History
Frequent snoring (≥3 nights/week)
Labored breathing during sleep
Gasps/snoring noises/observed episodes of apnea
Sleep enuresis (especially secondary enuresis) after ≥6 months of continence
Sleeping in a seated position or with the neck hyperextended
Cyanosis
Headaches on awakening
Daytime sleepiness
Attention-deficit/hyperactivity disorder
Learning problems
Physical examination
Underweight or overweight
Tonsillar hypertrophy
Adenoidal facies
Micrognathia/retrognathia
High-arched palate
Failure to thrive
Hypertension
Several questionnaires have been developed to aid in the diagnosis of OSA, including the OSA-18 [34] and the Pediatric Sleep Questionnaire (PSQ) [35]. Compared with the gold standard polysomnography (PSG), these detailed questionnaires, often with more than 20 items, have a sensitivity of 78 % and a specificity of 72 % [36]. In a recent study of children with craniofacial disorders, 57 % had positive PSQ, but only 28 % had PSG findings of OSA [37].
It is evident that the accurate diagnosis and stratification of OSA require that clinical evaluation be supplemented with a test [38].
14.2.2 Diagnostics Test
Currently, the gold standard for the diagnosis of OSA is a nocturnal, in-laboratory PSG study [1]. The typical PSG monitoring includes electroencephalography (EEG), chin and anterior tibial electromyography (EMG), bilateral electrooculography, pulse oximetry and photo plethysmography, airflow sensor (nasal pressure transducer, oronasal airflow thermistor, end-tidal capnography), chest and abdominal respiratory inductance plethysmography, body position sensor, microphone, and real-time synchronized video monitoring. Key metrics obtained from PSG are listed in Table 14.2 [1]. Abnormalities of sleep are quantified with the apnea-hypopnea index (AHI), which computes events related to obstruction [39] and various metrics of saturation including the nadir saturation and desaturation index. Hypercarbia is a feature of severe OSA.
Respiratory event | Definition |
---|---|
Apnea | Complete cessation of flow (drop of peak signal excursion to ≤10 % of the baseline for more than 90 % of the event duration, for ≥10 s) |
Hypopnea (partial apnea) | Drop of signal by ≥30 % of the baseline for at least two breaths and associated with a ≥3 % oxygen desaturation by pulse oximetry or an arousal |
Respiratory effort-related arousals (RERAs) | Increasing respiratory effort, flattening of the nasal flow waveform, or an elevation in the end-tidal PCO2, lasting at least two breaths and associated to an arousal from sleep |
Hypoventilation | Period of ventilation ≥25 % of total sleep time characterized by a PCO2 >50 mmHg |
Apnea-hypopnea index (AHI) | The combined total number of apneas and hypopneas per hour of sleep |
Respiratory disturbance index (RDI) | The combined total number of apneas, hypopneas, and RERAs per hour of sleep |
Unlike adult OSA, there remains no consensus on the criteria to establish the diagnosis of OSA in children [35].
14.2.3 Cardiorespiratory Studies
Abbreviated cardiorespiratory studies may be performed at home or at the bedside. These studies limit the recordings to the measurement of oronasal airflow, respiratory inductance plethysmography, body position, snoring sounds, electrocardiography, transthoracic impedance, and pulse oximetry saturation. They correlate well with PSG [43].
14.2.4 Oximetry
The incidence of hypoxemia among children with SDB younger than 3 years is almost 40 %, decreasing by 17 % for each 1-year increase in age [34].
Overnight oximetry, which is potentially more widely available, can be used to evaluate OSA at the bedside and at home [44].
In a population of children referred to sleep laboratories with a high pretest probability of OSA, there is a very high probability (99 %) that an abnormal oximetry will be associated with PSG-proven OSA. However, when applied to the general population where the pretest probability of OSA is lower, the probability that an abnormal oximetry is associated with OSA is lower [45].
Desaturation indices (number of desaturation events >4 % below the baseline saturation) of 2.0, 3.5, and 4.2 correlate with mild, moderate, and severe OSA (AHI >1, AHI >5, and AHI >10, respectively). Each correlation showed high sensitivity (77.7, 83.8, and 89.1 %, respectively) and specificity (88.9, 86.5, and 86.0 %, respectively) [46].
An abnormal oximetry trend study has been defined as at least three clusters of desaturation. The McGill Oximetry Score (MOS) was developed to further stratify the severity of OSA. It identifies three levels of severity (MOS2, MOS3, and MOS4) defined as nadir saturations below 90, 85, and 80 %, respectively. The MOS has been shown to correlate with PSG [47, 48] and may be used as an initial test to evaluate children with SDB [49, 50]. Constantin et al. [34] compared the MOS to the OSA-18 questionnaire. An OSA-18 score of 60 (maximum 126) had very low sensitivity (40 %) but high negative predictive value. Therefore, children with a low OSA-18 score are unlikely to have OSA. This suggests that whereas a careful clinical evaluation may exclude severe OSA, it cannot reliably stratify OSA severity.
14.2.5 Other Studies
Nap studies have also been proposed but results are conflicting. The use of home PSG, respiratory polygraphy, nasal resistance, electrocardiogram-based automated apnea screening, and pulse transit time (PTT) [2, 51] is under investigation. Similarly, audio and videotaping have shown a sensitivity of 94 %, a specificity of 68 %, a positive predictive value of 83 %, and a negative predictive value of 88 %, but they are, like polysomnography, time-consuming to perform or analyze [52].
Radiologic studies (i.e., X-ray) have been also used, but the sensitivity, specificity, and positive and negative predictive values of these tests have yet to be reported [2].
14.2.6 Future Prospective
14.3 Anatomical and Physiological Basis of Respiratory Control
14.3.1 Upper Airway Anatomy and Its Relationship with OSA
Infants and children have a small maxilla and a large occiput, which may predispose to airway collapsibility. Within the first year of life, both the maxilla and the cranium will grow and promote the stability of pharyngeal architecture [55].
The upper airway is an “X”-shaped passageway, with the mouth and nose as two distinct entry points, which meet in the pharynx before splitting apart into the larynx and esophagus.
The upper airway contains different anatomical structures, with different growth characteristics. The following section gives an overview of the relative importance of each structure.
14.3.1.1 Nasopharynx
The nose receives support from boney structures and cartilage. Therefore abnormalities of these structures may promote obstruction of the nasal airway [56].
The nasal resistance to airflow spontaneously fluctuates in both adult and children. In adults, nasal obstruction increases the number of apneas and hypopneas [57] and worsens the sleep disturbance [58]. In children, nasal resistance is also affected by body position, increasing in the dependent nostril [59].
In infants, the nasal route of breathing is extremely important as they are preferential nasal breathers [60]. Indeed infants with unrecognized choanal atresia may asphyxiate.
14.3.1.2 Mouth and Pharynx
The oral cavity is limited by the hard and soft palate superiorly and the tongue inferiorly, and it is bounded laterally and anteriorly by the alveolar process. It opens posteriorly into the oropharynx and forms the part of the “X” where the nasal and oral cavities meet.
More inferiorly, the oropharynx continues into the retroglossal region (or “hypopharynx”), which is bounded anteriorly by the tongue and epiglottis, superiorly by the retropalatal oropharynx, inferiorly by the esophagus and larynx, and posteriorly and laterally by the pharyngeal constrictors.
During development, the tonsillar and adenoid tissues grow faster than the surrounding structures, encroaching on the airway and predisposing to pharyngeal obstruction [61].
In normal children, airway closure occurs at the level of soft palate and base of the tongue, whereas in children with SDB, pharyngeal closure occurs at the level of tonsils and adenoids [62].
Children with OSA have shown larger soft palate and bigger tonsils and adenoids, which narrow the retroglossal pharyngeal space [63]. Adenoid size is an important determinant in children between 1 and 12 years.
The tongue plays a critical role in the genesis of OSA. The loss of tongue and neck muscle tone causes the tongue to fall back into the posterior pharynx, often resulting in partial or complete obstruction [64], especially accentuated in mandibular hypoplasia. Furthermore, lingual tonsillar abnormalities can also predispose to airflow obstruction. This is especially important in the child with trisomy 21 [65].
14.3.1.3 Larynx
Below the epiglottis, the upper airway divides into the esophagus, located posteriorly, and the trachea, located anteriorly. Abnormalities of this area are rarely associated with OSA, unless anatomical or neurological abnormalities are present [66].
14.3.2 Neuronal Control of Breathing in Sleep and Airway Collapsibility
Two models have been proposed to describe the airway collapsibility [55]: the anatomical balance model and the neural balance model.
The anatomical balance model [69] considers the upper airway as a tube with a collapsible segment (analogous to the nose, the hypopharynx, and the larynx), surrounded by soft tissue (i.e., tongue) contained in a rigid box (i.e., boney structures). The pressure at which the pharyngeal airway collapses has been investigated. Two pressures have been reported: the critical pressure (Pcrit) and the closing pressure (Pclose). The Pcrit [70] was studied in awake spontaneously breathing children. In contrast, Pclose [62] was determined in paralyzed, anesthetized, apneic patients.
Pharyngeal collapse (Pcrit) occurs when the surrounding pressure is greater than the pressure within the airway segment. If the upstream resistance (nose) is low, as in normal subjects, the downstream (hypopharynx) pressure does not achieve Pcrit and airway patency is determined by the inspiratory pressure.
This model has been successfully applied in wake adults and children to describe the dynamic interaction of neuronal and anatomical components in the genesis of pharyngeal obstruction [70, 71].
In paralyzed and ventilated children, Isono [62] endoscopically evaluated the static changes of pharyngeal cross-sectional area at different pressures and identified the Pclose and the anatomical levels at which the airway collapsed.
In the neural balance model [72], airway patency is determined by the balance between pharyngeal collapsing forces (diaphragm, external intercostal muscles) and pharyngeal dilator muscles (i.e., the genioglossus muscle, which is the major upper airway dilator).
The pharyngeal dilator muscles are regulated by:
1.
Consciousness: both sleep and general anesthesia decrease the pharyngeal dilator muscle activity relatively more than the diaphragm and external intercostal muscles, promoting airway collapse.
2.
The negative pressure airway reflex.
3.
The level of chemical stimuli: pO2, pCO2, and pH.
During wakefulness and non-rapid eye movement (NREM) sleep, activation of the pharyngeal dilators during inspiration maintains a patent pharyngeal airway. Transition to sleep results in reduced tone of the pharyngeal dilator and constrictor muscles [73], with a subsequent increase in airway resistance.
The pharyngeal collapsing forces may overcome the dilating forces, creating a subatmospheric (negative) pressure in the pharyngeal airway. Pressure receptors located in the mucosa of the nasopharynx and larynx are stimulated in response to this subatmospheric pressure (negative pressure reflex) [73], activating the pharyngeal dilators to counteract the collapse of the airways [74].
If negative pressure reflex fails, an apnea event occurs, and the subsequent changes in pO2, pCO2, and pH may stimulate a ventilatory response and an arousal.
Younes introduced the concept of loop gain (LG), which describes the feedback interaction (loop) between an apnea event, the subsequent increase of pCO2, and the ventilatory response (gain), which then normalizes the pCO2 to maintain respiratory homeostasis [75]. If the initial compensatory response overshoots, the resulting hypocarbia will cause a second hypopnea/apnea, which will be more severe than the initial one, and the cycle can perpetuate indefinitely. In normal individuals, this control system is stable (LG is <1). In patients with OSA, the system is unstable (LG >1) [76].
In normal children, the risk of obstruction due to anatomic factors such as small airway size, high nasal resistance, and high chest wall compliance is compensated by an increased ventilatory drive and decreased airway collapsibility [77]. In children with OSA, the neuromotor response to airway collapse has been linked to respiratory afferent cortical processing deficits [78].
14.4 Special Considerations
14.4.1 Infants and Premature Infants
A detailed description of OSA in infants is beyond the scope of this chapter. Interested readers are referred to the excellent review of Katz et al. [79]. Whereas in children high nasal resistance, relative hypoplasia of the mandible and maxilla is an anatomical feature associated with OSA, in infants, laryngomalacia, choanal atresia, cleft palate, and subglottic stenosis are commonly reported [79]. Adenotonsillar hypertrophy may become significant beyond 6 months of age; it is more common among males and preterm or low birth weight infants, and it is associated with high recurrence rate after surgery [80].
In infants, the site of obstruction is palatal or retroglossal, and it increases during neck flexion [79]. In a recent retrospective study [81], premature infants represented the 5.5 % of all surgical patients with SDB. Pulmonary and gastrointestinal comorbidities and airway abnormalities were common (40, 29.8, and 19.3 %, respectively). One third of the patients had abnormal preoperative pulse oximetry.
Polysomnographic criteria for OSA in infants have yet to be established, but OSA should be suspected in any infant with an AHI >2. Furthermore, infants with laryngomalacia and chronic respiratory distress should be evaluated for SDB [79].
In the majority of infants, symptoms of OSA resolve during development [68]. Nonpharmacological management of OSA includes continuous positive airway pressure (CPAP), position therapy, and nasopharyngeal intubation. Surgical options include adenotonsillectomy, supraglottoplasty, lip-tongue adhesion, mandibular distraction, and tracheostomy [79].
14.4.2 The Obese Child
Several factors contribute to OSA in the obese child [82]. Tonsillar or adenoid hypertrophy is reported in 65 % [83]. The adipose tissue, infiltrating the pharyngeal muscles and the surrounding structures [84], may contribute to the airway collapsibility that characterizes OSA. Obesity compromises upper airway patency not only by encroaching on the caliber but also by impairing the function of upper airway muscles. Obesity also decreases the longitudinal tension of the airway and reduces lung compliance and functional residual capacity, all of which promote pharyngeal airway collapse [85].
Severe obesity and OSA are associated with sleep fragmentation and subsequent psychomotor impairment, reduced memory recall, and lower spelling scores [86].
In children, obesity carries higher risk of depression [26], suicide, relationship difficulties, gastroesophageal reflux [87], hepatic diseases, irritable bowel syndrome [88], metabolic syndrome (note that insulin resistance is present in 30–50 % vs. 4 % in nonobese adolescents) [89], type 2 diabetes mellitus, fatty liver disease [90], hypertension, dyslipidemia, and atherosclerosis [91].
Compared to OSA-matched subjects, obese children showed lower cardiac index volume, stroke volume index, and oxygen consumption at peak exercise capacity [24].
The risk for the metabolic syndrome (insulin resistance, dyslipidemia, hypertension, and obesity) increases with every 0.5-unit increment in BMI z score (odds ratio, 1.55; 95 % CI 1.16–2.08), and it is present in half of the severely obese children (BMI z score >2.5) [89].
A retrospective analysis [92] shows that overweight/obese (BMI >27 kg/m2) children undergoing adenotonsillectomy are more at risk of desaturation, laryngospasm, and perioperative upper airway obstruction. These children are also admitted more often and hospital stays are longer than healthy children.
14.4.3 Syndromes
Syndromes and medical conditions predisposing to OSA in children and adolescents are summarized in Table 14.3 [94].
Craniofacial syndromes associated with significant mandibular or maxillary hypoplasia | Apert syndrome Crouzon syndrome Goldenhar syndrome (hemifacial microsomia) Hallermann-Streiff syndrome Pierre Robin syndrome (Robin sequence) Rubinstein-Taybi syndrome Russell-Silver syndrome Treacher Collins syndrome |
Other syndromes featuring prominent craniofacial involvement | Achondroplasia Klippel-Feil syndrome Larsen syndrome Saethre-Chotzen syndrome Stickler syndrome Velocardiofacial syndrome |
Conditions associated with macroglossia | Beckwith-Wiedemann syndrome Down syndrome Hypothyroidism Mucopolysaccharide storage disorders (e.g., Hunter, Hurler syndromes) |
Conditions associated with anatomic airway obstruction | Adenotonsillar hypertrophy Cleft palate and/or cleft palate repair Choanal atresia or stenosis Fetal warfarin syndrome Laryngotracheomalacia Nasal polyps or septal deviation Pfeiffer syndrome Vascular ring |
Neurologic disorders associated with weakness or impaired ventilatory control | Cerebral palsy Cranial neuropathies (e.g., Mobius syndrome, poliomyelitis) Neuromuscular disorders (e.g., Duchenne, myotonic dystrophies) Structural brainstem lesions (e.g., Chiari malformations, syringobulbia) |
Conditions characterized by obesity | Morbid obesity/metabolic syndrome Prader-Willi syndrome |
Other conditions | Arthrogryposis multiplex congenita Conradi-Hünermann syndrome Gastroesophageal reflux Sickle cell disease |
If mouth breathing and obesity are combined with poor chin development and long face (adenoidal face), OSA should be suspected [95].
OSA symptoms are common in craniofacial malformations [96]. In a recent study, PSG (AHI >5) was positive in 28 % of children with craniofacial malformations [37]. More than 70 % of patients with syndromic craniosynostosis (Apert, Pfeiffer, and Crouzon) may have OSA (median AHI 12.9), which is severe in most of the cases [96].
These patients frequently require more complex and invasive airway surgery such as tongue reduction or lingular tonsillectomy [97].
Children with trisomy 21 are at high risk of having OSA, and the obstruction may be at several levels in the airway [98, 99]. It has been recently reported that 97 % of these children have abnormal polysomnography, and 59–66 % have moderate to severe OSA [100]. Compared to normally developed children, patients with trisomy 21 show higher MOS and higher EtCO2 at night [101]. At the time of the adenotonsillectomy, children with trisomy 21 may have already developed clinically significant pulmonary hypertension [102].
The Prader-Willi syndrome is a condition that combines anatomical alterations (dimorphisms, including micrognathia) with obesity. Adenotonsillectomy has been proposed to treat the OSA, but it is associated with a higher risk of perioperative complications including delayed emergence, hemorrhage, hypoglycemia, laryngospasm, supplemental oxygen administration, and the need for reintubation [103].
14.4.4 Central Hypoventilation Syndromes
Congenital central hypoventilation syndrome is a sleep-dependent hypoventilation disorder, which is classically diagnosed at birth but may present late in infancy or even in adulthood (late-onset central hypoventilation syndrome, LOCHS) [104]. It is characterized by an impaired response to hypercapnia and an overnight oxygen saturation trace that differs from the OSA pattern [104]. Mutations of gene PHOX2B have been identified in patients affected by LOCHS. Exposure to anesthesia may result in delayed emergence, which may be confused with opioid hypersensitivity or residual neuromuscular blockade [105]. Postoperative hypoventilation may be the presenting feature.
Rapid-onset obesity with hypothalamic dysfunction (ROHHAD) is another central hypoventilation disorder, which may present in the perioperative period [106]. However, its etiology, pathophysiology, and associated endocrine abnormalities differ from the LOCHS.
14.5 Medical Treatment of OSA
Several studies are investigating medical treatments for OSA in children [107].
Noninvasive positive pressure ventilation (NIV) is extensively used in adults. Increasingly NIV is used to treat infants and children with OSA [108].
Since the settings for NIV systems after surgery may differ from the ones used at home, care must be taken to provide trained staff and monitoring throughout the hospital admission and immediate access to invasive ventilation in the event of respiratory deterioration during the postoperative period [109].
The application of high-flow oxygen nasal cannula, weight loss, and positional therapy (lateral and prone positions versus supine during sleep) are promising therapies in selected OSA patients, but long-term results are inconclusive or unknown [97, 110].
Dental procedures, such as rapid maxillary expansions and oral appliances, may be indicated. Two detailed reviews have been recently published [97, 110].
Since inflammation has been shown to play a pivotal role in tonsil and adenoid hypertrophy [107], immunomodulating drugs such as montelukast may prove useful in the future. Nasal corticosteroids seem to be effective [107, 110] and may be of short-term benefit allowing optimization prior to adenotonsillectomy. Their long-term safety has still to be established [110]. Studies on drugs directed to different biomarkers are under investigation [107].
14.6 Surgical Treatments
The first-line treatment of OSA in children is adenotonsillectomy, which is the most common surgery performed in the United States (annual caseload 500,000).
Adenotonsillectomy has been shown to improve the AHI, quality of life (QOL), behavior, and school performance, regardless of the presence of obesity [83]. Adenoidectomy alone has been shown to reduce airway collapsibility [70].
A recent systematic review analyzed the cardiovascular benefits of adenotonsillectomy [111]. Despite the high heterogeneity of the studies, adenotonsillectomy seemed to have beneficial effects on blood pressure (especially diastolic) and cardiac function [111].
Similarly, a recent study reported a decrease of mean pulmonary pressure, right and left ventricular isovolumetric contraction time length, myocardial performance index values, and an increase of ejection time [30].
Surgical alternatives to adenotonsillectomy have been proposed. In children older than 5 years, partial tonsillectomy has been shown to decrease pain, improve the return to a normal diet, decrease the risk of bleeding, and maintain immunologic function, with minimal risk of regrowth [112].
In fact, residual OSA may occur in 20–40 % [93] of children with severe OSA, depending on AHI threshold criterion to define severe OSA [113]. Obesity, age >7 years, the presence of asthma, a high presurgical AHI [93] and respiratory disturbance index (RDI) [42], AA ethnicity [114], and craniofacial malformation [96] are risk factors for persistent OSA after surgery.
Adenotonsillectomy, however, is not without risks [107, 115, 116]. Hemorrhage, cardiorespiratory events, and medication error are the most common complications (54, 18, and 17 %, respectively) after adenotonsillectomy and are responsible for half of the mortality events [117, 118]. In particular, children may demonstrate a delayed onset of respiratory compromise several hours after surgery [42].
In 3–16-year-old children with mild OSA (AHI between 1 and 5), adenotonsillectomy has been shown to give early relief of symptoms and improvement of QOL compared to conservative clinical observation [119]. However, watchful waiting also showed significant improvements in QOL after 8 months and may be considered as an alternative to adenotonsillectomy.
In a recent retrospective analysis of 126 infants with OSA [120], 86 children underwent a variety of surgical interventions including tonsillectomy, adenoidectomy, and adenotonsillectomy in 35 % of the patients. Comparing these children with those managed with nonsurgical interventions (watchful waiting, antireflux therapy, CPAP), surgical interventions showed a similar success rate at 6 months’ follow-up.
A recent large multicenter, single-blind, randomized study, the Childhood Adenotonsillectomy Trial (CHAT) [114], investigated the outcomes of children between 5 and 9 years of age undergoing adenotonsillectomy versus a strategy of watchful waiting. As compared to control, adenotonsillectomy did not improve neurological outcomes (NEPSY attention and executive-function test) at 7 months’ follow-up, but children who underwent early adenotonsillectomy had greater behavioral improvement (caregiver and teacher ratings), quality of life (Pediatric Sleep Questionnaire and Pediatric Quality of Life Inventory), and PSG indexes (AHI, change from baseline –1.6 vs. –3.5, p < 0.001). Most importantly, PSG findings normalized in 79 % of children in early-adenotonsillectomy group but also in 46 % of watchful waiting group. Of note, 59 % of the obese children (BMI z score >1.65) still manifested severe OSA after surgery (AHI >5).
In children with syndromic craniosynostosis, adenotonsillectomy has been performed to alleviate the symptoms of obstruction, but additional interventions, such as CPAP therapy, mandibular distraction, and tracheostomy, may be required [96].
A recent study [121] compared CPAP with surgical therapy in OSA patients with trisomy 21 and mucopolysaccharidoses. It showed a similar 1-year outcome in terms of PSG, clinical evaluation, Epworth Sleepiness Scale—Children, QOL, and OSA-18 score.
Tongue base suspension and uvulopalatopharyngoplasty, associated or not with adenotonsillectomy, have been applied to selected patients and they may be beneficial. Recently, the European Respiratory Society Task Force has reviewed their clinical application [97]. Finally, tracheotomy is reserved for severe refractory patients [40].
14.7 Anesthesia Management of the Patient with OSA
14.7.1 General Recommendations: The American Society of Anesthesiology Guidelines
The American Society of Anesthesiologists (ASA) recently updated the guidelines for the management of patients with OSA [122]. It must be noted that these guidelines:
1.
Do not address patients with central apnea, airways abnormalities (i.e., deviated nasal septum) or obesity not associated with obstructive apnea, and hypersomnolence not secondary to sleep apnea.
2.
Do not consider children younger than 1 year.
3.
Encourage evaluation of OSA with sleep study. In its absence, the ASA endorses clinical criteria to both diagnose and stratify OSA severity.
4.
Recommend that OSA patient to be managed in hospitals that have the facilities to treat postoperative airway complications.
5.
Encourage the use of CPAP perioperatively.
6.
Recommend a full reversal of neuromuscular blockade.
7.
Highlight that OSA patients are at higher risk of respiratory depression secondary to opioids and sedatives.
8.
Recommend an accurate assessment of patient in the postoperative period.
9.
Encourage a prolonged period of observation. Patients should not be discharged to an unmonitored setting until they are no longer at risk of postoperative respiratory depression. This new recommendation has been introduced in 2014 and highlights the critical role of postoperative monitoring in reducing the risk of respiratory complications.
14.7.2 Anesthesia Management Options
The anesthesiologist should plan the anesthesia based on the severity of the OSA. As mentioned, clinical evaluation with preoperative questionnaires has high negative predictive value but a very low sensitivity [34]. Tait et al. [123] developed a limited five-item questionnaire to identify children with SDB at increased risk for perioperative adverse respiratory events. The study, however, was not designed to stratify the OSA severity, and the respiratory events were restricted to the immediate postoperative period.
Therefore, if sleep study data are not available, the anesthesiologist must assess airway collapsibility clinically. The risk and benefits of premedication should be also carefully weighed in children with severe OSA, since significant airway obstruction and severe oxygen desaturation can occur [124].
The child’s response to anesthesia may be informative as it may unmask OSA by inducing skeletal hypotonia simulating conditions during REM sleep. During spontaneous ventilation, the requirement for positive pressure to maintain pharyngeal airway patency, a high apneic threshold for carbon dioxide [125], and a heightened opioid sensitivity are all suggestive of severe OSA and may warrant postoperative admission and extended period of cardiorespiratory monitoring [126].
The use of local anesthesia may also unmask OSA. In children with OSA, the application of local anesthesia results in a relatively greater decrease of pharyngeal cross-sectional area likely from the inhibition of the upper airway dilators [127]. Titration of opioids to the severity of OSA is critical to reduce respiratory complications (see below).
Finally, supplemental oxygen must always be administered carefully in the recovery room as in children with severe OSA, their blunted carbon dioxide responsiveness may render them more dependent on peripheral respiratory drive [125].
14.8 Specific Guidelines for Anesthesia Management of the Children Undergoing Adenotonsillectomy
14.8.1 Recommendations for Adenotonsillectomy: Italian National Program Guidelines, American Academy of Otolaryngology—Head and Neck Surgery Guidelines, and American Association of Pediatrics Guidelines
The 2008 Italian National Program Guidelines [128] state that the decision to perform adenotonsillectomy should be based on clinical evaluation (i.e., loud snoring, low hemoglobin saturation) with or without the use of specific questionnaires. Nocturnal pulse oximetry is recommended as an initial test in children with suspected SDB. PSG is reserved for children with inconclusive pulse oximetry studies [48]. Adenoidectomy alone is not recommended due to the high recurrence of symptoms. Intraoperative morphine is not indicated. Multimodal analgesia with dexamethasone 0.5–1 mg/kg i.v. (max 8 mg), acetaminophen 15 mg/kg p.o. q4 h around the clock, and codeine 1 mg/kg p.o. q4 h pro re nata are recommended. However, it must be noted that codeine received a black box warning from FDA in 2013 [39].
Short-term antibiotics are also indicated. The guidelines also recommend postoperative admission for children younger than 3 years of age.
The American Academy of Otolaryngology—Head and Neck Surgery recommends PSG before adenotonsillectomy be reserved for children with coexisting diseases, such as obesity, trisomy 21, craniofacial abnormalities, neuromuscular disorders, sickle cell disease, and mucopolysaccharidoses [129]. Importantly, the results of PSG should be communicated to the anesthesiologist prior to the induction of anesthesia. Postoperative admission is recommended for patients younger than 3 years of age and with severe OSA (AHI ≥10 or more obstructive events/h, oxygen saturation nadir <80 %, or both).
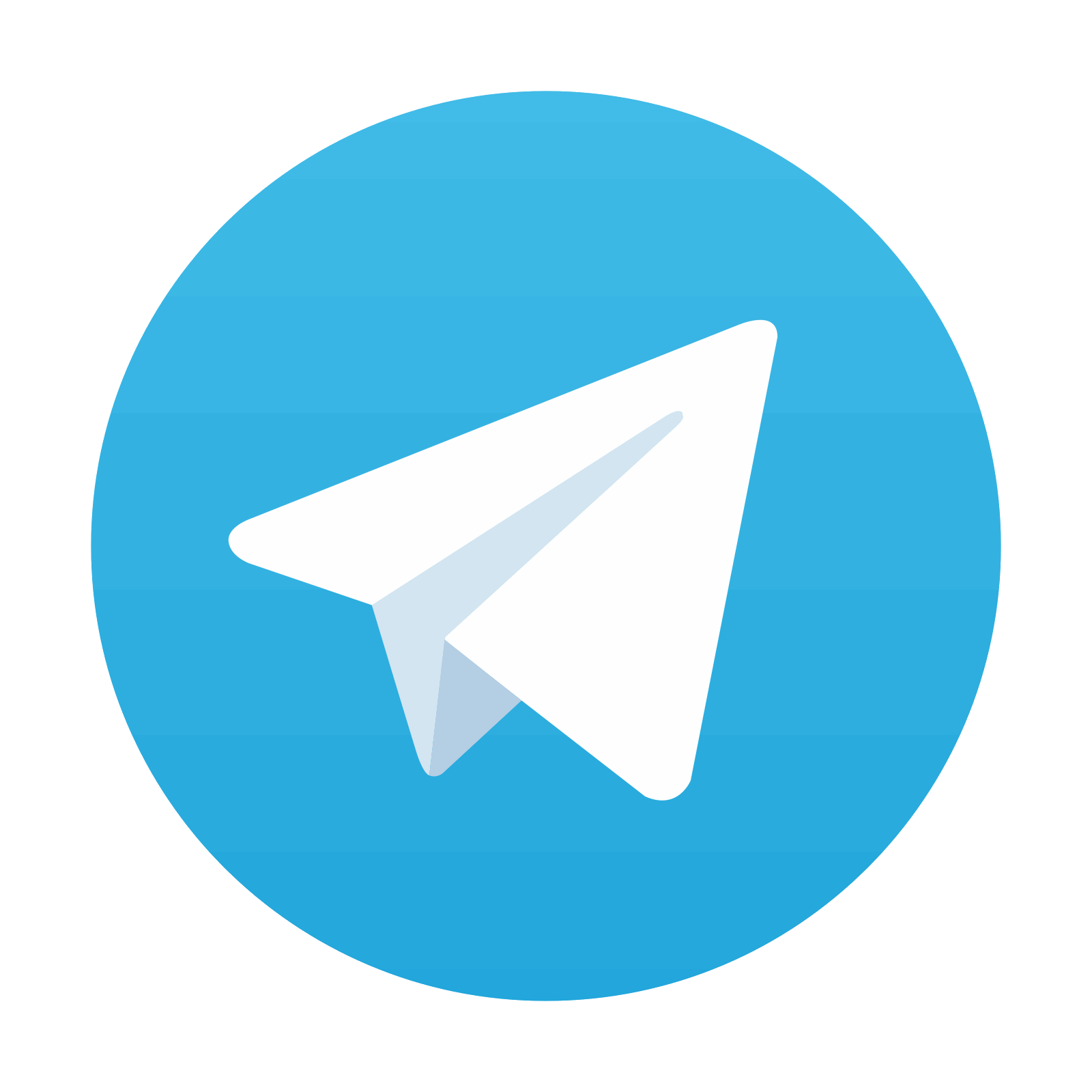
Stay updated, free articles. Join our Telegram channel

Full access? Get Clinical Tree
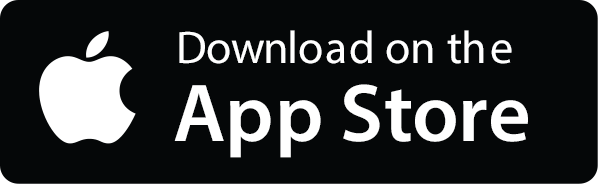
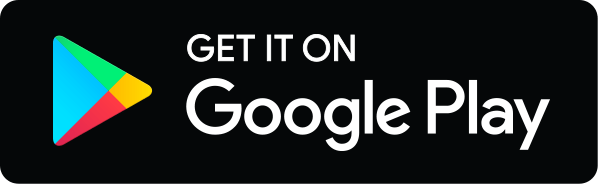