antagonist or by the prior administration of capsaicin, a neurotoxin that results in the depletion of afferent stores of SP and CCK and destroys small afferents marked by the cytosol enzyme fluoride-resistant acid phosphatase, but has no effect on large-diameter afferents.392,393 The early depolarization was unaffected by these manipulations. These observations suggest that, for this particular neuronal population in neonatal rat spinal cord, afferent stimulation results in two events: a delayed depolarization likely to be mediated by a population of SP-containing terminals, and an immediate depolarization mediated by a second neuroactive agent, perhaps an excitatory amino acid.355,394 More recent work has emphasized that the short-latencied primary glutamatergic excitation is mediated by one of several α-amino-3-hydroxy-5-methyl-4-isoxazolepropionic acid (AMPA)-type receptors.395
Role of Neurotransmitters in Pain Transmission
In view of the foregoing data, one may tentatively hypothesize that both the excitatory amino acids and one or more peptides may play some role in pain transmission at the level of the primary afferent synapse. The direct application of several of the peptides (SP, CGRP) and glutamate onto the dorsal horn of the spinal cord results in mild scratching behavior and signs of agitation. Importantly, given the co-containment and likely co-release of peptides and glutamate, the effect of the intrathecal agents is translated into a profound increase in behavioral signs of irritation when the peptide is coadministered with glutamate.396 The potentiating effects are reduced when this is coadministered with low doses of putative SP receptor antagonists. Aside from the direct excitation and behavioral indices of pain, the spinal delivery of several of these peptides and glutamate have been shown to produce a powerful augmentation of the animal’s response to an external stimulus. Thus, the iontophoresis of SP and glutamate will increase the size of the receptive field of the cell and enhance its response to light tactile stimulation as well as noxious thermal and mechanical stimuli.397,398 This increased spinal reactivity after spinal delivery of glutamate is accompanied by behaviorally defined thermal hyperalgesia and tactile allodynia. These effects underlie the prominent states of facilitated processing that will be discussed in the following sections.
Nociceptive Elements in the Spinal Cord
The differential distribution in the Rexed laminae398 of terminals associated with fibers that are activated by specific noxious stimuli is consistent with the existence of populations of second-order neurons relevant to the rostrad transmission of nociceptive information (Fig. 32-14).
Marginal Zone (Lamina I)
The superficial layer of the dorsal horn comprises classes of large neurons oriented transversely across the cap of the dorsal gray matter. Some cells project to the brainstem (notably the medullary reticular formation and the mesencephalic parabracheal nucleus thalamus),316,399,400,401,402 while others project intrasegmentally and intersegmentally along the dorsal and dorsolateral white matter.403,404,405 The local dendritic plexus of these spinal neurons extends up to several hundred microns along both the transverse and longitudinal axes of the cord, although the dendritic tree is largely confined to the marginal layer.323,406 As displayed schematically in Figure 32-11, afferent terminals tend to synapse distally in the dendritic tree, whereas nonafferent terminals tend to be proximal on the cell body.316,407 Lamina I neurons may be divided physiologically into three groups: (a) neurons activated by fibers having Aδ-/C-fiber conduction velocities that respond to intense mechanical stimulation (Fig. 32-14); (b) neurons activated by innocuous skin cooling, with afferents having a conduction velocity akin to those of an Aδ-fiber; and (c) a small percentage of neurons activated by C-fiber polymodal afferents.399,408,409,410,411 Although initial observations suggested that these neurons of lamina I were specifically sensitive to intense stimuli, a significant proportion of these cells also possess a wide dynamic range, that is, a response frequency proportional to the intensity of the stimulus.408 Importantly, those neurons responding to Aδ- and C-fiber input can also be activated by group III and group IV muscle afferents, indicating a convergence of muscle and cutaneous input.
The role of lamina I neurons in nocicpetion has been directly assessed through spinally delivered neurotoxin (SP; saporin) that destroys neurons that bear the NK1 receptor. In such studies, loss of these cells has been shown to produce a potent reduction in the facilitated nociceptive behavior generated by tissue injury, with little effect upon acute pain thresholds.412,413,414
Substantia Gelatinosa (Lamina II)
The clear band of neural tissue lying ventral to the marginal layer and dorsal to a region of coarser texture known as the nucleus proprius was given the name substantia gelatinosa by Rolando.415 The substantia gelatinosa, defined by gross observation in unfixed or semifixed tissue, corresponds to the laminae II of Rexed. Lamina II is divided into outer and inner layers. The former is characterized by small, densely packed cells and a neuropil made complex by the presence of a larger number of dendrites. The latter zone is similar but has a less coarse texture owing to the relative paucity of terminals. The principal cell type in lamina II is the stalk cell, with cone-shaped dendritic trees arborizing through lamina II into III and axons
branching into lamina I.404,416 The terminals of Aδ-afferents that project to this lamina are a likely source of the numerous axodendritic contacts observed in this region.55,407,417,418,419,420
branching into lamina I.404,416 The terminals of Aδ-afferents that project to this lamina are a likely source of the numerous axodendritic contacts observed in this region.55,407,417,418,419,420
A significant proportion of the substantia gelatinosa neurons receive Aδ-/C-fiber input.421,422,423,424 Neurons located in lamina II (the outer portion of the substantia gelatinosa) tend to be excited by activation of thermal receptive or mechanical nociceptive afferents.423 Neurons retrogradely labeled with horseradish peroxidase and activated by nociceptive input display dendritic branching in the outer layer of the substantia gelatinosa, whereas neurons activated by innocuous mechanical stimuli have dendritic trees in the inner layer of the substantia gelatinosa.326 Receptive fields of gelatinosa neurons responsive to peripheral stimuli are typically small (<2 cm200). Several properties of gelatinosa neurons have emerged:
Unlike those cells lying more deeply (see following), neurons of the substantia gelatinosa commonly exhibit prolonged periods of excitation and inhibition after afferent activation.424,425
Islet and stalked cells constitute several classes of functionally distinct cells, with variable degrees of background activity, in which afferent input will drive complex “on/off” responses. Significantly, those cells inhibited by non-noxious stimuli were excited by noxious input, whereas cells inhibited by noxious input were excited by non-noxious input.426,427 Such profiles suggest that the activity of substantia gelatinosa neurons is governed by a convergence of excitation arising from large- and small-fiber input acting either directly on these neurons or by inhibitory interneurons.
Nucleus Proprius (Laminae III, IV, and V)
Lamina III contains fewer neurons and a less dense neuropil than does lamina II. In addition, the islet neuron428,429 (Golgi type II) appears in high concentration, in contrast to the stalk cells that occur predominantly in the outer layer of lamina II.
Lamina IV, as defined by Rexed, is composed of a broad layer of relatively large neurons (10 to 15 μm in diameter) that endow this region with their characteristic morphology. The dendritic tree of these neurons transversely and dorsally spreads into laminae II and III. The neuropil of lamina II and IV is characterized by axodendritic and axoaxonic synapses,419,430 originating from afferent input of the large-diameter fibers that contact the apical portion of the dendritic tree,431 and from local axonal plexuses derived from intrinsic fibers.316 Lamina V, located along the neck of the dorsal horn, displays a dendritic organization that does not differ prominently from that of neurons in lamina IV.
Neurons in both lamina IV and lamina V project to the ventrobasal thalamus, mesencephalon,400,401,432,433,434 and the lateral cervical nucleus,421,435 and provide propriospinal projections within the spinal cord in various species (see following). Cells in the nucleus proprius may be broadly classed as those that respond to low-threshold (Aβ) input and those that respond at a progressively greater frequency as a function of stimulus intensity. This reflects the convergent input from several classes of functionally defined afferent types (e.g., Aβ, Aδ, and C). These dorsal horn cells are referred to as wide dynamic range (WDR) neurons.436 The latter class of cells responds to transient brush and touch but show no elevation in activity with prolonged pinch; these cells are referred to as lamina IV neurons, whereas the former class of neurons are referred to as lamina V neurons because of the early studies that localized them in that region.437 Lamina V neurons have several discrete properties that make them of particular interest to our interpretation of nociceptive mechanisms:
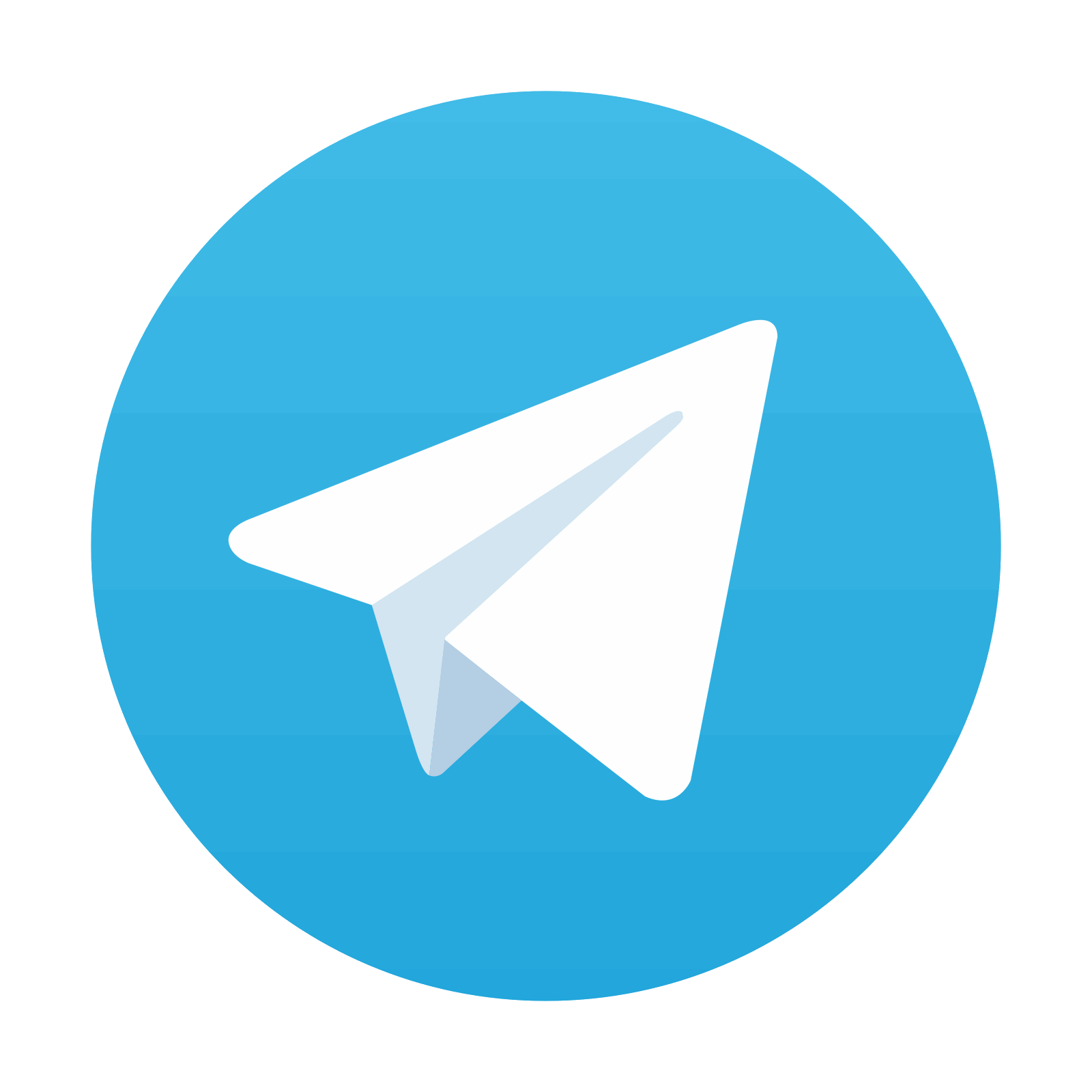
Stay updated, free articles. Join our Telegram channel

Full access? Get Clinical Tree
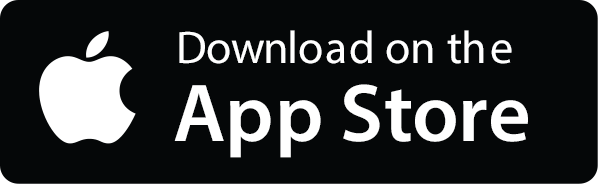
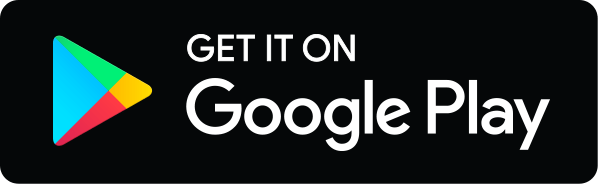