Perioperative Management of the Patient with Pulmonary Hypertension
E. Andrew Ochroch
Pulmonary hypertension is a common but underappreciated pathological condition in patients who undergo thoracic surgery, and being unaware of this condition or underestimating its severity can lead to significant perioperative complications. Management of this condition requires foresight and preparation as discussed in this chapter. Thus, this chapter will serve to: (1) review the pathogenesis of pulmonary hypertension, (2) describe the preoperative preparation and evaluation of patients with pulmonary hypertension who present for thoracic surgery, (3) explain the effects of anesthesia and surgery on the pulmonary vasculature, and (4) define the proper use of the pharmacological aids to control pulmonary hypertension.
NORMAL PULMONARY PHYSIOLOGY AND HYPOXIC PULMONARY VASOCONSTRICTION
At rest, the normal pulmonary vascular system is noted as a high-compliance, high-flow, low-pressure system. This stands in contrast to the systemic circulation, which has much higher resting level of arterial and venous tone. This difference stems partly from the anatomy because the pulmonary precapillary arterioles have a thinner media and less smooth muscle than their systemic counterparts. Furthermore, at rest, there are far more recruitable vessels in the pulmonary bed, which permits dramatic increases in flow with minimal impact on pressure.
The difference between the systemic and arterial systems is also due to the response of the pulmonary vascular endothelium to the challenges of hypoxia (hypoxic pulmonary vasoconstriction: HPV). This vasoconstriction is known as the Euler-Lijestrand reflex. Mitochondria play a key role as the primary sensor of hypoxia, with intracellular calcium increasing as a key response, but the basic mechanism is controversial.1 Figure 4–1 displays several of the key pathways for hypoxic vasoconstriction in which voltage gated potassium (K+) channels directly alter mitochondrial responses. L-type calcium (Ca2+) channels are facilitated by the depolarization of the K+ channels; they then directly increase intracellular Ca2+. Further, classical transient receptor potential channel 6 (TRPC6) also increases intracellular Ca2+ as do store operated channels (SOC) and sodium (Na+)/Ca2+ exchangers (NCX).1 This rise in intracellular Ca2+ also triggers release from sarcoplasmic reticulum via activation of the ryanodine receptors. The end result is constriction of the smooth muscle of the precapillary sphincters and pulmonary arterioles. This calcium-dependent vasoconstriction is the primary phase of HPV and lasts 15 to 30 minutes. The Ca2+ independent phase (the sustained phase) of pulmonary vascular constriction starts at 15 minutes and can last for hours. It is highly dependent on RhoA/Rho kinase (ROCK) mediated Ca2+ sensitization,2 and may be a key to the development of pulmonary hypertension.3 Interestingly, nitric oxide (NO)-induced relaxation and endothelin-1-induced vasoconstriction of pulmonary arteries have been shown to be due to regulation of ROCK-mediated Ca2+-sensitization, rather than altered Ca2+ metabolism.4,5 See Figure 4–1.
Figure 4–1. Ca2+ mobilization in HPV: mechanisms that have been implicated in the hypoxia-induced elevation of [Ca2+], and their potential signaling pathways. Note that some mechanisms are probably only applicable to one phase of HPV (see text). Not shown for clarity: possibility of functionally different types of SOC and Ca2+ store. Note that Na+ entry via NSCC would also contribute to depolarization. y: action of Mg2+ and ATP on KV channels depends on membrane potential (see text). DAG, diacylglycerol; cADPR, cyclic ADP ribose; depol, depolarization; KV, voltage-gated K+ channels; L-type, voltage-gated Ca2+ channels; NCX, Na+– Ca2+ exchanger; RyR, ryanodine receptors; SOC, store-operated channels. TRPC6, classical transient receptor potential channel 6. (From: Ward J, McMurtry I. Mechanisms of hypoxic pulmonary vasoconstriction and their roles in pulmonary hypertension: new findings for an old problem. Curr Opin Pharmacol. 2009;9(3):287-296, with permission. Copyright © Elsevier.)
Anesthetics can influence pulmonary vascular function and inhibit HPV. Inhalational agents (halothane, enflurane, isoflurane, desflurane, and sevoflurane) will all inhibit HPV, but at concentrations of greater than 1 MAC.6 However, intravenous anesthetics such as propofol have a less profound impact on HPV than the inhalational agents.7 Opioids, benzodiazepines, and epidural analgesia/anesthesia have minimal impact. For further discussion on the effect of anesthetic drugs on HPV see Chapter 3.
PULMONARY HYPERTENSION
The pathologic changes of pulmonary hypertension (PH) stem directly from a derangement of the basic mechanisms that lead to HPV. The increased vasoconstriction of the pulmonary arteries in PH has been attributed to a reduced expression or activity of voltage-gated K+ channels and dysfunction of the endothelium and platelets; this leads to imbalances in the production and release of both vasodilators such as NO and prostacyclin (PGI2) as well as vasoconstrictors such as endothelin-1 (ET-1) and serotonin (5-HT).8 Many of these regulators of vascular tone also have roles in the control of cell proliferation and apoptosis and thus may also contribute to the characteristic remodeling of the pulmonary vasculature in PH.3 Much of the pharmacologic management of PH is directed toward modulating these known mechanisms.
Pulmonary hypertension is diagnosed by measurements indicating that mean pulmonary arterial pressure (mPAP) exceeds 25 mm Hg at rest and 30 mm Hg during exercise. To make the diagnosis of primary PH left ventricular end diastolic pressure or pulmonary capillary wedge pressure has to be ≤15 mm Hg and the pulmonary vascular resistance (PVR) greater than 3 Wood units (mm Hg/L: 1 Wood unit = 80 dyn.cm.sec-5).9 Secondary PH is due to the increase in pulmonary vascular impedance from intrinsic parenchymal lung disease. Increased PVR, which requires the right ventricle (RV) to raise pulmonary artery pressure (PAP) to maintain cardiac output, can cause failure of the afterload-intolerant RV, ultimately leading to death. Median survival time for untreated idiopathic PH (formerly included in primary PH) in a historical series was 2.8 years.10
There are a myriad of causes of PH, and the World Health Organization’s list of causes is shown in Table 4–1.11 The most common cause of PH in patients presenting for lung cancer resection is cigarette smoking. Acute exposure to tobacco smoke increases vasoconstriction through multiple routes including increased expression of endothelin-1 (ET-1).12 Tobacco smoke also causes a widespread injury to the lung with epithelial and endothelial apoptosis and necrosis.13,14 Further, the normal healing mechanisms for the lung are inhibited by apoptosis of alveolar macrophages and release/expression of other inflammatory mediators.15 The “earliest” pathology in the lungs of smokers is the development of intimal thickening of pulmonary arteries, the severity of which is correlated to the pack-year history.16 Thus, the lung and vascular changes seen in PH associated with chronic obstructive pulmonary disease (COPD) may represent alternate pathways and responses to the damage of tobacco smoke.17
Table 4–1. World Health Organization Classification of Pulmonary Hypertension
EFFECTS OF PULMONARY RESECTION ON RIGHT VENTRICULAR AND PULMONARY VASCULAR DYNAMICS
Lung resection reduces the available cross section of pulmonary vasculature. This is typically very well-tolerated at rest. However, when post-thoracotomy patients are examined in detail, cardiac output and stroke volume decrease, while pulmonary and systemic resistance increase.18 One study examined 40 “normal” subjects and 40 subjects with PH or restrictive pulmonary disease for thoracotomy utilizing transthoracic echocardiography and PA catheter data, preoperatively and postoperatively. They found that preoperatively the subjects with PH had higher right ventricular end diastolic volume (RVEDV), lower RV ejection fraction (RVEF), and higher pulmonary artery wedge pressures. After lobectomy or bi-lobectomy, all subjects demonstrated a rise in RVEDV, PVR and wedge pressure with a fall in RVEF. Subjects with preexisting PH or restrictive lung disease had a greater rise in RVEDV, PVR and wedge and a greater fall in RVEF than “normal” subjects.19 The authors comment that the rise in RVEDV and RV end diastolic pressure in subjects with PH caused a trend toward new postoperative wall motion abnormalities in the RV, suggesting that the myocardium was at significant risk.
PREOPERATIVE MEDICAL MANAGEMENT OF PATIENTS WITH PULMONARY HYPERTENSION
Many patients with PH receive warfarin, diuretics, digoxin, and oxygen. These treatments are often referred to as background or conventional therapy. Diuretics and digoxin provide symptomatic relief but are not thought to affect the course of the disease. Warfarin might provide a survival advantage due to altered platelet and endothelial function in PH, but its contribution is difficult to estimate.20 Calcium channel blockers, such as nifedipine, offer considerable benefit to the patients that respond to them, although these account for only around 6% of patients with PH.21
Patients with PH have reduced production of prostacyclin (PGI2), a product of the arachidonic acid cascade that promotes vasodilation and inhibits vascular proliferation and platelet aggregation.22 Activation of the prostacyclin receptor by PGI2 produces activation of the enzyme adenylate cyclase, increased intracellular cyclic adenosine monophosphate levels, and opening of Ca2+-activated K+ channels.23 Increased K+ conductance produces hyperpolarization of the cell membrane, blockade of L-type Ca2+ channels, and decreased cytosolic Ca2+. The net result of this process in the vascular smooth muscle cell is relaxation with consequent vasodilation. Epoprostenol was the first PGI2 replacement to be studied; in addition to symptomatic improvement, it has been shown to offer a survival advantage.22,24,25 However, its administration (intravenous, via an indwelling catheter) is complex and it causes adverse effects such as decreased platelet function, hypotension, headache, nausea, and diarrhea.
Other prostanoid analogues have since been studied, including iloprost (inhalation),26 treprostinil (IV)27 and beraprost (oral).25 The duration of hemo-dynamic effect of nebulized iloprost averages approximately 90 minutes after inhalation; the drug requires 6 to 9 nebulizer treatments per 24 hours, with each treatment requiring up to 10 to 20 minutes. While iloprost improved 6-minute walk performance similar to epoprostenol, its use is cumbersome for outpatients. Treprostinil was developed as a longer acting intravenous epoprostenol, so that brief interruptions of the delivery would not have dramatic consequences. Beraprost is an oral drug that is under evaluation. Data from clinical trials support the short-term benefits of these drugs22 but their cost-effectiveness has been challenged by the United Kingdom’s National Institute for Health and Clinical Excellence.28
As previously discussed, in PH there is an imbalance between endogenous production of vasodilators and vasoconstrictors, with decreased levels of PGI2 and increased levels of endothelin-1.22 Endothelin-1 is a vasoconstrictor which acts via two receptors, ETA and ETB to both regulate vascular tone and cell proliferation. Both receptor subtypes are found on vascular smooth muscle cells and their activation causes vasoconstriction, probably by augmenting Rho kinase Ca2+ sensitivity,4 while the ETB receptor activation on endothelial cells increases NO and prostacyclin release. There are three clinically available ET blockers: bosentan, a nonspecific blocker, and ambrisentan and sitaxsentan, which selectively block ETA. These drugs improve 6-minute walk distance, improve functionality and delay progression of PH.29 Hepatotoxicity limits their usefulness in some patients, and anemia is common. These drugs are eliminated through the cytochrome P450 system, and thus may alter anesthetic drug half-life.29
Pulmonary vascular tone can also be reduced through phosphodiesterase inhibitors. Sildenafil is a selective inhibitor of phosphodiesterase type 5 (PDE5). Present throughout the body, PDE5 is found in high concentrations in the lungs. Inhibition of PDE5 enhances the vasodilatory effects of nitric oxide in PH by preventing the degradation of cyclic guanosine monophosphate (cGMP).30,31 When added to conventional therapy, sildenafil increased exercise capacity in patients with PH due to both decreased PVR and improved ventilation-perfusion matching.31 Sildenafil was also associated with improvements in the World Health Organization functional class and hemodynamic parameters. Sildenafil has a short half-life, and it requires dosing three times a day for clinical effectiveness.
PREOPERATIVE PREPARATION
Preoperative preparation of patients with lung cancer is typically foreshortened due to the threat of metastasis. Several reviews and meta-analyses have focused on perioperative pulmonary complications and strategies to reduce morbidity and mortality. Collectively, these reviews indicate that most risk factors are not modifiable; the surgical incision site has been identified as the single most important risk factor, with thoracic incisions placing the patient at significant risk (odds ratio [OR] of 4.24) for perioperative morbidity and mortality.32–34 Age was the most important patient-related predictor with postoperative pulmonary complication rates being linearly related: age 50 to 59: 6.1%, age 60 to 69: 8.1% and 70 to 79: 11.9%.35 In a multivariate model that adjusted for cardiac and pulmonary comorbidities, the OR of postoperative pulmonary complication also varied by age 50 to 59 years OR 1.5 (CI 1.31–1.74), 60 to 69 OR 2.28 (CI 1.86–2.8), and 70 to 79 OR 3.9 (CI 2.7–5.7).33 Traditional spirometry did not stratify risk, and smoking cessation within 8 weeks of surgery did not impact risk.32–34
Although it is important to consider the risk of pulmonary complications in patients undergoing thoracic operations, epidemiologic studies have shown that perioperative cardiac complications remain the leading cause of death after anesthesia and surgery.36–38 Most of the data regarding perioperative myocardial risk comes from studies of noncardiac surgical patients, of whom thoracic surgical patients were only a small proportion. For example, The Coronary Artery Surgery Study (CASS) registry reported 1600 operations over a 3-year period. However, only 89 of the 1600 were thoracic operations.39 Consequently, the published estimates of perioperative cardiac morbidity do not address the risks incurred specifically from the perturbations of the thoracic procedure itself on the typical patient presenting for thoracic surgery. Instead, they address the average risk of a patient with coronary risk factors undergoing either high-risk or low-risk procedures. While few studies have addressed the incidence of perioperative cardiovascular complications in thoracic surgical patients selectively, it is likely that cardiovascular events are a major cause of perioperative mortality in this subgroup. Further discussion on cardiac and pulmonary complications following thoracic surgery procedures may be found in Chapter 24.
Pulmonary Rehabilitation
Pulmonary rehabilitation combines optimizing bronchodilator management, improving anti-inflammatory therapy, treating preexisting pneumonia/atelectasis, increasing exercise capacity, and optimizing oxygen therapy. Typical regimens last 4 to 9 weeks. Although lung mechanics are usually not altered, exercise capacity, severity of dyspnea and quality of life are improved.40 One small trial of pulmonary rehabilitation in patients with Stage 1 lung cancer indicated that while exercise capacity increased, no discernable effect on perioperative morbidity was found.41 Specifically for PH, only prolonged oxygen therapy has been shown to stop the progression of the disease, but not to reverse the condition.42
Determination of Presence of Pulmonary Hypertension
Pulmonary hypertension is an uncommon and underdiagnosed disease. Although the effect of PH on perioperative outcome of thoracic procedures has not been extensively studied, preoperative diagnosis should promote optimal intra- and postoperative care. Determining whom to evaluate for PH is difficult, because the typical presenting symptoms of PH, such as breathlessness, decreased exercise tolerance, and fatigue, are nonspecific and easily attributable to COPD. In patients with COPD significant enough to be evaluated for lung volume reduction surgery, pulmonary function tests did not distinguish between those with and without PH. A study by Bach et al, determined that PaO2 while breathing room air was significantly lower in PH patients (51.5 +/– 1.4 vs 69 +/– 1.3 mm Hg, p = 0.000008), but this finding had a poor positive and negative predictive value.43 Similarly, end tidal CO2 did not distinguish those with and without PH. However, this study only examined the criteria for PH at rest. When patients with significant or severe COPD were examined for PH during exercise, one-third met criteria with mean PA pressures greater than 30 mm Hg.44 Consequently, patients with COPD may have limited cardiopulmonary reserve to deal with exercise or perioperative challenges due to rises in pulmonary artery pressures.
In patients with PH, or those suspected of it, further testing may help to delineate risk. Trans-thoracic echocardiography (TTE) can provide significant data on cardiac function. Of 207 subjects with significant COPD studied prior to surgery with TTE, 98% had adequate studies of right and left ventricular wall motion. Right heart abnormalities were found in 40% of subjects and included right atrial enlargement (32%), right ventricular hypertrophy (12%), and RV systolic dysfunction (7%).43 Unfortunately, pulmonary arterial pressure could not be consistently measured as it relies on detection and measurement of tricuspid regurgitant jet, not attainable in all patients.
Right heart catheterization has also been used in an attempt to better assess risk. Patients who had exercise induced decreases in RVEF were at significantly greater risk for developing postoperative cardiopulmonary complications than patients who had exercise induced increases in RVEF.45 The authors claim that decreases in RVEF in response to exercise indicated a limited cardiopulmonary reserve. Further reduction of the pulmonary vascular bed after lung resection may stress this limited reserve leading to an increased risk of cardiopulmonary decompensation and complications.45 Similarly, unilateral pulmonary artery occlusion has been used to determine if the right heart can compensate for a decrease in the vascular bed by assessing the subsequent rise in total pulmonary vascular resistance.46 Unfortunately, both of these methods are highly invasive and risky, and neither have been prospectively evaluated in randomized, blind studies; neither methods are commonly used.
There are no studies in which pulmonary pressures have been linked to outcome after thoracic surgery. The oft-quoted cutoff of 35 mm Hg for maximal pulmonary pressure for pulmonary resection candidates is an arbitrary number that originated from guidelines for lung volume reduction surgery patients.45 It remains unclear if there is a better test to determine likelihood of postsurgical survival than having the patient walk two flights of stairs, as it is primarily a broad test of cardiopulmonary reserve.
PERIOPERATIVE CARE
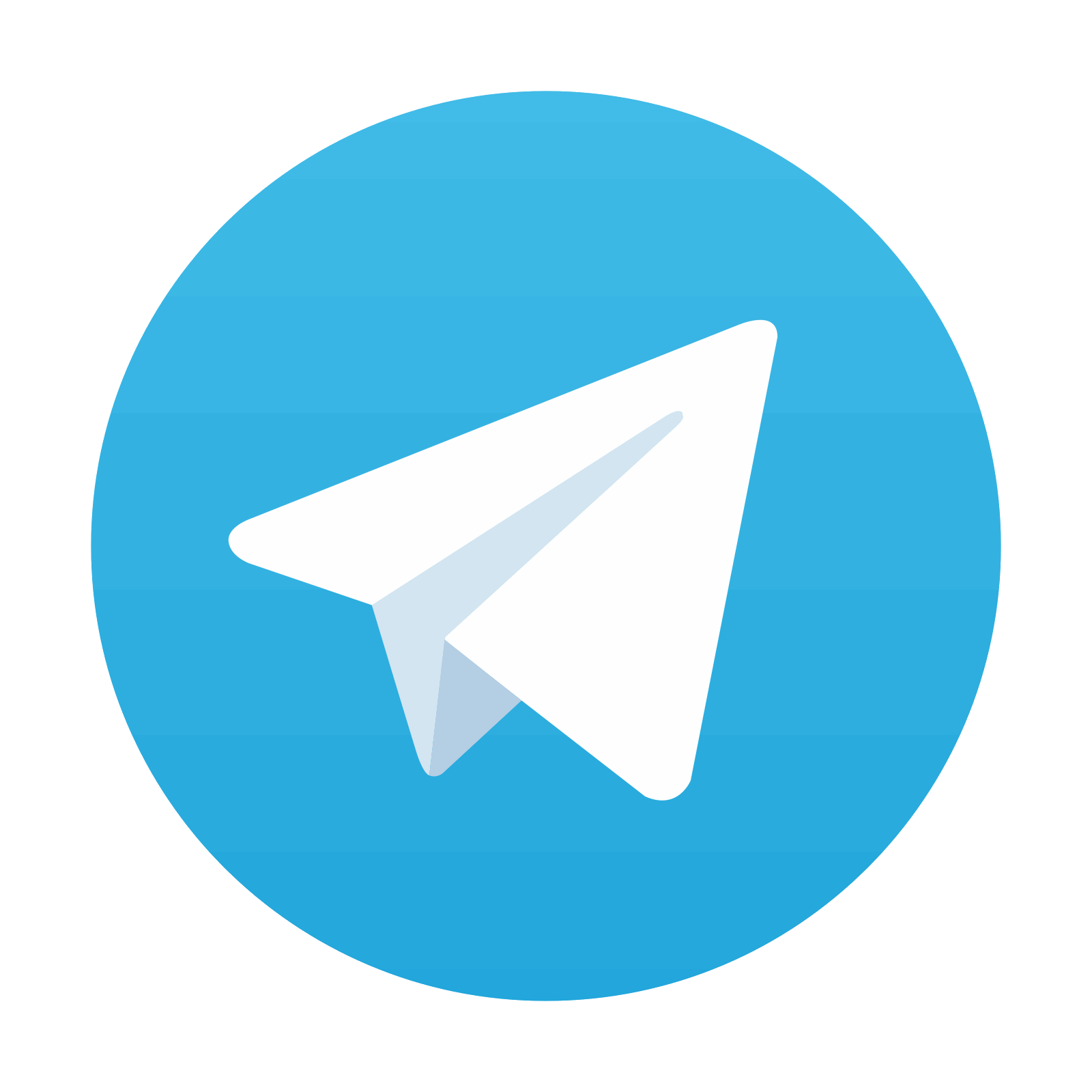
Stay updated, free articles. Join our Telegram channel

Full access? Get Clinical Tree
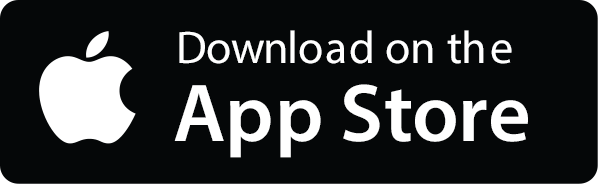
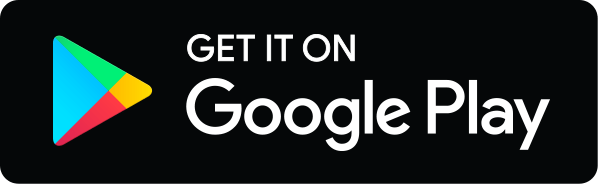