Rhonda J. Moore (ed.)Handbook of Pain and Palliative CareBiobehavioral Approaches for the Life Course10.1007/978-1-4419-1651-8_25
© Springer Science+Business Media, LLC 2013
25. Phantom Limb Pain
(1)
Department of Cognitive and Clinical Neuroscience, Central Institute of Mental Health, University of Heidelberg, Mannheim, Germany
Abstract
Chronic phantom limb pain is a common occurrence after the amputation of an extremity, often causing a considerable level of suffering for years or decades after an accidental injury or an operation. Due to its intractable nature and multilayered working mechanisms, it has proven to be a challenge for both practitioners and researchers. Studies have linked this type of chronic pain to alterations in the peripheral nervous system, the spinal cord, the brainstem, the thalamus, and the cortex. Mechanisms that contribute to the elicitation and perpetuation of chronic phantom limb pain may be the formation of neuroma, central sensitization, cortical reorganization, and pain memory. The tendency of developing phantom limb pain after limb amputation seems to be connected to perceptual illusions, such as integrating the mirror image of a limb or a rubber hand into one’s own body representation. These findings have led to novel forms of treatment, such as mirror therapy, imagery training, or the application of virtual reality. Other promising treatments include pharmacological approaches before and after amputation, sensory discrimination, central stimulation, the use of myoelectric prostheses, and the combination of medication and behavioral approaches.
The phenomenon of phantom limb pain, the feeling of pain in a body part that has been lost in an accidental or clinical amputation, has been known to medicine for a long time. In 1552, Ambroise Paré described this seemingly strange occurrence and postulated that the causal factor of phantom limb pain might not be some type of mental illness, like it was thought before, but a pain memory or peripheral factors (Keil 1990). The phenomenon received its modern name from Mitchell in 1872.
Phantom limb pain has to be distinguished from nonpainful sensations in the lost limb (phantom sensations), including the general perception that the limb is still there (phantom awareness), and residual limb (or stump) pain or nonpainful residual limb phenomena (Hunter et al. 2008). Whereas the majority of people who have had an amputation experience the feeling that the limb is still there (Hunter et al. 2003), phantom limb pain occurs in about 50–80% of amputees (Ephraim et al. 2005). While most amputations, both medical and accidental, affect arms or legs, painful and nonpainful phantom sensations have also been reported after the loss of other body parts like the breast (Rothemund et al. 2004) or a tooth (Marbach and Raphael 2000) and can also occur after spinal cord injury (Melzack and Loeser 1978) or brachial plexus avulsion (Finnerup et al. 2010).
The development, intensity and quality of phantom limb pain, compared between individuals, tends to be very heterogeneous. Some people report occasional short bursts of pain, divided by long stretches of painlessness, while others constantly experience the pain. The intensity ranges from a slightly annoying perception of pin pricks to a severe and excruciating experience of pain. Usually felt as more intense in the distal part of the phantom, it can also be experienced in a variety of different ways, including throbbing, burning, and cramping. In keeping with the general heterogeneity of the syndrome, the onset of phantom limb pain can occur immediately after the amputation or years later. In many cases, phantom limb pain can be elicited or exacerbated by a variety of environmental factors, like a change in the weather, psychological factors, or emotional distress. Similar variations are visible in nonpainful phantom phenomena: Patients can experience a numbness or a change in temperature of the phantom limb, the feeling that the missing limb is staying in a specific position (in some cases in a position that would be physically impossible for an actual limb) as well as voluntary and involuntary movements of the phantom. Onset and intensity of these nonpainful sensations are also heterogeneous. While most of this variability remains unexplained, factors such as age and gender do not seem to have an influence on the development of phantom limb pain (Hanley et al. 2007). Both phantom limb pain and nonpainful phantom phenomena are only very rarely reported in patients with congenital limb deficiency (Wilkins et al. 1998) and it is unclear how those rare cases are to be interpreted in terms of a model of phantom limb pain (Price 2006).
In the light of the described variability, it is crucial to identify causal factors for phantom limb pain in order to prevent it from developing or to determine alleviating factors as a foundation for possible therapies. While there is a correlation between preoperative pain and phantom limb pain, recent improvements in pre- and perioperative pain treatments still have not led to a decrease in the number of new phantom limb pain sufferers (Nikolajsen and Jensen 2005). Also, if preoperative pain is an important factor for whether or not phantom limb pain will develop, we would expect a higher rate of phantom limb pain among amputees in the next few years or decades, as (at least in the Western world) an ever-increasing proportion of amputations are performed due to the effects of vascular diseases. In these cases, there have usually been painful sensations in the affected limb for months or even years prior to the amputation.
The removal of a limb not only changes the peripheral nervous system by cutting nerve fibers, but it also causes changes in the central nervous system, because the brain has to adapt to a new and different situation. In order to determine whether peripheral or central factors are responsible for eliciting phantom limb pain, Birbaumer et al. (1997) anesthetized the brachial plexus of amputees suffering from phantom limb pain, thereby ensuring that for a certain amount of time no peripheral input could be processed. They found that this method eliminated pain in 50% of the participants, raising questions about the influence of central and peripheral factors in the experience of pain. The knowledge of both types of causal factors is crucial if we are to prevent as well as provide effective pain relief for patients with phantom limb pain.
Peripheral Factors
Since neurons are flexible entities, which can grow and change their shapes to some degree, the damage caused to nerve fibers during an amputation can have differing consequences in individual cases. Sometimes, the terminal swelling that is formed and the axonal sprouting that occurs after a nerve cell is cut can lead to the formation of a neuroma. These are tangled knots of nerve tissue that develop because the axons are unable to reconnect properly. The activity of such malformed neurons can be spontaneous and unpredictable (Fried et al. 1991). Ectopic signals produced by these neuromas might also be responsible for some forms of pain in amputees.
Changes in the electrical properties of cell membranes can increase the excitability of damaged nerve cells. The mechanisms responsible for these changes involve the upregulation or novel expression, as well as the trafficking, of voltage-sensitive sodium channels and decreased potassium channel expression (Devor 2006). Another factor are altered transduction molecules for the sensitivities to heat, cold, and mechanical stimulation of the neuroma (Gorodetskaya et al. 2003). These alterations in the firing behavior of injured neurons might lead to the often reported exacerbating effects of changing temperatures on phantom limb pain, as well as the elicitation of phantom limb pain by nonpainful tactile stimulation of the stump (Nikolajsen and Jensen 2005).
However, the formation of neuromas is not instantaneous. Thus, the described effects cannot account for the frequent reports of phantom limb pain immediately after the amputation. Thus, researchers have looked for other sources of ectopic activation and found such activity, which can even summate with the ectopic discharges described earlier, in the dorsal root ganglion (DRG). This is a module on a dorsal root, containing the cell bodies of afferent neurons in the spine. Interactions between neighboring neurons can amplify the overall ectopic signal and thus exacerbate the pain. Moreover, both spontaneous and triggered sympathetic activation can elicit or intensify ectopic discharges (Chen et al. 1996), which might be one reason why the experience of phantom limb pain is generally stronger during times of emotional distress. This sympathetic-sensory coupling occurs both at the level of the neuroma and at the level of the DRG. Recently, it has been shown that there might be a genetically determined disposition to develop ectopic neuroma and DRG discharge (Devor 2005a, b). The genetic influence on the development of phantom limb pain, though understudied at this point in time, could also explain some of the observed differences in the experience of pain between individuals with very similar medical histories and kinds of amputation.
Central Factors: The Spinal Cord
There have been reports of usually pain-free amputees who have experienced phantom limb pain during spinal anesthesia (Schmidt et al. 2005). This phenomenon, paired with results from animal studies on neuropathic pain, suggests that changes in the spinal cord might be an important factor in the development of phantom limb pain. Altered activity in peripheral neurons can lead to enduring changes in the behavior of nerve cells at the level of the spinal cord: Increased nociceptive input related to inflammatory pain causes long-term changes in the synaptic responsiveness of neurons in the dorsal horn of the spinal cord, in a process known as central sensitization (Woolf and Salter 2005). It is feasible that a similar process of hyperexcitability is caused by the nerve damage also occurs during amputation. Increased firing of the dorsal horn neurons, structural changes at the central endings of the primary sensory neurons, and reduced inhibitory processes can be spinal effects of injured nerves (Woolf 2004). Interneurons containing the inhibitory substance γ-aminobutyric acid (GABA) or inhibitory glycinergic interneurons in the spinal cord may actually be destroyed by rapid ectopic discharge or other effects of axotomy (Woolf 2004), or they might change from their usually inhibitory to an excitatory effect, being influenced by BDNF (brain-derived neurotrophic factor), which is released by microglia cells (Coull et al. 2005).
The described mechanisms might all contribute to the hyperexcitability of the spinal cord circuitry after major nerve damage. Joining them is the downregulation of opioid receptors, both on primary afferent endings and intrinsic spinal neurons (Wang et al. 2005), which is expected to reduce activity of the inhibitory neurotransmitters GABA and glycine, thus increasing the disinhibition in the spinal cord. The upregulation of cholecystokinin, an endogenous inhibitor of the opiate receptor, which occurs in injured neural tissue (Wiesenfeld-Hallin et al. 2002), can also add to this effect.
Long-term changes in firing behavior also occur in ascending projection neurons from the spine to supraspinal centers. For example, the response of N-methyl-d-aspartate (NMDA) receptors to the primary afferent neurotransmitter glutamate can be facilitated after nerve damage (Torsney and MacDermott 2006). Interestingly, in some instances the behavioral change of neurons after tissue damage is not confined to an altered sensitivity: After injury, the large and myelinated Aβ-fibers, which usually carry nonnociceptive information, may express substance P. This neuropeptide is attributed to the sensation of pain and is usually expressed only by Aδ-afferents and C-afferents, most of which are nociceptors. These nociceptors conduct quick, sharp pain and secondary, delayed pain, respectively. This means that the damage caused by an amputation can cause low-threshold afferents to become fractionally connected to ascending spinal projection neurons which carry nociceptive information to supraspinal centers (Ueda 2006), thus being effectively transformed into nociceptors contributing to phantom limb pain.
The physical damage caused to neurons can be exacerbated by the degeneration of central projection axons. After dorsal root injury, or their separation from the spinal cord, deafferentation occurs on a large scale. If a region of the spine is vacated because of injured afferents, the neighboring regions can invade this area, which results in a spreading of hyperexcitability in the spinal cord. In animals, it has been shown that this spinal reorganization can lead to an expansion of the receptive fields on the parts of the skin next to the deafferented area (Devor and Wall 1978). As we shall see, similar effects of reorganization play an even more important role when it comes to the brainstem and cortex.
Central Factors: Brainstem, Thalamus, and Cortex
The peripheral and spinal causes that have been discussed cannot be the only determining factors associated with the development of phantom limb pain. For example, the fact that spinal anesthesia does not always alleviate phantom limb pain (Baron and Maier 1995) suggests that supraspinal changes can also influence this phenomenon. It is, of course, possible that the spinal changes discussed earlier may also have a strong influence on the supraspinal alterations, although this “bottom-up” model of sensory processing has been challenged by Ergenzinger et al. (1998), who caused alterations in receptive field characteristics of monkeys by suppressing cortical activity. Changes contributing to the occurrence and severity of phantom limb pain can be found in the cerebral cortex, the brainstem, the thalamus, and the anterior cingulated cortex (Zhang et al. 2005). In the cortex, the process of axonal sprouting, where new nerve endings and new connections between neurons are created, can lead to reorganization, as has been shown in amputation experiments with monkeys (Merzenich et al. 1984; Florence et al. 1998). Reorganization at the level of the thalamus has been shown in human amputees as well as in monkeys (Jain et al. 2008) and seems to be connected to the development of phantom awareness and phantom limb pain: Davis et al. (1998) elicited phantom sensations via thalamic stimulation. Brainstem, thalamus, and the cerebral cortex are connected by a variety of fibers and the alterations that occur in one of these parts after tissue damage could be at least partially responsible for changes in the other structures, although the exact nature and direction of these influences are not yet clear.
In order to better understand this process of cortical reorganization, Merzenich et al. (1984) used microelectrode recordings to measure the cortical activity of adult owl monkeys before and after removal of their digits. They looked at the primary somatosensory (SI) cortex. This is the region on the surface of the brain where the perceptive input from the body is processed and ordered in a somatotopic map. They showed that the areas next to the representation zone of the fingers that had been severed invaded the area that was no longer used. This way, cortical resources that are no longer necessary may be put to a new use and the representation of different body parts in the brain can shift by several millimeters. This shift is not found in patients with congenital limb deficiency (Montoya et al. 1998). A quite large representational shift, in the order of centimeters, was reported by Pons et al. (1991) after they performed a dorsal rhizotomy of the monkey’s arms. They found that the representation of the cheek, which is next to the representational zones of arm and hand in the SI cortex, invaded these adjacent areas. This phenomenon has been postulated as being responsible for certain nonpainful referred sensations (Ramachandran et al. 1992): Some amputees, for example, report sensations in their phantom limb when they touch their cheeks or lips, usually while they are shaving or applying makeup. The connection between changes in the SI cortex and these referred sensations has since been challenged (Grüsser et al. 2001) and they might actually be related to alterations in the premotor cortex and parietal areas (Grüsser et al. 2004). Referred sensations can be recreated in a laboratory setting and their sensational quality is usually maintained, so that, for example, a vibrating stimulus applied to the correct spot on the cheek elicits the feeling of vibration in the phantom limb (Flor et al. 2000).
However, the relationship between referred sensations and phantom limb pain is still unclear. The described sensations are only experienced by a small proportion of amputees (Grüsser et al. 2001), some who may suffer from phantom limb pain and some who will not. It has been shown that the cortical remapping can vary strongly over time (Halligan et al. 1994), whereas phantom limb pain seems to be much more rigid over several years. Also, the shift of the mouth representation in SI into the representational zone of the hand, which occurs in upper extremity amputees, has been shown to be tightly connected to the severity of phantom limb pain (Flor et al. 1995). This means that the intensity of phantom limb pain increases the further the hand zone in SI is invaded by the neighboring mouth representation. The varying extent of cortical reorganization between individuals might be caused by peripheral factors like loss of C-fibers, since those fibers appear to play an important role in maintaining cortical maps (Calford and Tweedale 1991).
Another important point about cortical reorganization is that it occurs in several distinct stages. The first stage is represented by the unmasking of neural connections that are normally inhibited. Mechanisms that are involved in this process are an increased release of excitatory neurotransmitters, a higher density of postsynaptic receptors (so that the same amount of released transmitters results in a stronger excitatory reaction), an altered conductance of neural membranes, decreased inhibitory inputs, and the removal of inhibition from excitatory inputs (Kaas and Florence 1997). This results in a higher cortical excitability in amputees with phantom limb pain (Karl et al. 2004). The second stage involves structural rather than biochemical changes and includes processes such as axonal sprouting and changes in synaptic strength. Another important factor is Hebbian learning, which leads to reorganization based on the usage of certain cell networks or assemblies, as well as long-term potentiation (Elbert et al. 1997). Churchill et al. (1998) propose a use-dependent third stage of cortical reorganization characterized by a refinement of receptive fields.
Perceptual Illusions Related to Phantom Limb Pain
There are several experimental paradigms to create illusory perceptions that shed some light on the workings of phantom limb pain. For example, Botvinick and Cohen (1998) established the so-called rubber hand illusion (RHI), in which one of the participant’s hands is hidden from view and replaced in the visual field by a more or less lifelike artificial hand. The hidden hand and the rubber hand are then stimulated in a synchronous manner using brush strokes or cotton swabs. Thus, the visual and tactile senses report congruently and the rubber hand looks and feels like the participant’s actual hand. In this situation, a feeling of ownership towards the rubber hand is reported and the perceived position of the hidden hand shifts towards the rubber hand. In measurements using functional magnetic resonance imaging (fMRI) during the application of the RHI it has been shown that not only the somatosensory cortex is involved in creating the illusion (as would be suggested by the mechanisms discussed earlier), but also frontal and parietal areas (Ehrsson et al. 2004), suggesting that bottom-up processes of visuo-tactile integration and top-down processes stemming from the representation of the participant’s own body interact to create illusory perceptions such as the RHI (Tsakiris 2010). The crucial factors in creating the illusion of an artificial hand belonging to one’s own body are so far only partially known: On the one hand, Armel and Ramachandran (2003) showed that the visual similarity between rubber hand and actual hand do not seem to be very important. They also noted a feeling of ownership towards a two-dimensional picture of a hand that was projected onto a table. On the other hand, if a rubber hand is used and put into a physically impossible position relative to the participant’s body, the illusion does not occur (Ehrsson et al. 2005).
The effects and workings of the RHI have several implications for neuropsychological aspects of amputations: It shows under which circumstances a more or less external object, like the phantom limb or a prosthetic replacement for the lost limb, will be successfully integrated into the body image. In regard to the integration of prostheses, the results suggest congruent tactile feedback to be crucial. If, for example, a prosthesis collects tactile information (using pressure sensors in the fingertips of the artificial hand) and relays this data to the body by stimulating the stump, the patient’s ability to accept this external object as a part of the body will most probably depend on the temporal and spatial congruence between the visual and tactile modalities. If there is too much discordance between the senses, this incongruence will lead the patient to reject the prosthesis as a part of their body and might even have adverse consequences, as discussed below.
Another illusion with important implications on phantom limb pain has been examined by McCabe et al. (2005): Here, a mirror was put between the arms or legs of healthy participants in such a manner that the limb seen as a mirror image was viewed at the same angle and in the same position as the limb that is hidden behind the mirror. In this setup, when participants are asked to move both limbs in a congruent manner (e.g., lifting both arms or both legs up at the same time), the mirror image behaves exactly like the hidden limb. If, however, the participants are instructed to do incongruent movements (e.g., moving the left arm or leg up while moving the other one down), the mirror image contradicts the body movement, leading to an incongruence between the sensory modalities of vision and proprioception. McCabe et al. (2005) report a variety of subjective reactions to this sensory incongruence, ranging from a general feeling of uneasiness and paresthesias like numbness or tickling to the perception of an altered number of limbs and even to pain. These perceptions only occurred in some participants and did not occur when the mirror was replaced with a whiteboard. The implication for phantom limb pain and related phenomena is that the sensorimotor incongruence caused by an amputation might induce the abnormal sensations that are part of many neuropathic pain syndromes. However, this study was not controlled and did not include measures other than verbal descriptions of subjective sensations.
Functional neuroimaging studies suggest that the activity in primary cortical areas during illusory perception experiments reflects the perceived, subjective input rather than the stimulus that was applied physically (Chen et al. 2003; Blankenburg et al. 2006). This also seems to be the case in the phenomenon of telescoping: This term refers to the feeling, reported by many amputees, that the size and length of the phantom limb change over time, sometimes shortening so far that the phantom fingers are perceived as being attached directly to the stump. In this way, telescoping might be an additional source of sensory incongruence. In the light of the aforementioned strong correlation between SI reorganization and the severity of phantom limb pain, it is not surprising that the extent of telescoping has been found to be associated with phantom limb pain (Grüsser et al. 2001). These studies also indicate that visual, somatosensory and motor feedback to the cortex might not only be an important determining factor of phantom limb pain, but also a potential gateway to effective feedback-based therapies.
Pain Memory
Prospective studies similar to the one by Nikolajsen et al. (1997) have postulated that chronic pain before amputation as a predictor for phantom limb pain, although it has to be pointed out that these studies usually include only a very limited number of traumatic amputees and many patients with long-term pain problems, since they rely on those cases of amputations that can in some way be expected and therefore be evaluated in a prospective manner. There also seems to be a similarity in pain quality between preoperative pain and phantom limb pain, although the likelihood of this similarity has a wide range and seems to depend on the type and time of assessment (Katz and Melzack 1990). The effects of pain memory in phantom limb pain patients may be similar to sufferers from chronic back pain, where the representational area of the back in the SI cortex increases with increased chronicity (Flor et al. 1997). This effect is an example for implicit pain memories: This term refers to central nervous changes regarding nociceptive input that lead to changes in the processing of somatosensory input and do not require changes in conscious processing of the pain (Flor et al. 2003). Thus, long-term noxious input can lead to long-lasting alterations in the cortex, affecting the subsequent processing of somatosensory input. The prominent role of the SI and motor cortex in the processing of phantom limb pain is supported by reports that the surgical removal of parts of this cortex abolishes phantom limb pain, while the stimulation of this area can elicit it (Head and Holmes 1911; Appenzeller and Bicknell 1969; Knotkova and Cruciani 2010). With an established pain memory in this cortex, the invasion of the cortical amputation zone by neighboring areas might preferentially activate neurons coding for pain. Because these neurons used to be assigned to the limb that has been amputated, the responding sensation is felt in this limb (Doetsch 1998).
As a result of these findings and the idea that central anesthesia might not be sufficient protection against the afferent nociceptive input during amputation, peripheral anesthesia has been added for some time before and during surgery to prevent central sensitization (Woolf and Chong 1993). The effectiveness of this approach, however, has been challenged by several studies (e.g., Nikolajsen et al. 1997). That said, NMDA receptor antagonists given during the operation and the postoperative weeks might be beneficial (Wiech et al. 2001; Schley et al. 2007). These neurotransmitters are expected to erase preexisting somatosensory pain memories and inhibit the creation of new ones.
The role of individual preexisting sensitivities including psychological and genetic susceptibility in the processing of nociceptive stimuli might also account for some of the observed interindividual variation in the proneness to develop phantom limb pain after amputation. This is also suggested by a study showing a connection between pain thresholds relating to pressure before the amputation and the occurrence of postoperative phantom limb pain (Nikolajsen et al. 2000). Since it is unclear which phases of the life course and which pain experiences have the largest influence on this sensitivity, additional data from longitudinal studies including those that highlight the role of biobehavioral factors to better understand the role of pain memory in the development of phantom limb pain are warranted.
Therapy
One can easily imagine that a phenomenon with such a wide range of diverse and complex origins and contributing factors will be quite resistant to simple therapeutic approaches (Sherman et al. 1980). Phantom limb pain has been treated by medication (including barbiturates, muscle relaxants, antidepressants, anticonvulsants, or neuroleptics) or other somatic interventions (such as local anesthesia, sympathectomy, dorsal root entry zone lesions, or neurostimulation), but the maximal beneficial effect of these strategies did not exceed about 30% and is therefore not higher than the placebo effect that has been reported in other studies (Finnerup et al. 2007). There are alternatives in the form of behavioral therapeutic approaches to chronic pain which will not be discussed in detail here since they are not specific to phantom limb pain (see also Donovan et al. 2011). They include treatments based on operant learning, cognitive-behavioral therapies, biofeedback, and relaxation as well as extinction training (Flor and Diers 2007).
Pharmacological Treatments
In animal models, cortical reorganization could be prevented and reversed using NMDA receptor antagonists or GABA agonists, both of which work by disrupting the mechanisms of cortical reorganization. In human phantom limb pain patients, short-term pain alleviation was achieved using the NMDA antagonist ketamine (Eichenberger et al. 2008). However, the NMDA receptor antagonist memantine was not effective (Maier et al. 2003). A reduction of phantom limb pain has been shown in several studies regarding the effect of opioids (see Huse et al. 2008). Certain pharmaceutical treatments seem to be effective only in patients with a specific cause of phantom limb pain: The anesthetic lidocaine improved the situation of patients with neuromas (Chabal et al. 1992), whereas patients with a strong contribution of peripheral factors seem to benefit from biofeedback treatments which aim for vasodilation of or decreased muscle tension in the residual limb (Sherman et al. 1997). A recent study noted that pain alleviation in sufferers from phantom limb pain after administering duloxetine, an antidepressant, and pregabalin, an anticonvulsant, with each drug influencing a different part of the nociceptive pathway (Spiegel et al. 2010). Gabapentin, a widely used treatment for neuropathic pain (Baillie and Power 2006), was not shown to reduce stump pain or phantom limb pain in a controlled study (Nikolajsen et al. 2006).
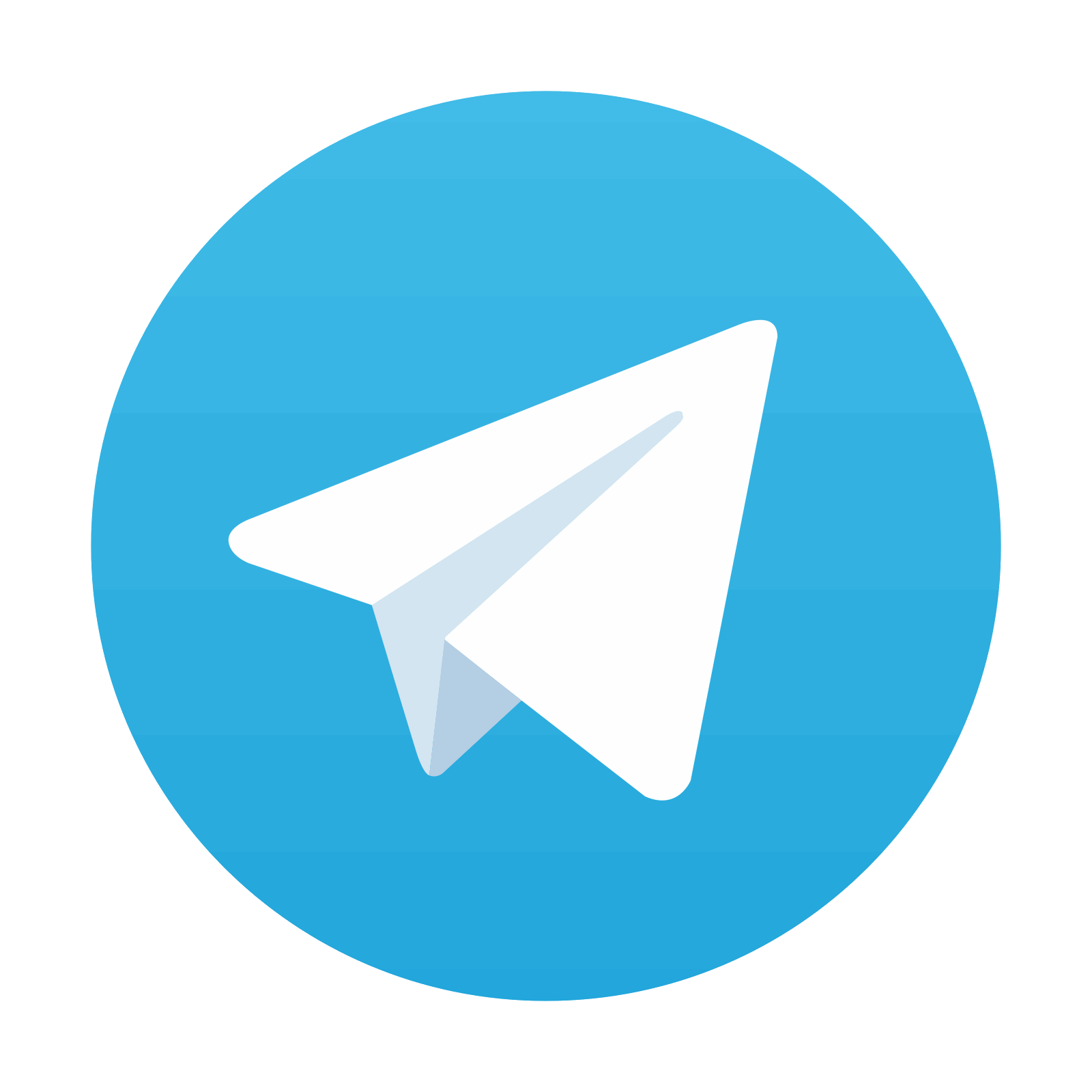
Stay updated, free articles. Join our Telegram channel

Full access? Get Clinical Tree
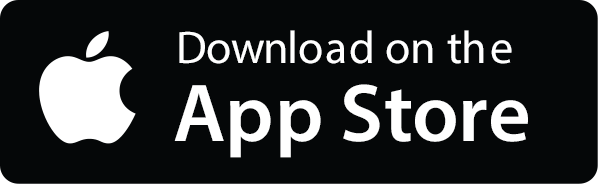
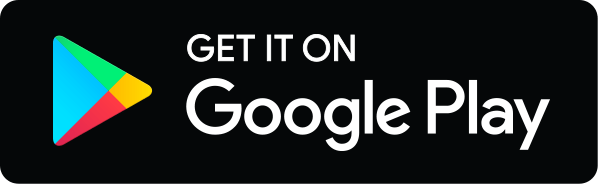