Abstract
Central to the practice of anesthesiology is the goal of safety and maximal efficacy for every individual patient. Genetics can influence drug metabolism, drug transport, and drug response target of action of drugs used in the anesthetic practice. This chapter provides a primer for those new to the concepts of pharmacogenomics and the historical role that anesthesia has played in the development of the field of pharmacogenomics/genetics. The majority of the chapter looks at pharmacologic classes of medications used in the anesthetic practice and the current role of pharmacogenetics in the pharmacokinetics and pharmacodynamics of commonly used anesthetic and analgesic drugs. Finally, the area of pharmacogenetics continues to evolve, and we cite examples where polymorphisms may influence the selection of perioperative medications to provide the safest and most efficacious care for each patient.
Keywords
Analgesics, Anesthesia, Interindividual variability, Metabolism, Pharmacodynamics, Pharmacogenetics, Pharmacogenomics, Pharmacokinetics, Polymorphism
Outline
Introduction 913
Basic Genetic Principles 914
Basic Pharmacologic Principles 914
Anesthesia Contribution to the History of Pharmacogenomics 915
Pharmacogenomics: Current Application to Clinical Anesthesia 916
Volatile Anesthetics 916
Intravenous Anesthetics 917
Analgesics 918
Mu (μ)-Opioid Receptor 918
Beta-Arrestin 2 Receptor 918
Catechol-O-Methyltransferase (COMT) 918
Melanocortin-1 Receptor (MC1R) 919
Drug Metabolism of Specific Opioids 919
Codeine 919
Hydrocodone 919
Oxycodone 919
Tramadol 919
Morphine 919
Methadone 920
Buprenorphine 920
Fentanyl 920
Muscle Relaxants 920
Local Anesthetics 920
Antiemetics for Postoperative Nausea and Vomiting 921
Conclusion 921
References 922
Introduction
Central to the practice of anesthesiology is the goal of safety and maximal efficacy for every individual patient. The challenge is that patients can have a range of responses to anesthetic drugs both beneficial and adverse. Potential causes for this variability include disease state, age, nutritional status, organ dysfunction, drug–drug interactions, and other comorbidities. While the basic pharmacologic principles of pharmacokinetics (PK) and pharmacodynamics (PD) remain paramount to safe anesthetic drug administration across the perioperative anesthetic practice (operative, critical care, pain), in the era of personalized medicine, these pharmacologic principles should be expanded to incorporate the influence of inherited differences. Knowledge of pharmacogenomics may be applied toward understanding and managing such interindividual variability to mitigate risk and tailor the anesthetic plan.
Basic Genetic Principles
Two terms frequently used interchangeably to describe the variability in sensitivity and responses to anesthetic drugs are pharmacogenetics and pharmacogenomics. Pharmacogenetics was the initial term used to describe the genetic influences of drug metabolism. As technology and knowledge of the human genome evolved, the term pharmacogenomics was born. Both terms are relevant, and for simplification, pharmacogenomics is what will be used throughout this discussion. Other commonly used terminology and definitions of concepts referred to in this chapter are summarized in Table 58.1 .
Common Term | Definition |
---|---|
Allele | One of at least two forms of a gene; one allele is inherited from each parent |
Genotype | A particular set of genes or DNA sequence inherited by an individual |
Haplotype | A combination of multiple genetic variants inherited by an individual |
Pharmacogenetics/pharmacogenomics | The study of how genetic variations affect drug response. Also using an individual’s genotype to predict drug efficacy or toxicity |
Phenotype | Any clinical or biochemical characteristic |
Polymorphism | Any natural genetic variation that occurs in at least 1% of the population |
Single nucleotide polymorphism | A change of a single nucleotide in the DNA that occurs in at least 1% of the population. This is the most common genetic variation |
Variant (or mutant) | Any change in the DNA sequence |
Most common genetic variations are a result of a change in a single nucleotide, referred to as a single nucleotide polymorphism (SNP). When a SNP is identified or studied the SNP is often named by the gene, the amino acid position at which the polymorphism occurs, and the wild-type and variant change. COMT V128M is an example of the nomenclature of the SNP. The first letter represents the most common allele, and the second represents the variant at that position. There are millions of SNPs known to date, yet it is unknown which polymorphisms identified are clinically important. This vast genetic variation presents a challenge to pharmacogenomics research—how to design a study that will best uncover the genetic explanation for the variability observed in clinical effect. Pharmacogenomic investigations in the field of anesthesia often focus on associations between clinical effect and important genetic polymorphisms from the pharmacokinetic or pharmacodynamic pathways of the anesthetic drugs commonly used in practice.
Basic Pharmacologic Principles
Two important principles in the area of pharmacology are PK—what the body does to the drug—and PD—what the drug does to the body. Genetic variation in PK (absorption, distribution, metabolism, and excretion of drugs), and PD (receptors, ion channels, and enzymes), may affect an individual’s response to the drugs used in anesthetic practice.
The majority of drugs used in anesthetic practice undergo phase I metabolism in the liver by the cytochrome P450 (CYP) enzymes, with a much smaller contribution from the phase II glutathione S-transferase and UDP glucuronosyltransferase (UGT) drug-metabolizing enzymes. There are a large number of CYP enzymes each named based on the family, subfamily, isoenzyme, and allele variant if known ( Table 58.2 ). The most clinically relevant ones are CYP1A2, CYP2B6, CYP2C19, CYP2D6, CYP2E1, and CYP3A4. Their specific roles in categories of anesthetic drugs are outlined in Table 58.3 and are further discussed in detail later in this chapter.
1A2 | 2B6 | 2C19 | 2D6 | 2E1 | 3A4 |
---|---|---|---|---|---|
Lidocaine | Methadone | Diazepam | Codeine | Volatile anesthetics | Buprenorphine |
Methadone | Propofol | Hydrocodone | Fentanyl | ||
Ondansetron | Methadone | ||||
Oxycodone | Midazolam | ||||
Tramadol |
Drug | Gene | Effect Associated With Variant |
---|---|---|
Halothane, other inhalation anesthetics | CYP2E1 | Hepatitis |
Halothane, succinylcholine | RYR1 | Malignant hyperthermia |
Succinylcholine | BChe | Prolonged paralysis |
Midazolam | CYP3A4 | Associated with prolonged sedation |
Codeine | CYP2D6 | Lack of efficacy (PM) toxicity (UM) |
Tramadol | CYP2D6 | Lack of efficacy (PM) toxicity (UM) |
Methadone | CYP2B6, CYP3A4 | Toxicity (PM) |
How effectively a patient metabolizes a drug determines the efficacy of that drug. Genetic variation in the metabolizing enzymes can lead to variable plasma levels and widely different metabolic phenotypes. This clinical variation in metabolism is used to classify how effectively a patient will metabolize a drug.
CYP2D6 is the best example of how this occurs and is most commonly studied. The genetic variation found in CYP2D6 can range from absent enzyme activity to enzyme activity >200-fold. Although a number of different genetic variants have been found, they are commonly grouped into four categories of metabolizers: poor metabolizer (PM), intermediate metabolizer, extensive or normal metabolizer, rapid and ultrarapid metabolizer (UM). The four categories correlate with the enzyme activity of the expressed alleles. The PM has two variant alleles that result in decrease or absent enzyme activity. The intermediate metabolizer has one normal allele with one deficient allele. The extensive or normal metabolizer has two wild-type or normal alleles. The rapid or UM have alleles that result in increased enzyme activity or an increase in the number of alleles present.
Identifying these categories of metabolizers has clinical significance. Individuals who are PMs are susceptible to overdose if the drug administered is active because of the accumulation of the drug and will have a poor response if the drug administered is a prodrug and requires metabolism to the active form. In contrast, those individuals carrying the UM phenotype will have a poor response to a drug that is administered in the active form because it will be rapidly cleared before clinical effect can be met, and will have an exaggerated response for a prodrug potentially leading to toxic plasma levels. This overview of the effect of genetic polymorphisms on drug metabolism, mainly PK, gives the foundation for the differences that will be explained later when a patient receives a standard dose of an anesthetic drug.
The majority of pharmacogenetics research in the field of anesthesia began with and still focuses on studies on the PK of drugs. While the receptors, transporters, and targets of anesthetic drugs that influence PD are being discovered, future studies on the influence of genetics on these targets will be revealing and in all likelihood alter the administration of anesthetic drugs.
Anesthesia Contribution to the History of Pharmacogenomics
The birth of pharmacogenomics reflects the gradual amalgamation of two historically great scientific disciplines: genetics and pharmacology. Its history dates back to 1865 when geneticist Gregor Mendel proposed the principles of heredity. His work subsequently laid the foundation for the physician Archibald Garrod to predict that there were genetically determined variations in enzyme activity involved in drug reactions.
The 1950s brought rapid recognition and adoption of the present concepts and approaches of pharmacogenomics. The association of prolonged muscle relaxation after succinylcholine with inherited deficiency of plasma cholinesterase exemplified inherited differences in drug effects. This pioneer work by the pharmacologist Werner Kalow placed anesthesia at the forefront of this evolution of linking aberrant drug responses with inherited differences and thus improving patient safety. In a now considered “classical” pharmacogenetics article, Motulsky wrote a review on cases of abnormal reactions caused by exaggeration or diminution of the usual effect of a given dose, including a case of prolonged succinylcholine effect to suggest that “hypersusceptibility or hyposusceptibility reaction may be caused by otherwise innocuous genetic traits or enzyme deficiencies.” The official term, pharmacogenetics, was coined shortly thereafter in 1959 by Friedrich Vogel in Heidelberg Germany.
Investigations into the anesthetic complication known as malignant hyperthermia (MH) also contributed to the novel science of pharmacogenetics. MH is now known to be a disorder of the skeletal muscle cell. Select individuals with variants in the gene encoding the ryanodine receptor (RYR1), which is a skeletal muscle sarcoplasmic reticulum calcium release channel, are susceptible to the life-threatening disease. Under normal conditions the ryanodine receptor through calcium release produces a signal that triggers muscle contraction. When individuals with RYR1 variants are exposed to halogenated volatile anesthetics and depolarizing muscle relaxants there is an exaggerated release of calcium from the sarcoplasmic reticulum resulting in uncontrolled activation of other biochemical pathways. Clinically this cellular overactivation leads to hyperthermia, elevated end-tidal carbon dioxide levels, hyperkalemia, acidosis, muscle rigidity, rhabdomyolysis, organ system failure, and possibly death if not identified and treated promptly. Genetic testing for RYR1 variants is specific but not sensitive for MH. A positive genetic test for a RYR1 variant confers susceptibility to MH, but those with a negative test result might still be susceptible to MH but not through the RYR1 variants. Other variants that have been identified that may contribute to MH susceptibility include the voltage-dependent L-type calcium channel (CACNA1S).
Pharmacogenomics: Current Application to Clinical Anesthesia
Included in this section is a summary of genetic associations with clinical outcomes for the most widely used perioperative drugs classifications: volatile and intravenous (IV) anesthetics, analgesics, muscle relaxants, local anesthetics, and a few adjuncts of clinical relevance with the best evidence of support. We have included areas with superficial evidence to demonstrate interest and the future of pharmacogenomics application in the field of anesthesia, perioperative outcomes, and pain. Each section will emphasize the inherited differences in proteins involved in the PK and PD of the different anesthetic classes when known.
Volatile Anesthetics
Volatile anesthetics have been the mainstay of anesthetic practice ever since the first public demonstration of ether by William Morton in October 1846. This drug class has undergone rapid evolution to the newer anesthetics. However, this class of drugs has not been exempt to adverse reactions, with the most notable being MH syndrome. Pharmacogenetic research has contributed to our present understanding of this severe life-threatening reaction, as described in detail earlier. Beyond that, there has been limited application of pharmacogenetics of volatile anesthetics. One explanation for this deficiency is the lack of mechanistic studies for volatile anesthetics. The available data to date are primarily from case-reported variation in inhalation effect or rarely observed variability in adverse outcome.
Halogenated Inhalational Anesthetics
Postoperative immune hepatitis correlates with the extent to which the halogenated anesthetics, halothane, enflurane, isoflurane, and desflurane, are metabolized. The metabolism of these halogenated anesthetics by the enzymes CYP2E1 and CYP2A6 lead to trifluoroacetyl chloride synthesis and tissue acetylation, which can further produce damage, which is more pronounced especially in the liver where the anesthetic metabolism occurs. With the exception of halothane, fortunately metabolism is only a small percentage of the commonly used anesthetics, and as of yet, there is limited literature to support any genetic influences on this anesthetic adverse outcome.
Another observed adverse outcome is nephrotoxicity related to elevated plasma fluoride concentrations. This is seen with the anesthetic sevoflurane. The PK of sevoflurane involves 95% secretion of the unchanged form and 5% biotransformation to the inorganic fluoride metabolite. The enzyme CYP2E1 is responsible for this biotransformation. Theoretically, elevated inorganic fluoride concentrations could contribute to nephrotoxicity. Studies involving the known polymorphic variants of CYP2E1 and plasma concentrations of inorganic fluoride metabolite after sevoflurane anesthesia are lacking, likely related to the large number of variants found in this gene ; thus no appreciable pharmacogenetics association can be drawn at this time, but could be warranted in the future.
Nitrous Oxide
Acute demyelination has been associated with prolonged exposure to nitrous oxide. The mechanism responsible for this relates to deficiencies in folate metabolism. Vitamin B12 is a cofactor for the enzyme methionine synthase in the folate cycle. This folate cycle is responsible for downstream cellular functions such as DNA synthesis. Nitrous oxide inactivates vitamin B12, which can then impact folate metabolism and subsequent cellular functions, which manifest clinically as neurologic and hematologic (agranulocytosis, megaloblastic anemia) disorders. The enzyme 5,10-methylenetetrahydrofolate reductase (MTHFR) of the folate pathway, is not affected by nitrous oxide; however, this enzyme is known to have common genetic variants resulting in decreased function. In the literature there are case reports of nitrous oxide administration and concomitant MTHFR enzyme deficiency resulting in neurologic deterioration and even death of an infant after anesthesia. Later studies showed the presence of common genetic variants in the MTHFR gene in those cases. These reports highlight how underlying genetic influences may alter anesthetic outcomes.
Until further investigated, the contribution of these pharmacogenetic observations only provides awareness of the potential clinical relevance of the genetic influences on inhalation anesthesia. Having a better understanding of the mechanisms of actions of these drugs will continue to transform volatile anesthetics and the application of pharmacogenetics.
Intravenous Anesthetics
IV anesthetics have a primary as well as secondary role to the inhalation anesthetics in induction and maintenance of anesthesia. This section aims to review the currently known PK and PD of the commonly used IV anesthetics and assess the pharmacogenetic implications.
Propofol
Propofol is a widely used IV anesthetic from sedation to induction to its use in total IV anesthetic. This drug is metabolized primarily by UGT1 with other contributions by CYP2B6 and SULT1A. Adverse effects of propofol administration include delayed emergence or propofol infusion syndrome described as metabolic acidosis and cardiovascular collapse after prolonged infusion. Studies have been initiated to identify any association with the enzymes involved in propofol metabolism and adverse effects and interindividual variability. Given that UGT1 is the primary pathway for metabolism of propofol, association studies with UGT1 polymorphisms and clinical response to propofol have shown decreased metabolism with three common variants (D256N, Y482D, Y486D). For the other pharmacokinetic pathways, the CYP2B6 G516T variant was reported to significantly affect propofol plasma concentrations. Other CYP2B6 polymorphisms also alter the kinetics of propofol up to 19-fold. These positive pharmacogenetic associations between variation in the pharmacokinetic pathway and clinical outcome have yet to be further replicated. One possible explanation for this lack of reproducibility is that most studies have focused on single variant association, while the interindividual differences in propofol metabolism may be best explained through the contribution polymorphisms in multiple pathways. This is a limitation of many pharmacogenomics association studies.
Equally important to the PK of propofol is the potential for variation at the site of action for propofol. It is known that propofol, along with many other anesthetics, potentiates the actions of the γ-aminobutyric acid type A (GABAA) receptor function. Therefore, interest in genetic variation that could alter GABAA receptor and thus contribute to the variable response to propofol as well as other anesthetics has garnered research attention. In vitro studies of the various GABAA variants and propofol have shown the Y444W variant to attenuate the effect of propofol. However, at present, no in vivo associations with the GABAA receptor polymorphisms and clinical effects of propofol have been identified.
Benzodiazepine
This class of drugs is used for sedation and anxiolysis. Prolonged sedation or unconsciousness after administration has the potential for severe adverse outcome as well as delays in discharge. Genetic differences have been investigated for two drugs in this category: diazepam and midazolam. Both are metabolized by CYPP enzymes with CYP3A4 primarily responsible for midazolam metabolism and CYP2C19 for diazepam metabolism. Diazepam appears to have the most clinically significant effects of genetic differences in CYP2C19 G681A as a result of decreased enzyme activity with the variant and therefore increased plasma half-lives of the drug. Midazolam clearance and CYP3A4 polymorphisms are tightly associated ; however, this has not translated to any clinical significance. The explanation for this is thought to be a result of redundant pathways for excretion and metabolism of midazolam.
Ketamine
Unlike the previously discussed anesthetics, ketamine’s primary source of action is on the N -methyl- d -aspartate (NMDA) receptor. Variations in plasma concentrations of ketamine metabolites have been observed suggesting that pharmacogenomics may play a role in clinical response. Despite polymorphisms being identified in the NMDA receptor, this is a virtually uncharted territory for pharmacogenomics and anesthesia.
Dexmedetomidine
Although not used as a primary anesthetic, dexmedetomidine is often used in conjunction with other IV anesthetics for total intravenous anesthetic, emergence delirium, or analgesia. Dexmedetomidine acts as a selective α2A receptor agonist. These receptors are located both centrally and in the periphery. While central activation gives rise to the analgesic and anesthetic effects, peripheral activation is what is responsible for adverse side effects like bradycardia and hypotension. A few studies of the effect of variants in the α2A receptor and the cardiovascular response have been carried out. In a study by Kurnik et al., they discovered that specific haplotypes in the α2A receptor gene, ADRA2A, had direct influence on individual heart rate or blood pressure response to dexmedetomidine.
Analgesics
Opioids have long been used in the field of anesthesia for the treatment of moderate or severe acute and chronic pain. There is wide interindividual variation in opioid analgesic dosage requirements, efficacy, and side effects. Some of this variation can be explained by genetic variation affecting both the pharmacodynamic and pharmacokinetic parameters of opioids. Undoubtedly, this area of anesthesia is the best developed from a pharmacogenomics perspective. This section aims to review how pharmacogenomics can transform analgesic therapeutic decisions, most fundamentally, how the anesthesiologist can decide which opioid to select and the appropriate dosing.
Mu (μ)-Opioid Receptor
There are three known opioid receptors—mu (μ), kappa (κ), and delta (δ), yet of these three it is binding to the μ-opioid receptor that primarily mediates the opioid response of analgesia, sedation, and respiratory depression. There are known interindividual differences in responses to painful stimuli and response to opioid drugs. Pharmacogenomics research has contributed to a better albeit incomplete understanding of the mechanisms responsible for this variation. The μ receptor is encoded by the OPRM1 gene. Studies have shown that genetic polymorphisms in the opioid receptor leads to biochemical changes in receptor density and function, and this may help to explain the variation in clinical effects observed. Although numerous genetic variants have been identified in the OPRM1 gene, the most commonly studied is the A118G SNP. This variant allele has a frequency of 10–48%. Multiple studies in simulated environments as well as surgical populations suggest that carriers of the variant allele require more opioid to achieve comparable efficacy of pain relief as compared to wild type.
Although there are several opioids available, most pharmacogenomics studies have centered on morphine as the prototypical example. In addition to the pharmacogenomic contribution to the positive effects of opioid analgesia, some studies have utilized pharmacogenomics to address the common adverse events of opioid use such as nausea and vomiting, drowsiness, and altered sensorium. The limited studies completed to date show the 118G variant to be associated with decreased sedation and less nausea and vomiting postoperatively with morphine use. This genetic association does not appear to translate to other opioids, however, since fentanyl-induced postoperative nausea and vomiting did not have any association with the genotype.
Beta-Arrestin 2 Receptor
Opioid receptors do not work in isolation. Intracellular signaling pathways both carry the activities of the receptors and modulate receptor expression. Beta-arrestin 2 is one of many intracellular proteins that regulate μ-opioid receptor expression as well as regulate the agonist-bound μ-opioid receptor signaling cascade. Genetic variation in the beta-arrestin 2 gene (ARRB2) has been shown to influence the clinical response to opioids. A study by Ross et al. showed differential tolerance to morphine in accordance with beta-arrestin 2 polymorphisms and that opioid switching mitigated this effect. A study by Oneda et al. showed that a beta arrestin 2 haplotype was associated with a decrease in efficacy for methadone maintenance therapy.
Catechol-O-Methyltransferase (COMT)
Indirect pathways can also impact opioid effectiveness. Pain perception and even efficacy of opioid analgesia can be influenced by catecholamines. Catecholamines are inactivated by the enzyme COMT. A known common polymorphism G1947A leads to reduction in COMT enzyme activity and subsequent lower catecholamine levels. The largest reduction in enzyme activity is seen with the C158M polymorphism. Those with the G1947A variant allele have been shown to have decreased tolerance to experimental pain. Conflicting studies in cancer pain show the wild-type allele to have increased morphine requirements.
Melanocortin-1 Receptor (MC1R)
MC1R has a number of known functions including adrenocortical steroidogenesis, inflammatory response, and thermoregulation. Laboratory studies in mice and humans indicate a link between MC1R variants, pain tolerance, and enhanced response to opioid analgesia. This could be because of the role of MC1R in κ-opioid receptor sensitivity. Of note, genetic variants in MC1R have also been associated with red hair phenotype. It has been well documented that patients with red hair have been associated with reduced sensitivity to pain and augmented analgesic response to opioids. At present, there is no genetic test for MC1R performed prior to anesthesia or opioid regimen, but until then, red hair might serve as an indirect marker when considering anesthetics and analgesic plans.
Drug Metabolism of Specific Opioids
Genetics can influence the metabolism and therefore the effectiveness of opioids. The various opioids commonly in use today undergo metabolism via different enzymes in phase 1 or phase 2 metabolism. The following discussion will highlight the major pharmacogenomic influence on metabolism of currently used opioids, beginning with codeine, which has been the prototype example for the genetics can influence metabolism, drug toxicity, and efficacy.
Codeine
Codeine is considered a prodrug. Its effectiveness as an analgesic depends on its metabolism to the active compound morphine. CYP2D6 is the enzyme responsible for approximately 10% of this metabolism. Individuals with the CYP2D6 PM genotype do not respond well to codeine. The inadequate pain relief is from the decreased metabolism to the active form. These “nonresponders” would achieve a better clinical response when given an alternative opioid that does not require the CYP2D6 pathway for activation. In contrast, individuals with the CYP2D6 UM genotype have increased transformation of codeine to morphine and are at risk for side effects due to the accumulation of higher than normal, if not fatal, levels of morphine in the setting of normal doses of codeine. There have been cases reported of neonatal fatalities from opioid toxicity in children breastfed by mothers with the CYP2D6 UM genotype who were taking codeine as well as deaths in children with the ultra-rapid genotype undergoing adenotonsillectomies receiving codeine for outpatient pain relief. This genetic information has resulted in federal warnings in the United States against prescribing codeine for nursing mothers as well as children undergoing adenotonsillectomies.
Hydrocodone
Hydrocodone when administered is a weak opioid with only a limited affinity for the μ-opioid receptor. It undergoes metabolism to hydromorphone, which has a higher affinity for the μ-receptor and greater analgesic efficacy. This metabolism is by the enzyme CYP2D6. Although studies have shown differences in levels of hydrocodone and hydromorphone in individuals with the known UM or PM genotypes, currently the known genetic variation in CYP2D6 has not influenced the efficacy or toxicity to the extent that it warrants an understanding of genotype prior to administration.
Oxycodone
Oxycodone is an active compound that is primarily metabolized to an inactive metabolite noroxycodone through the CYP3A4/5 enzymes. However, approximately 10% of the metabolism is through the CYP2D6 enzyme, which produces the active metabolite oxymorphone. The clinical significance of genetic variation in CYP2D6 in the analgesic effects of oxycodone is not clear. Thus there are no current recommendations for dosage adjustments.
Tramadol
Tramadol is metabolized to the M1 metabolite by CYP2D6. This metabolite is more potent than tramadol. Studies in the postoperative setting indicate that tramadol use was increased in individuals with the PM genotype as compared to the UM or intermediate metabolizer genotype. It is recommended both codeine and tramadol be avoided in PM or UM.
Morphine
Morphine is metabolized to the metabolites morphine-3-glucuronide and morphine-6-glucuronide by the hepatic enzyme UGT2B7. There is known genetic variation in UGT2B7, and this variation can influence metabolite production, yet no clinical studies to date have shown an association between those genotypes and clinical response to morphine.
Methadone
Methadone is used in opioid addiction therapy as well as for treating acute and chronic pain. Unfortunately, the effectiveness of methadone is hindered by clinically observed variability in the PK and PD. It is well established that methadone is metabolized by CYP3A4 and CYP2B6. Investigations have demonstrated an association with CYP2B6 genotypes and clinical response. Individuals with a CYP2B6 variant genotype have higher plasma concentrations of methadone and reduced clearance. Associations have not been identified with any of the CYP3A4 variants. This could be a result of the presently known CYP3A4 genotypes having little effect on enzyme function.
Buprenorphine
Buprenorphine is a semisynthetic opioid structurally similar to morphine, yet reported to be at least 25 times more potent. The strong receptor affinity prevents binding of other opioids, such as fentanyl, interfering with the effect of these other opioids and thus preventing their analgesic or antagonistic benefits. Several buprenorphine formulations are being used to treat chronic pain or substance abuse. The metabolism of buprenorphine is primarily through CYP3A4, with minor contribution from UGTB7. Limited evidence suggests that those with a CYP3A4 genotype have a decreased response to buprenorphine for limb pain, while those with the UGT2B7 polymorphism have a decreased response to pain after thoracic surgery. These studies are surgery specific, yet introduce the potential influence that genetic variation may have on the clinical efficacy of buprenorphine.
Fentanyl
Fentanyl is widely used in anesthesia, analgesia, and sedation because of its rapid onset. However, like many other opioids, there can be up to a fivefold interindividual variation in dose to achieve a similar clinically effect. Fentanyl is metabolized to an inactive metabolite by CYP3A4. In a study by Yuan et al., it was found that the metabolism of fentanyl was lower in patients homozygous for a CYP3A4 variant haplotype as compared to those with the wild-type allele. This work was performed using explanted liver microsomes and therefore would need to be replicated in human studies to determine if plasma levels are consistent with the decreased metabolism.
While many studies demonstrate an association of genetic variants in the PK or PD pathways of opioids, for the majority, there is limited guidance from the current literature regarding pharmacogenomics and perioperative management for patients on these agents.
Muscle Relaxants
Endotracheal intubations as well as surgical conditions are two primary indications for using neuromuscular blocking drugs. They can be categorized into short-, intermediate-, and long-acting drugs. The intermediate- and long-acting neuromuscular blocking agents have no identified variation in the duration of action related to genetics.
Among the most commonly used short-acting drugs, mivacurium and succinylcholine metabolism depend on rapid hydrolysis by the enzyme butyrylcholinesterase. Variation in metabolism leading to prolonged duration of action has significant clinical implication. Known genetic variants of plasma butyrylcholinesterase correlate with clinical differences in the duration of muscle paralysis. For example, the expression of the D70G polymorphism has decreased enzyme activity of the plasma butyrylcholinesterase. Homozygous expression results in the most profound clinical effect with muscle paralysis lasting 60 times longer than normal after drug-induced muscular paralysis with these short-acting neuromuscular drugs. The prevalence of this genetic variant can be up to 25% in the Caucasian population. Knowledge of the genetic profile for butyrylcholinesterase is typically identified postoperatively once prolonged paralysis is observed. Administering drugs to a homozygote or heterozygote could increase recovery time as well as cost after surgery due to prolonged monitoring in a higher level of care. This is an example of where pharmacogenomics has been useful to provide an explanation for abnormal response to drug; however, the best way to utilize this knowledge would be to identify preoperatively which patients are at risk. As genomic testing evolves and practitioners are able to procure genetic profiles, this would be the future of pharmacogenomics practice in anesthesia.
Local Anesthetics
Local anesthetics have a role in regional and topical anesthesia. Observations of variability in duration of local anesthetics have been reported and studied. Trescot et al. performed a series of skin tests with different local anesthetics to establish the variability in response to pain among the different agents. Over 1000 patients were enrolled, with 20% having difficulty achieving adequate analgesia though local anesthetics. There was variability in the degree of analgesia among the different agents with 7.5% having numbness only to mepivacaine and 3.8% only to lidocaine. Although no genetic testing was performed on these individuals, this presents a clinical phenotype that would be amenable to further genetic testing.
Possible explanations for these differences could be in the PK or PD of local anesthetics. Metabolism of local anesthetics varies depending on the amide or ester classification, with the esters undergoing more rapid metabolism by esterases. The amides can undergo oxidation by the CYP3A4 or CYP1A2 isoforms of metabolizing enzymes. Despite the known genetic variation in these metabolizing enzymes, there has yet to be genotype–phenotype studies of the association of those variants with local anesthetic onset or duration of action. This is probably a reflection of the fact that local anesthetic administration and onset do not depend on metabolism.
Although distinctive differences in the PK of the local anesthetics can be made, they universally share the same mechanism of action by blocking the voltage-gated sodium channel in the axon thus abolishing action potentials being propagated to the central nervous system. There have been examples of resistance to lidocaine with a genetic variant N395K in the Nav1.7 sodium channel. As more is uncovered regarding the structure and function of the sodium channel, this will provide additional areas for genotype–phenotype studies to be performed.
Antiemetics for Postoperative Nausea and Vomiting
Postoperative nausea and vomiting (PONV) is an unpleasant and debilitating effect of general anesthetics. Risk factors for experiencing PONV include female sex, postoperative narcotic use, and a history of motion sickness or PONV. 5-Hydroxytryptamine type 3 receptor (5HT3) antagonist, ondansetron, granisetron, and dolasetron are widely used for PONV prophylaxis treatment as well as rescue agents. Differences in clinical efficacy are thought to be attributed to variability in affinity of the drug for the receptor. Granisetron is metabolized by CYP3A4, while the remaining 5HT3 antagonists are metabolized through the highly polymorphic CYP2D6. This was clinically observed in a study by Candiotti in which the incidence of PONV correlated with the CYP2D6 metabolizer status, with those in the UM category having a significant increase in the incidence of PONV as compared to the extensive (normal) metabolizers (45.5% vs 14.7%). The decreased effectiveness of the 5HT3 antagonist in the UMs would suggest two future directions: (1) the benefit of known CYP2D6 status prior to drug administration and (2) consideration of another agent for treatment that is not dependent on the CYP2D6 metabolism, such as granisetron. Obviously major practice changes would require additional large-scale studies, but this is the first step in a pharmacogenomics approach to tailored drug therapy.
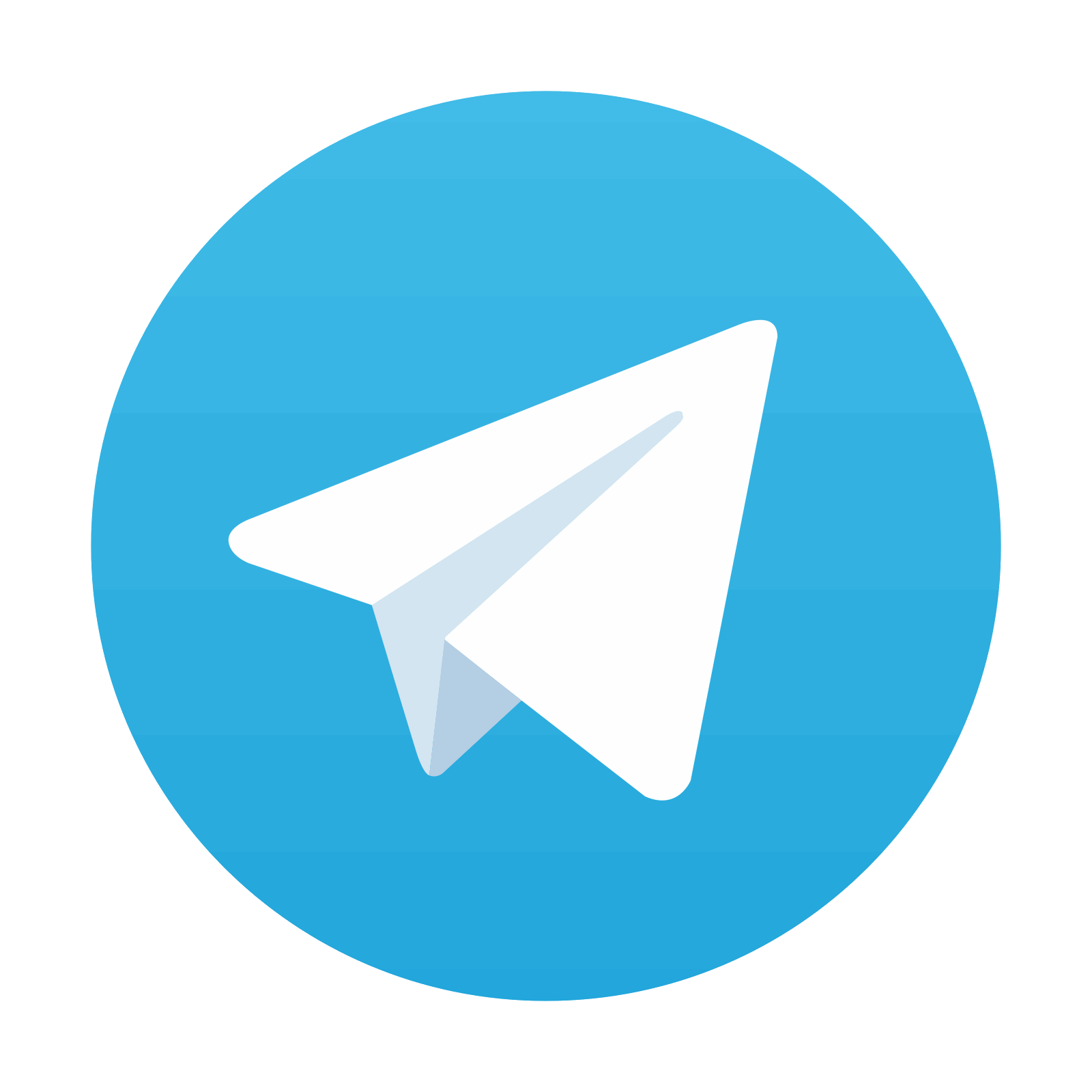
Stay updated, free articles. Join our Telegram channel

Full access? Get Clinical Tree
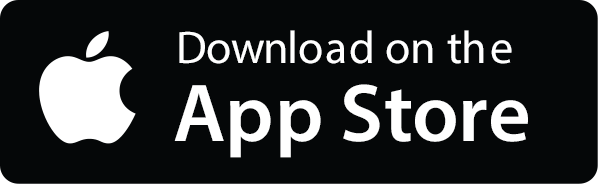
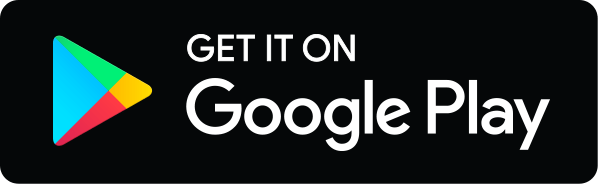