The transesophageal echocardiography (TEE) machine records the electrocardiogram (ECG) simultaneously with all TEE imagery. This is done so that echocardiographers can correlate echo images throughout systole and diastole. Alterations in rhythm can have sweeping and at times ultimately fatal hemodynamic consequences. Thus, the rapid interpretation of abnormal rhythms and their correction is critical in cardiac anesthesia practice.
THE ELECTROCARDIOGRAM
The ECG remains one of the main monitors used by anesthesiologists. It is primarily employed in anesthesia practice to detect heart rate and rhythm changes, and perioperative myocardial ischemia. The ECG detects electrical currents flowing through the body generated by the electrical activity of the heart. ECG leads are positioned throughout the body and provide various perspectives (depending upon where the lead is placed) of the electrical activity of the heart. Examining the ECG in multiple leads provides the anesthesiologist the ability to discern if perceived changes in ECG pattern are widespread (found in multiple leads) or are perhaps less significant (motion artifact). At the end of diastole, the atria contract providing the atrial contribution to the patient’s cardiac output generating the “P” wave. Following atrial contraction, the ventricle is loaded awaiting systole. Systole commences at the QRS beginning with isovolumetric contraction following a 120 to 200 milliseconds conduction delay at the AV node. Subsequently, intracavitary pressure builds, the atrioventricular valves (eg, mitral or tricuspid) close, and the arterioventricular valves (eg, aortic, pulmonic) open resulting in ventricular ejection of the stroke volume (SV). The QRS represents the electrical activity generated by the depolarization of the left and the right ventricles. Depolarization proceeds from the AV node through the interventricular septum via the His-Purkinje fibers. The QRS segment lasts approximately 120 milliseconds. Repolarization of the ventricles produces the ST segment and the T wave. Electrolyte abnormalities (eg, hypocalcemia) and drug effects (eg, droperidol) can delay repolarization leading to a prolonged QT interval. This can result in potentially life-threatening ventricular arrhythmias.
ECG ABNORMALITIES
Electrolyte disorders, heart structure abnormalities, and myocardial ischemia can cause aberrations in the patient’s baseline ECG without producing an arrhythmia per se (Figure 3–1). Electrolyte abnormalities occur with some frequency perioperatively. Hyperkalemia can present following cardioplegia administration during cardiopulmonary bypass (CPB), following iatrogenic administration, or associated with metabolic acidosis. As the potassium concentration increases, the T wave becomes progressively peaked. Hyperkalemia can ultimately produce broad, complex ventricular activity and asystole. Treatment is with immediate administration of calcium chloride. Glucose and regular insulin are given to lower the potassium concentration.
Hypokalemia lengthens the QT interval placing the patient at risk for les torsades de pointes type of sinusoidal ventricular fibrillation.
Hypocalcemia and hypomagnesemia likewise can prolong the QT interval placing the patient at risk for ventricular fibrillation. Hypermagnesemia is associated with various conduction abnormalities and is seen from time to time in the obstetrical patient being treated for preeclampsia. Hypercalcemia can also cause T-wave abnormalities.
Ventricular hypertrophy, cardiomyopathies, and inherent conduction disorders can all alter the baseline ECG. Increased QRS size in the left precordial leads (eg, V5, V6) may indicate the presence of left ventricular hypertrophy associated with increased afterload and/or obstruction to left ventricular outflow tract (LVOT) ejection. Conversely, tall QRS waves in the right precordial leads (eg, V1, V2) might indicate right ventricular hypertrophy secondary to pulmonary hypertension.
Right and left bundle branch blocks in addition to various prolongations in the QRS are evidence of impaired cardiac conduction and often herald the presence of cardiac disease.
ST-segment elevations and depressions are associated with transmural and subendocardial ischemia, respectively. Pathologic (wide and deep) Q waves suggest myocardial infarction. TEE imagery demonstrating poorly contractile myocardium can be compared with ECG tracings to identify and to confirm the presence of ischemic myocardium.
Figure 3–1A. Common ECG findings during cardiac surgery
Access to the chest is limited during cardiac surgical procedures. Since ECG interpretation is critical throughout the procedure it is very important that the leads be securely fixed to the patient to avoid having to replace them during the operation. ECG tracings should be clear at the start of the case so to facilitate rhythm interpretation intra-operatively.
BRADYARRHYTHMIAS
Bradycardia and bradyarrhythmias routinely complicate the perioperative management of the cardiac surgery patient. Hyperkalemia, myocardial ischemia, vagal effects, intrinsic cardiac disease, hypoxemia, loss of sympathetic tone can all contribute to the development of varying degrees of heart block and/or sinus bradycardia at any time during heart surgery.
Figure 3–1B. AV sequential and atrial pacing are frequently employed during cardiac surgery as various degrees of heart block are often encountered perioperatively. Prolongation of the PR interval is seen in first-degree heart block here associated with the prolonged QRS complexes often seen during bundle branch blocks. In second-degree heart block the PR interval gradually lengthens until the impulse is no longer conducted to the ventricles. In third-degree block there is no association between atrial and ventricular beats. The atrial and the ventricular contraction are not coordinated.
Sinus bradycardia (sinus rate less than 60 bpm) frequently is associated with anesthesia induction, narcotic administration, a reflex response to systemic hypertension, and beta blockade. Sinus bradycardia is of little consequence assuming cardiac output is sufficiently preserved to maintain an acceptable blood pressure and tissue perfusion.
Recall,
Blood pressure = Cardiac output × Systemic vascular resistance
Cardiac output = Stroke volume × Heart rate
Therefore, if the heart rate falls—even if sinus rhythm is maintained—the patient may become hemodynamically unstable. Too slow a sinus rhythm provides other pacemaker cells in the heart the opportunity to emerge resulting in various other nodal or ventricular arrhythmias. Additionally, too slow a heart rate increases the diastolic filling time resulting in a prolonged filling of the left ventricle, increased left ventricular end-diastolic volume, and depending upon left ventricular compliance, increased left ventricular end-diastolic pressure (LVEDP). Reduced endocardial perfusion may occur.
Recall,
Coronary perfusion pressure (CPP) = Diastolic blood pressure (DBP) – LVEDP
Treatment for sinus bradycardia must be determined on a case-by-case basis depending upon the patient’s hemodynamic performance. Bradycardia in response to systemic hypertension is corrected by lowering the blood pressure. Sinus brady-cardias resulting from anesthesia induction may need to be treated if they result in hypotension and low cardiac output. Atropine (IV) 0.4 to 2.0 mg can be administered to correct the heart rate. The causes of sinus bradycardia should be sought and corrected. A slowing heart rate in the setting of hypoxemia is immediately worrisome and may indicate impending circulatory arrest. Ephedrine (5-10 mg IV) can be judiciously administered to the heart surgery patient experiencing hypotension and sinus bradycardia as a consequence of anesthesia induction. However, ephedrine’s reliance upon release of native catecholamine reserves may make it a poor choice in the catecholamine-depleted patient. Likewise, various inotropic infusions (eg, epinephrine [2 μg/min IV] infusion and upward, dobutamine [2-10 μg/kg/min] etc) can be administered to increase the patient’s heart rate. Of course, administration of catecholamines as well as atropine might lead to the development of accelerated nodal or ventricular rhythms. Temporary pacing (see later) can be used where available and when indicated to provide atrial, ventricular, or atrial-ventricular pacing as needed.
At times, patients will present with competing atrial pacemaker cells each resulting in an atrial driven rhythm albeit at different rates and with differing “P”-wave morphologies. Nodal rhythms emanate from the AV node and result in inadequate filling of the left ventricle due to the absence of a coordinated atrial contraction. The contribution of the atrial beat to effectively load the left ventricle at the end of diastole varies from patient to patient depending upon age and left ventricular compliance and atrial elastance. The less compliant the left ventricle the more dependent it becomes upon the contribution of the atrial contraction. When nodal rhythms emerge hemodynamic consequences may be nil or great depending upon the individual patient and their degree of diastolic dysfunction. Patients with a slow nodal rhythm may respond to administration of atropine and inotropic agents in a manner similar to the treatment of sinus bradycardia. On the other hand, some nodal rhythms develop into AV junctional tachycardia and require treatment as described below for supraventricular tachycardias.
Varying degrees of heart block are also quite common in the cardiac surgery patient. Impairment of the conduction system occurs frequently perioperatively secondary to ischemia, electrolyte disorders, and iatrogenic, surgically mediated injury to the heart’s conduction system as it passes through the intraventricular septum. First-degree heart block occurs when there is a prolongation in the AV interval. Second-degree heart block occurs when not all of the atrial beats are conducted through the AV node to the ventricle. Mobitz type 1 block or Wenckebach block is associated with a gradual prolongation of the AV interval until a ventricular beat fails to occur. Often Mobitz type 1 block requires no direct therapy. Conversely Mobitz type 2 block has a propensity to deteriorate to third-degree heart block. In this form of second-degree atrioventricular block the atrial pacemaker beat is not always conducted through to the ventricle. Sporadically, atrial contractions can occur without a ventricular beat. In third-degree heart block no atrial beats are conducted through to the ventricle. A slow ventricular pacemaker may emerge leading to the atria and ventricles contracting in a discordant fashion. The “P” waves can be seen marching through the ECG tracing without an associated QRS immediately thereafter. Hemodynamic instability often becomes manifest and temporary pacing is frequently required perioperatively.
Left and right bundle branch blocks are very common in heart surgery patients as they can portend the presence of intrinsic cardiac disease. In patients with a left bundle branch block pulmonary artery catheterization can result in a mechanically induced concomitant right bundle branch block producing complete heart block and hemodynamic instability. A temporary pacing ability (eg, external cutaneous pacing) should be available whenever right heart catheterization is planned in the left bundle branch block patient.
TEMPORARY PACEMAKER
The ability to provide temporary pacing is essential in cardiac anesthesia practice. Bradyarrhythmias as described earlier can present at any point in the patient’s peri-operative course. Many patients require some form of pacing to separate from cardiopulmonary bypass even if only for a few minutes until the heart is reperfused and/or the potassium concentration reduced. Transient perioperative ischemia can also produce varying degrees of heart block necessitating some form of emergent pacing. Temporary pacing must be distinguished from permanent pacing. Permanent pacemakers are increasingly being implanted in patients for the treatment of heart failure.1 Permanent biventricular pacing provides a more effective myocardial contraction in the heart failure patient by reducing the width of the QRS complex. Additionally, many permanent pacemakers contain the ability to sense an increase in respiratory rate and thus are able to increase the pacing rate in response to exercise. Finally, with an ever-increasing number of patients at risk for sudden cardiac death (SCD), these devices contain a defibrillation or anti-tachycardia function permitting electrical conversion of malignant rhythms (ICDs). Permanent pace-maker/defibrillators are discussed in Chapter 15.
Temporary pacemakers in general are designated by a three-letter code relating to their function: The first letter identifies the chamber or chambers of the heart paced (A for atrium, V for ventricle, D for dual [both A+V] and O for none). The second letter identifies which, if any, of the chambers are sensed. The third letter indicates the response of the device to a sensed beat (I for inhibited, D for both inhibition and triggering). Thus, a temporary VVI pacemaker would pace the ventricle, sense the ventricle, and be inhibited if a ventricle beat was detected. Likewise a DDD temporary pacemaker would pace both the atrium and the ventricle, sense both chambers, and either be inhibited or deliver a beat depending upon what the device senses.
There are many different modes of temporary pacing available to anesthesiologists perioperatively. Transcutaneous pacing is usually available as a part of most modern cardioverter/defibrillator devices. Pacing/defibrillation patches are applied to the chest and ventricular pacing initiated by setting the pacing rate and the output from the device until the heart is captured and a beat generated. Transcutaneous pacing is ventricular pacing and as such the atrial contribution to the cardiac output is lost. Often pacing patches are placed prophylactically in cardiac surgery patients in order to provide for a quick pacing capability should the rhythm be lost before the heart is exposed and epicardial pacing wires placed. In reoperative heart surgery patients, access to the heart may be difficult thus preventing rapid sternal opening and application of epicardial pacing wires. Placement of transcutaneous pacing pads perioperatively is especially suggested in these cases to provide for a temporary ventricular beat.
Temporary esophageal pacing systems can be used to pace the atrium. They have limited application in the heart surgery patient since this atrial pacing modality (AOO) does not provide for ventricular capture. Moreover, a TEE probe frequently occupies the esophagus during cardiac surgical procedures.
Temporary epicardial pacing has been used in cardiac surgery since the 1960s.2 Surgeons routinely place epicardial pacing wires to provide for both atrial and ventricular pacing. These wires are passed from the surgical field to the anesthesiologist and attached to a pulse generator. The anesthesiologist next chooses the pacemaker mode and settings. Generally, the DDD mode is chosen to provide for temporary pacing. To set the pacemaker the anesthesiologist selects:
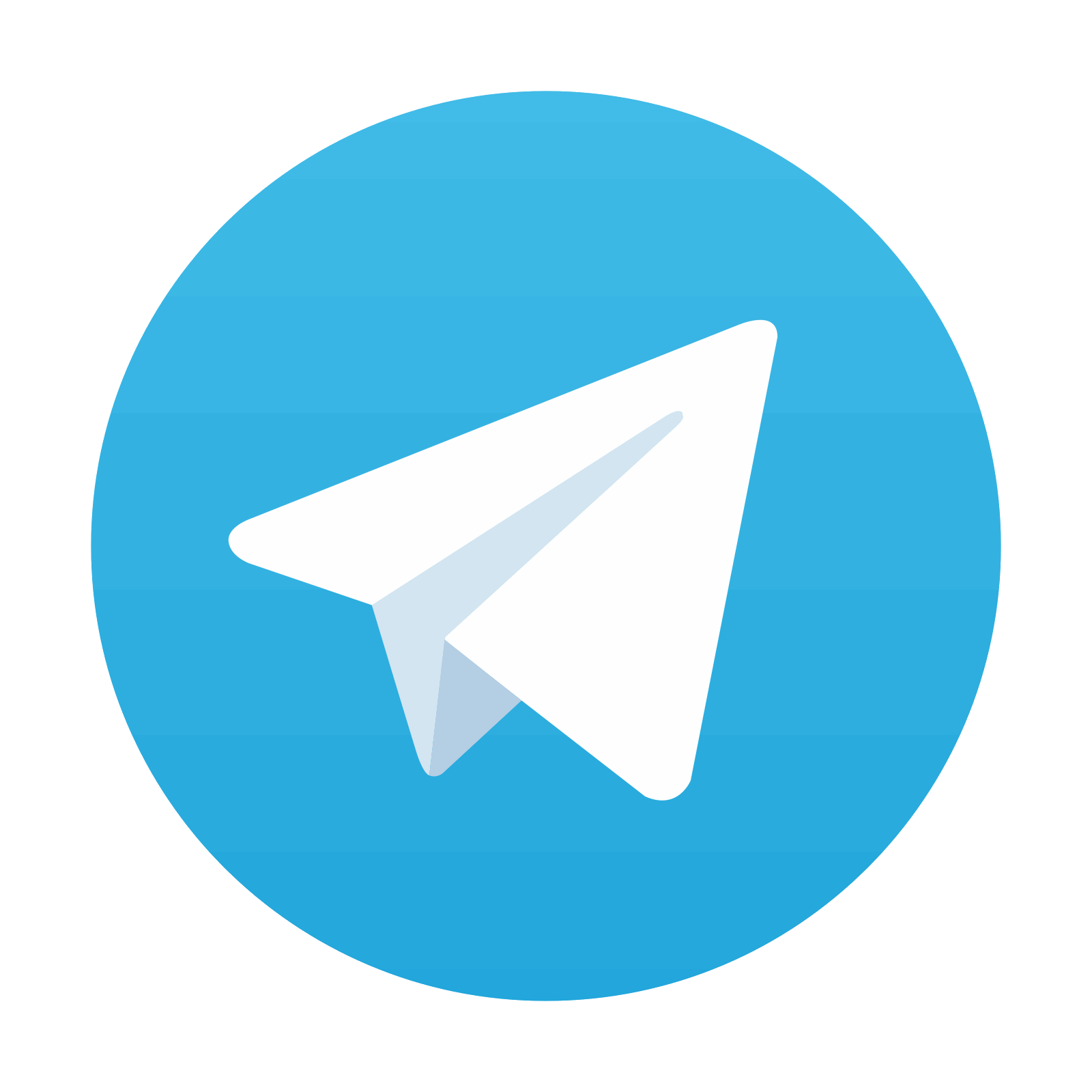
Stay updated, free articles. Join our Telegram channel

Full access? Get Clinical Tree
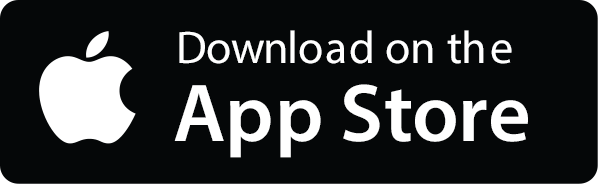
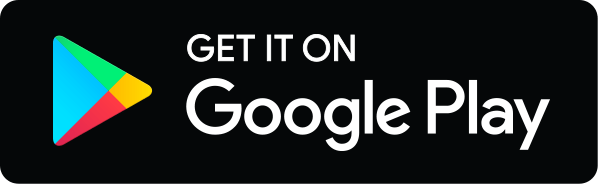