Chapter 7
Pharmacokinetics of Inhalation Anesthetics
The basic action of an anesthetic in the body is largely a function of the drug’s chemical structure and the resulting interaction with a cellular receptor complex (Figure 7-1). A number of heterogeneous compounds exhibit anesthetic properties. The inorganic molecule nitrous oxide and halogenated ethers (e.g., isoflurane, desflurane, and sevoflurane) are all capable of binding to central nervous system and spinal cord neuronal membranes to produce reversible depression. A single specific anesthetic receptor has yet to be found. In fact, multiple sites of action and protein targets probably exist; however, once a critical concentration of drug has entered the brain and spinal cord, loss of consciousness ensues.1–3
Primary Factors Controlling Uptake, Distribution, and Elimination of Anesthetics
The basic task of anesthetic administration involves taking a drug supplied as a liquid, vaporizing it in an anesthesia machine, and delivering it to the patient’s brain and other tissues via the lungs. Therefore the main factors that influence the ability to anesthetize a patient are technical or machine specific, drug related, respiratory, circulatory, and tissue related. The primary factors that influence absorption of the inhalation anesthetics are ventilation, uptake into the blood, cardiac output, the solubility of the anesthetic drug in the blood, and alveolar-to-venous blood partial-pressure difference. Other factors such as the concentration and second gas effects also play a role (Figure 7-2).4
FIGURE 7-2 Transfer of an anesthetic gas from the machine through the lungs into the blood and tissues.
A few assumptions are usually made. The level of anesthesia is related to the alveolar concentrations of anesthetic agents, which can be readily and continuously measured or inferred. The concentration or partial pressure of anesthetic in the lungs is assumed to be the same as in the brain, because the drugs are highly lipid soluble and diffusible, and they quickly and easily reach equilibrium among the highly perfused body compartments. For this reason, the dose of an individual drug is expressed in terms of the minimum alveolar concentration (MAC) necessary to produce anesthesia (lack of movement) upon surgical stimulation.5–8 The faster the lung (and therefore brain) concentration rises, the faster anesthesia is achieved. Conversely, the faster the lung (brain) concentration falls after discontinuation of the drug, the more quickly the patient emerges.9
Machine-Related Factors
Concepts regarding the anesthesia machine and its function are described in detail in Chapter 15. Two factors that may affect uptake early in anesthetic administration are drug solubility in the rubber and plastic machine parts and total machine liter flow of the gases chosen.
The rubber and plastic components of the machine, as well as the ventilator and absorbent, can retain small quantities of anesthetic gases. Theoretically, this could slow administration to the patient at the start of anesthetic delivery. The effect on uptake is minimal in actual clinical practice and essentially ceases after approximately 15 minutes of administration.10 Nonetheless, sequestration of small amounts of gas in the apparatus has other implications, such as when anesthetizing patients with malignant hyperthermia. All gases except nitrous oxide are potent triggering agents for a hyperthermic episode. To avoid exposure resulting from residual trace amounts of gases, a thorough flush of the anesthesia machine with 100% oxygen at 10 L/min for at least 20 minutes, replacement of breathing circuits and the carbon dioxide canister, and draining, inactivation, or removal of vaporizers are advised when preparing for a patient who is susceptible to malignant hyperthermia.11 Proper preparation may vary with different machines and consultation with the machine manufacturer is advised.12,13
Low liter flows of oxygen and nitrous oxide carrier gas, although economical, deliver the anesthetic more slowly at the start of induction. Increasing liter flows for the first few minutes of the anesthetic minimizes this effect without unduly adding to cost.14
Drug-Related Factors
The blood/gas solubility coefficient of an anesthetic is an indicator of the speed of uptake and elimination.15,16 It reflects the proportion of the anesthetic that will be soluble in the blood, “bind” to blood components, and not readily enter the tissues (blood phase) versus the fraction of the drug that will leave the blood and quickly diffuse into tissues (gas phase). The more soluble the drug (high blood/gas coefficient), the slower the brain and spinal cord uptake and therefore the slower the anesthesia achieved by the patient. Soluble drugs stay in the blood in greater proportion than less soluble agents; therefore, less of the drug is released to the tissues during the early, rapid-uptake phase of induction. For example, isoflurane has a blood/gas solubility coefficient of 1.4 or, expressed as a ratio, 1.4:1. Therefore 1.4 times as much stays in the blood as a nonreleasable fraction for every 1 molecule that enters the tissues and produces anesthesia. Conversely, agents with low solubility properties (low blood/gas coefficient) leave the blood quickly and enter the tissues, producing a rapid anesthetic state. Desflurane, for example, has a low blood/gas coefficient of 0.42 or, expressed as a ratio, 0.42:1. Only 0.42 of a molecule stays in the blood for every 1 (greater than twice as much) that enters the brain. Anesthesia is achieved quickly. Blood/gas solubility coefficients for the inhalation anesthetic agents are listed in Table 7-1.17 The rate of rise of an anesthetic in the alveoli relative to the concentration administered is graphically depicted by plotting the fraction in the alveoli over the fraction inspired (Figure 7-3).
As noted previously, the lower the blood/gas solubility, the faster the rise in lung and brain concentrations. The rate of rise of low-solubility agents such as nitrous oxide and desflurane is greater than moderately soluble drugs such as isoflurane. Note in Figure 7-3 that nitrous oxide exhibits a slightly faster rate of rise compared with desflurane, despite a higher blood/gas coefficient. This variation in the usual trend is a result of the concentration effect—that is, nitrous oxide is given at much higher concentrations (50% to 70%) than desflurane (3% to 9%). Figure 7-4 depicts the effect of anesthetic blood solubility on uptake.
Ventilation Factors
As with all diffusible drugs, anesthetics move down a concentration gradient. Continuous inhalation administration of the agent into the lungs promotes subsequent diffusion into the blood and tissues as the anesthetic progresses. Anesthetic uptake slows throughout the surgical procedure as the tissue compartments become more saturated.18 The anesthetic is delivered, along with the necessary amount of oxygen or an oxygen mix appropriate for the patient’s condition. Supplemental nitrous oxide may also be used. Basically, the faster and more deeply a patient breathes or is ventilated, the faster the patient loses consciousness at the start of anesthesia and emerges at the end.16,19,20 This is often referred to as the ventilation effect.
Ventilation-perfusion deficits or poor lung function hinders inhalation drug administration.21,22 Rapid-acting (low blood/gas solubility) agents are affected by these deficits to a greater extent than are slower-acting (high blood/gas solubility) drugs.23 These decreases in speed can be partially compensated for by increasing the concentration of insoluble (fast) agents or increasing ventilation with soluble (slow) drugs.
Concentration or Dose
During the first minutes of gas administration, a higher concentration of the drug than necessary for maintenance, or a loading dose, is delivered to speed initial uptake. This is commonly referred to as overpressuring or the concentration effect.24 Overpressuring during initial administration is a common clinical practice and is more effective the more soluble the anesthetic. Overpressuring can speed the effect of slow agents but has less of an effect on relatively fast agents. This practice follows the kinetic standard of using a loading dose to speed onset. After the first few minutes, the dose is decreased to normal maintenance levels.25
As noted previously, the dose of an anesthetic is expressed in terms of MAC—the relative concentration when the anesthetic is combined with all the other gases in the lungs. Induction and maintenance doses are given as vaporizer settings of partial pressure in Table 7-2.
Second-Gas Effect
Simultaneous administration of a relatively slow agent such as isoflurane and a faster drug such as nitrous oxide (in high concentrations) can speed the onset of the slower agent. This is known as the second-gas effect.26 The uptake of the slower agent in the alveoli and to even a greater proportion in the arterial blood, is increased by administering it with a high concentration of the faster anesthetic nitrous oxide.27 The faster the inherent speed of the second gas on its own, however, the less prominent the augmentation when given with nitrous oxide. For example, sevoflurane, which has low blood solubility (blood/gas solubility coefficient 0.6) is rapidly taken up into tissues. Coadministration of sevoflurane with the slightly faster nitrous oxide produces a small but significant increase in uptake as compared with sevoflurane administration alone.25,28–30 The mechanism for the second-gas effect is not definitive, but it has traditionally been explained as the large volume uptake of nitrous oxide concentrating the other alveolar gases. Recent data suggests an added effect of ventilation perfusion scatter on the distribution of blood flow and gas uptake in the lung.27
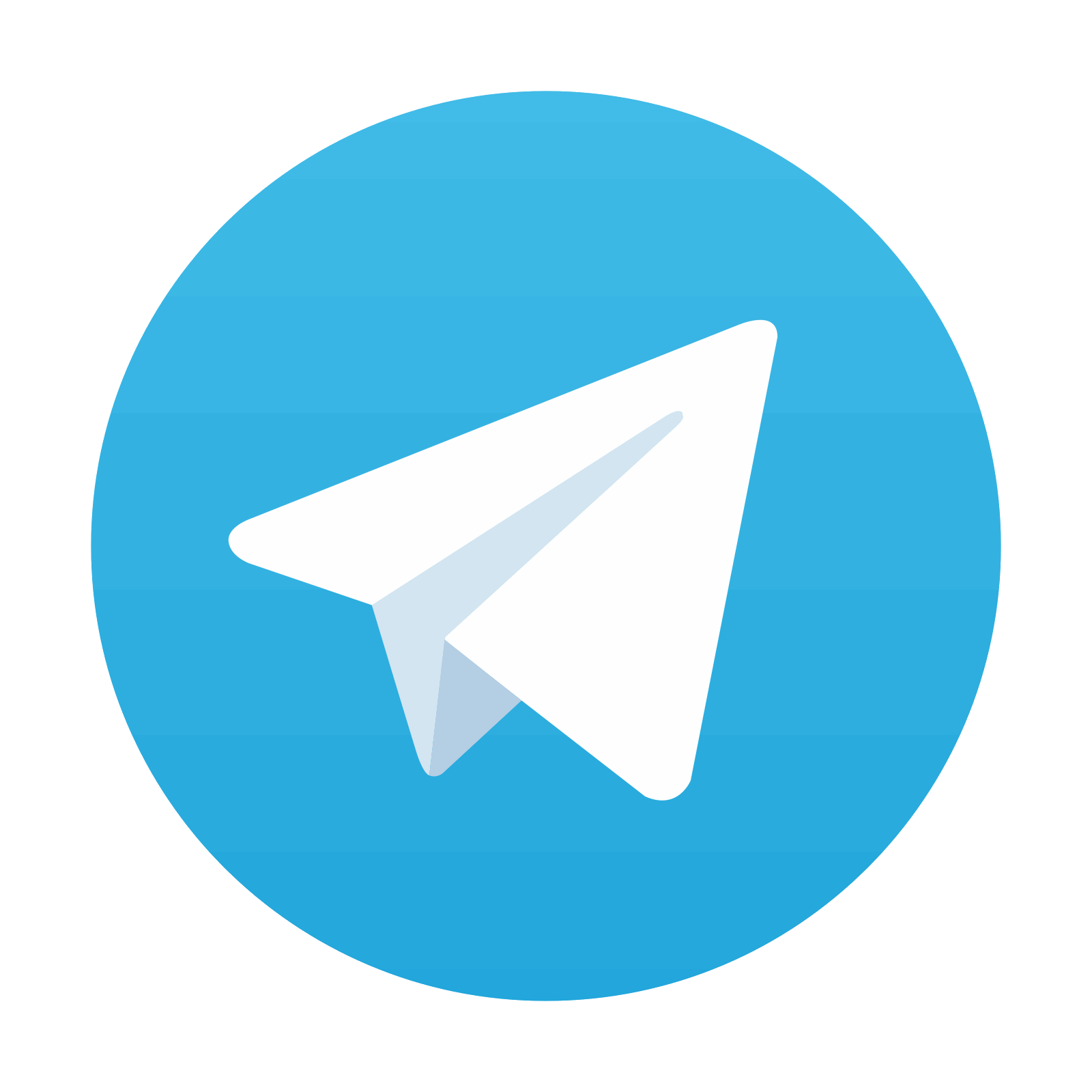
Stay updated, free articles. Join our Telegram channel

Full access? Get Clinical Tree
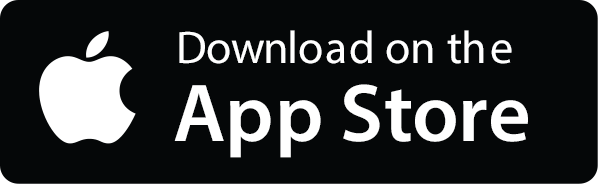
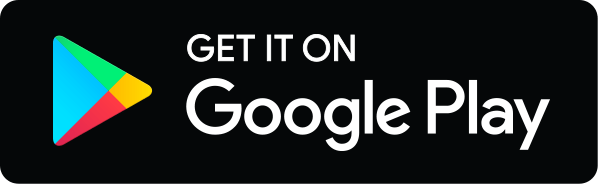