Type of cancer
Age (years)
Overall
0–4
5–9
10–14
15–19
0–19
Leukemias
36.1
33.4
21.8
12.4
25.2
CNS tumors
16.6
27.7
19.6
9.5
16.7
Lymphomas
3.9
12.9
20.6
25.1
15.5
Carcinomas and epithelial tumors
0.9
2.5
8.9
20.9
9.2
Soft tissue sarcomas
5.6
7.5
9.1
8.0
7.4
Germ cell, trophoblastic, and other gonadal tumors
3.3
2.0
5.3
13.9
7.0
Malignant bone tumors
0.6
4.6
11.3
7.7
5.6
Sympathetic nervous system tumors
14.3
2.7
1.2
0.5
5.4
Renal tumors
9.7
5.4
1.1
0.6
4.4
Retinoblastoma
6.3
0.5
0.1
0.0
2.1
Hepatic tumors
2.2
0.4
0.6
0.6
1.1
Miscellaneous neoplasmsa
0.5
0.3
0.6
0.8
0.6
16.2 Immunomodulatory Effects
Immunosuppression and cancer cell augmentation have been associated with certain anesthetic agents such as ketamine, inhalant anesthetics, and opioids. Propofol and nitrous oxide have been shown to confer less immunosuppression. Regional anesthesia may actually be beneficial in reducing cancer recurrence [5, 6]. Without stronger evidence, at the current time, we cannot recommend a specific anesthetic plan that avoids suppressing the immune system and augmenting cancer.
16.2.1 Toxicity of Oncological Therapies
Chemotherapy, radiation therapy, hematopoietic stem cell transplantation (HSCT), and surgical interventions are mainstays of cancer treatment. All of these modalities can significantly affect healthy tissue and lead to multiorgan dysfunction. The toxicities of pharmacologic agents must be understood and recognized by the anesthesiologist in order to optimally manage these children.
16.2.1.1 Chemotherapy
Chemotherapy is one of the cornerstones of cancer treatment. The majority of children with cancer who present for anesthesia are or have been submitted to one or more chemotherapy regimens. These medications can have significant biological effects on a range of organ systems as well as enzyme function. Therefore, the anesthetic must be optimized to address the altered physiology and medication interactions for each child. The greatest concerns pertain to the acute effects of chemotherapy, although occasionally the effects may persist and alter the child’s susceptibility to anesthesia years after conclusion of treatment. The effect of chemotherapy on perioperative care is determined by the specific agent, its inherent toxicity, and the cumulative dose. The impact of these agents on cardiorespiratory, hematopoietic, and gastrointestinal systems is most relevant to anesthesia. Table 16.2 provides an overview of chemotherapy agents and their association with organ toxicities.
Table 16.2
Common complications associated with chemotherapeutic agents
System toxicity |
Chemotherapeutic agents |
---|---|
Cardiac toxicity |
Busulfan, cisplatin, cyclophosphamide, daunorubicin/adriamycin, 5-fluorouracil |
Pulmonary toxicity |
Methotrexate, bleomycin, busulfan, cyclophosphamide, cytarabine, carmustine |
Renal toxicity |
Methotrexate, l-asparaginase, carboplatin, ifosfamide, mitomycin-C |
Hepatotoxicity |
Actinomycin D, methotrexate, androgens, l-asparaginase, busulfan, cisplatin, azathioprine |
CNS toxicity |
Methotrexate, cisplatin, interferon, hydroxyurea, procarbazine, vincristine |
SIADH secretion |
Cyclophosphamide, vincristine |
Cardiotoxicity
Cardiotoxicity can manifest as acute or subacute, chronic, and late-onset pathology. The most common chemotherapy agents that cause cardiotoxicity are anthracyclines (doxorubicin, daunorubicin, and epirubicin), mitoxantrone, cyclophosphamide, bleomycin, 5-fluorouracil, and paclitaxel [7–9].
Anthracyclines and the anthracycline analog, mitoxantrone, impair myocardial contractility that may lead to cardiomyopathy and congestive heart failure, especially if mitoxantrone total does exceeds 140 mg/m2 [10]. Anthracycline therapy enhances the cardiodepressive effects of anesthesia even in children who have normal resting cardiac function [11]. Dysrhythmias, which develop independent of the dose administered, may be manifested as sinus tachycardia, supraventricular tachycardia, junctional tachycardia, atrioventricular and bundle-branch block, or ventricular tachycardia. Dysrhythmias may occur as early as few hours after the administration of these agents [12, 13]. Forty percent of anthracycline-induced acute toxicity presents as electrocardiographic changes, which include the aforementioned dysrhythmias as well as decreased QRS voltage, and prolongation of the QT interval, which is characteristic of doxorubicin [7, 13]. With the exception of decreased QRS voltage, these changes usually resolve within 1–2 months of the conclusion of therapy. However, sudden death secondary to ventricular fibrillation may occur. Rare cases of subacute cardiotoxicity resulting in acute failure of the left ventricle, pericarditis, or a fatal pericarditis-myocarditis syndrome have been reported particularly in children [14].
Chronic anthracycline cardiotoxicity usually presents as cardiomyopathy. This sequela depends on the cumulative dose administered. The average reported incidence of congestive heart failure is 0.14 % with doses <400 mg/m2, 7 % with 550 mg/m2, 15 % with 600 mg/m2, and 35 % with 700 mg/m2 total dose of adriamycin [15, 16].
It is important to understand that anthracycline cardiotoxicity can have a late onset. A recent review reported occult ventricular dysfunction, heart failure, and arrhythmias appearing in previously asymptomatic patients more than 1 year after conclusion of anthracycline therapy [17]. Doxorubicin, in particular, can cause subclinical myocardial injury during preadolescent years, which later, during the period of growth spurt, impairs growth of the myocardium. In addition to the total dose of anthracyclines, high-dose radiation to the mediastinum, concurrent cyclophosphamide therapy, extremes of age, prior myocardial injury, hypertension, valvular heart disease, and liver diseases play a significant role in the development of cardiotoxicity.
Cyclophosphamide is the second agent that can cause myocardial injury. A total dose of cyclophosphamide in excess of 120 mg/kg administered over 2 days can cause severe congestive heart failure, hemorrhagic myocarditis, pericarditis, and necrosis.
Conventional daily oral doses of busulfan may cause endocardial fibrosis that manifests as constrictive cardiomyopathy [18, 19]. In case of preexisting cardiac disease, the use of interferon may exacerbate the disease. Mitomycin may also cause myocardial damage if administered over an extended period of time or in large doses [20]. Paclitaxelin combined with cisplatin has been reported to cause ventricular tachycardia [21].
If any of the aforementioned agents have been used, the child who presents for anesthesia care should undergo a thorough cardiac evaluation that may require echocardiography or nuclear medicine studies. Diastolic dysfunction may present as an early manifestation of anthracycline toxicity. If the left ventricular ejection fraction (LVEF) decreases to less than 45 %, it is considered indicative of anthracycline-induced cardiotoxicity.
The choice of intraoperative monitors should be based on the preoperative symptoms and the specific surgical procedure. Invasive arterial blood pressure monitoring and less commonly central venous catheter or transesophageal echocardiography may be helpful in children with documented cardiac dysfunction. Children who have received anthracycline therapy can develop acute intraoperative left ventricular failure refractory to beta-adrenergic receptor agonists under anesthesia. Amrinone and sulmazole are effective treatments in such cases [22]. Effects of non-anthracycline chemotherapeutic agents on the heart are summarized in Table 16.3.
Table 16.3
Adverse cardiac effects with non-anthracycline chemotherapeutic agents
Agent |
Cardiovascular effect |
---|---|
Cyclophosphamide |
Fulminant CHF secondary to hemorrhagic myocarditis |
Acute pericarditis with effusion | |
Risk increased with dose > 200 mg/m2 and anthracycline combination | |
Bleomycin |
Acute pericarditis |
5-Fluorouracil |
Coronary insufficiency presenting as angina/myocardial infarct due to coronary spasm |
Paclitaxel and docetaxel |
Asymptomatic bradycardia, severe bradyarrhythmias and tachyarrhythmias including ventricular fibrillation and asystole, conduction disorders, myocardial ischemia and infarction; Overall risk is increased with concomitant cisplatin therapy. Peripheral edema is due to fluid retention (docetaxel) |
Pulmonary Toxicity
Children who have cancer often present with pulmonary complications that may be the result of the disease itself or its treatment. Respiratory failure arises secondary to pulmonary metastases or primary lesions, infections, chemotherapy, radiotherapy, and/or extensive surgical pulmonary resections [23]. The mortality rate in these children is as great as 75 % if mechanical ventilation is required [24–26].
The primary chemotherapeutic agents that damage the pulmonary system are busulfan, cyclophosphamide, paclitaxel, and bleomycin, the last being the most detrimental of these.
Bleomycin is known to cause six distinct pathologies:
1.
Interstitial pneumonitis that can progress to chronic fibrosis
2.
Acute hypersensitivity pneumonitis
3.
Acute chest pain syndrome
4.
Bronchitis obliterans with organizing pneumonia
5.
Pulmonary venoocclusive disease
6.
Noncardiogenic pulmonary edema
Bleomycin-induced pulmonary toxicity develops 4–10 weeks after therapy with an incidence between 0 and 40 %. Nonlethal pulmonary fibrosis is known to occur in 11–30 % of children with an associated mortality from bleomycin of 2–10 %. As many as 20 % of children with radiological and histological evidence of bleomycin toxicity are symptom-free [27]. Risk factors for bleomycin pulmonary toxicity are old age, cumulative dose >400–450 mg, poor pulmonary reserves, radiotherapy, uremia, greater inspired oxygen concentrations, and concomitantly administered other anticancer drugs.
Bleomycin is believed to cause direct cytotoxicity that involves the production of superoxides and other free radicals. The use of greater concentrations of inspired oxygen augments this process. Although pulmonary injury is thought to develop with cumulative dose >400–450 mg, fetal pulmonary fibrosis has been reported with total doses as low as 50 mg [22]. The fetal injury is believed to occur as type II pneumocytes replace type I pneumocyte in the alveoli. With continuous exposure, type II pneumocytes are replaced by cuboidal epithelium. Further, since effective tissue repair is absent, this leads to the development of fibrosis. The chest X-ray shows bilateral basal and perihilar infiltrates with fibrosis. The clinical picture includes fever, cough, dyspnea, bibasilar rhonchi, and rales. Exertional dyspnea may occur with mild X-ray changes and a normal or low resting PaO2. Arterial hypoxemia is a common sign of bleomycin toxicity. An increased alveolar-arterial PO2 gradient and decreased carbon monoxide diffusing capacity (DLCO) are consistent findings in interstitial fibrosis. Pulmonary function testing indicates a restrictive lung pattern. Regression of the injury may occur with immediate cessation of therapy. Steroid therapy has been found to be effective in some cases.
The perioperative inspired oxygen concentration has been a concern in those who received bleomycin therapy. In one report of patients who were treated with bleomycin and who received an intraoperative FiO2 > 0.39, five developed ARDS and subsequently died [28]. In two more recent, larger series, no perioperative pulmonary complications occurred in patients who received bleomycin and who were subsequently exposed to intraoperative FiO2 > 0.25 and >0.41 [29, 30]. A recent, much larger study demonstrated minor pulmonary complications in patients exposed to inspired FiO2 > 0.40 and >0.25, although none progressed to ARDS or died. Instead, meticulous fluid balance, duration of surgery, and postchemotherapy forced vital capacity were recognized as significant predictors of postoperative pulmonary morbidity [31]. In the face of this conflicting evidence, the current recommendations are to minimize the FiO2 wherever possible to maintain the SpO2 in excess of 90 % and to use intraoperative PEEP.
During preoperative assessment of patients with pulmonary complications chest X-ray, pulmonary function tests, arterial blood gas analysis, and in some instances DLCO may assist in identifying the severity of the pulmonary dysfunction. In some cases, arterial access may facilitate repetitive intraoperative arterial blood gas analyses. Depending on the preoperative presentation and intraoperative course, postoperative ventilatory support may be anticipated.
Other chemotherapy agents and their adverse pulmonary effects important to anesthesia are listed in Table 16.4.
Table 16.4
Adverse pulmonary effects with non-bleomycin chemotherapy agents
Agent |
Incidence (%) |
Description |
---|---|---|
Busulfan |
4–10 |
Pulmonary fibrosis, pulmonary alveolar lipoproteinosis |
Cyclophosphamide |
<2 |
Pneumonitis with or without fibrosis |
Mitomycin |
<10 |
Similar to bleomycin |
Cytosine arabinoside |
5–32 |
Noncardiogenic pulmonary edema with or without pleural effusion |
Methotrexate |
7 |
Hypersensitivity pneumonitis or |
Noncardiogenic pulmonary edema or | ||
Pulmonary fibrosis or | ||
Pleurisy with acute chest pain |
Hepatorenal Toxicity
The renal system is affected by several chemotherapy agents, the most common and important of which is cisplatin. Approximately 30 % of patients who are treated with cisplatin develop nephrotoxicity, which is the dose-limiting consequence. The most important measure to prevent nephrotoxicity is adequate hydration with forced diuresis. Normal saline is a particularly beneficial fluid to infuse as the large concentration of chloride in the tubules inhibits the hydrolysis of cisplatin [22]. Renal injury stems from coagulation necrosis of proximal and distal renal tubular and collecting duct epithelium that reduces the renal blood flow and glomerular filtration rate (GFR). A single dose of cisplatin of 2 mg/kg or 50–75 mg/m2 produces nephrotoxicity in 25–30 % of patients [32]. Acute renal failure can occur within 24 h of administration. Chronic decreases in GFR can also occur. The reported decrease in GFR after 16–52 months of cisplatin therapy is 12.5 % [33]. Coadministration of other nephrotoxic drugs, such as aminoglycosides, increases the risk of nephrotoxicity. Newer cisplatin analogs, i.e., carboplatin and oxaliplatin, are less nephrotoxic than cisplatin. Other nephrotoxic agents are listed in Table 16.5.
Table 16.5
Adverse nephrotoxic effects with non-cisplatinum chemotherapeutic agents
Agent |
Description |
---|---|
Mitomycin |
Chronic progressive increase in serum creatinine to microangiopathic hemolytic anemia |
Methotrexate |
Physical effect because of precipitation of drug within the renal tubules |
NSAIDs reduce excretion of methotrexate and therefore should be avoided [34] | |
Ifosfamide |
Acute tubular necrosis and renal failure |
Hepatic injury presents as increased liver enzymes, impaired synthetic function with low protein and coagulation factor synthesis, fatty infiltration of liver, and cholestasis. The cause of the hepatic injury may be due to either the chemotherapy itself or their metabolites. Among these, l-asparaginase and cytarabine are the most common chemotherapeutic agents that induce hepatocellular dysfunction, although cyclophosphamide and mitomycin have been reported to cause dysfunction as well. The resultant hepatic dysfunction can range from mild to very severe, with associated ascites, painful hepatomegaly, and encephalopathy.
Neurotoxicity
Chemotherapy can affect central, peripheral, and autonomic nervous systems. Vincristine is the only known chemotherapeutic agent whose dose is limited by the presence of neurotoxicity. It can affect all aspects of the nervous system. Central nervous system toxicity may be manifested as ophthalmoplegia and facial palsy. Peripheral neuropathy is characterized by depression of deep tendon reflexes and peripheral paresthesia that progresses proximally over the course of chemotherapy. Motor dysfunction and gait disorders can also occur. Autonomic dysfunction may result in orthostatic hypotension, erectile dysfunction, constipation, difficulty in micturition, bladder atony, and more.
About 50 % of those who receive cisplatin treatment develop neurotoxicity that depends on the dose administered and the duration of therapy. It usually presents as peripheral neuropathy in stocking and glove distribution and auditory and visual impairment. A subclinical, unrecognized neuropathy may cause unexpected complications from regional anesthesia. Recently, a diffuse brachial plexopathy was reported after an interscalene block in a patient receiving cisplatin [35]. Therefore, if regional anesthesia is planned, a thorough neurological examination is warranted.
Severe neurologic consequences have occurred after inadvertent intrathecal administration of vincristine. Close to 100 fatal or serious irreversible neurologic injuries have been reported, although the true number of cases is unknown [36].1 Vincristine is an effective intravenous chemotherapeutic agent for leukemia, lymphoma, Wilms’ tumor, neuroblastoma, and other tumors. However when injected intrathecally, it produces either a local motor neuropraxia (resulting in paresis of varying severity) or ascending radiculomyeloencephalopathy, coma, and death. Typically, vincristine is prepared in a syringe for intravenous administration, but when a patient is scheduled for intrathecal chemotherapy, it has been inadvertently given along with or in place of the prescribed intrathecal chemotherapy with disastrous sequelae. Although there is no strategy to prevent the neurologic injury or death once intrathecal vincristine has been given, if the error is identified immediately, then the CSF has been irrigated and flushed with fresh frozen plasma, resulting in para- or tetraparesis and only a 15 % mortality [36]. This contrasts with a 100 % mortality without aggressive treatment with plasma. Since 50 % of vincristine is bound to plasma proteins within 20 min of injection into blood, circulating plasma in the CSF is believed to bind with vincristine, preventing it from destroying the neuronal cytoskeleton and inducing neurologic injury or death. The key strategy to avoid this potentially catastrophic outcome is to never prepare vincristine in a syringe and to always keep vincristine remote from any intrathecal procedures.
Additional neurotoxic chemotherapy agents are listed in Table 16.6.
Table 16.6
Adverse neurotoxic effects with non-vincristine chemotherapy agents
Agent |
Incidence (%) |
Description |
---|---|---|
Cytarabine |
15–37 |
Cerebellar dysfunction, peripheral neuropathy, seizures, encephalopathy, myelopathy, pseudobulbar palsy |
Ifosfamide |
0–10 |
Cerebellar dysfunction, hemiparesis, coma, extrapyramidal abnormalities |
5-Fluorouracil |
0–5 |
Cerebellar dysfunction, multifocal leukoencephalopathy |
Methotrexate |
0–2 |
Meningeal irritation, transient paraparesis, encephalopathy |
Paclitaxel |
50–70 (high dose) |
Peripheral neuropathy, autonomic neuropathy |
Procarbazine |
Cerebral effects: lethargy, depression to psychosis, peripheral neuropathy |
Hematological Effects
All lines of hematopoiesis can be affected either by the primary malignancy, metastasis to bone marrow, or chemotherapy. A dysfunctional coagulation cascade can prolonged PT and PTT times; increase factor I, V, VIII, IX, XI, and fibrinogen degradation products; reduce platelet life span; and decrease antithrombin III activity. To date, no prospective trials have estimated the minimum platelet count required to prevent bleeding during specific procedures. The empiric recommendation is to maintain the platelet count in excess of 50,000 per microliter during the perioperative period. It is essential to correct any preexisting coagulopathy before commencing surgery.
Anemia is very common in cancer patients. Recommendations differ regarding the transfusion threshold for packed red blood cell (PRBC). Most recommend a hemoglobin threshold of <9 g/dl for transfusion in asymptomatic children, with greater values in symptomatic patients [37]. During perioperative period, the anesthesiologist should closely follow the blood loss and guide the decision to transfuse based on age-adjusted estimated allowable blood loss calculations. Immunocompromised children including infants depending on the institutional policy; hematopoietic progenitor cell transplant recipients; those with Hodgkin’s disease, leukemia and lymphoma, aplastic anemia with severe lymphocytopenia, and solid organ tumors; those receiving nucleoside analog therapy (i.e., fludarabine); those requiring granulocyte transfusions; and those undergoing intense chemotherapy require irradiated PRBCs. Accordingly, red blood cell transfusions should be planned before surgery in collaboration with the treating oncologist [38].
Most children receiving broad-based chemotherapy will experience immunosuppression. Chemotherapy, by design, targets rapidly dividing cells, including immune system cells. For this reason, these children are more susceptible to infections. Meticulous aseptic techniques are mandatory during all invasive procedures. Wound healing can also be affected by the absence of neutrophils. Nonetheless, children whose WBC exceeds 500/mm3 generally have uncomplicated surgical wound healing. The effect of chronic anemia on surgical wound healing is minimal [39]. Other factors that complicate wound healing include chemotherapy. The effect of chemotherapy depends on the dose and timing of chemotherapy administration, as well as concurrent radiotherapy.
Myelosuppression caused by chemotherapy is transient and usually resolves within 1–6 weeks after cessation of treatment.
Hormone and Enzyme Production
The most common hormonal disturbance in children treated for cancer is steroid-induced adrenal suppression. The stress response may be blunted for 6 months or greater after therapeutic exogenous corticosteroids. Most children who continue their usual doses of corticosteroids during surgery have appropriate corticosteroid levels and can mount an endogenous stress response [40]. Whether they require a supplemental dose of steroids in the perioperative period remains controversial [41, 42]. The corticotrophin (ACTH) stimulation test is the definitive test to confirm adrenal suppression, but individual testing is labor-intensive, costly, and of limited predictive value. A recent review (with limited power) determined that patients with intact hypothalamic-pituitary axis who are receiving physiologic doses of exogenous steroids more than likely can mount a stress response, whereas those with dysfunction of the hypothalamic-pituitary axis (e.g., Addison’s disease) likely cannot mount an endogenous response to steroids and require supplementation [43]. Current practice in most centers favors supplementing children who have taken exogenous steroids in the perioperative period. If a child develops unexplained intraoperative or postoperative hypotension, particularly if it is unresponsive to a fluid bolus and/or pressors, treatment with hydrocortisone (1.5 mg/kg intravenously) is definitely warranted.
Syndrome of inappropriate antidiuretic hormone secretion (SIADH) is best known in association with paraneoplastic syndrome of small cell lung cancer, which is exceedingly rare in children, but it has been associated with other oncologic diseases as well. About 1–2 % of cancer patients develop SIADH. Some drugs, including vasopressin, carbamazepine, oxytocin, vincristine, vinblastine, cyclophosphamide, phenothiazines, tricyclic antidepressant agents, opioids, and monoamine oxidase inhibitors, can also induce SIADH [44]. The diagnostic criteria for SIADH include a serum sodium level <135 mEq/L, plasma osmolality <280 mOsm/kg, urine osmolality >500 mOsm/kg, and urine sodium >20 mEq/L. Patients who develop SIADH can experience malaise, altered mental status, seizures, coma, and occasionally death. Focal neurologic findings can occur in the absence of brain metastases. Acute treatment is indicated if serum sodium concentration is <125 mEq/L. Correction of the hyponatremia should be undertaken slowly, particularly if the hyponatremia has persisted for several days. The increase in serum sodium should be ≤20 mEq/L during the first 48 h of treatment. Children who experience too rapid an increase in the serum concentration of sodium may suffer neurologic insults and central pontine myelinolysis.
Some chemotherapy agents can interfere with several enzyme activities. Alkylating agents such as cyclophosphamide inhibit pseudocholinesterase activity. The effect may persist for 3–4 weeks after the final dose. Accordingly, the use of succinylcholine may cause prolonged muscle paralysis, and a reduction of the dose is indicated in such patients [45]. Procarbazine exerts inhibitory effect on monoamine oxidase. Because of potential synergistic action, barbiturates, antihistaminic, phenothiazines, opioids, and tricyclic antidepressants should be used with caution.
16.2.1.2 Radiation Toxicity
Toxicity from radiation is unavoidable, but children are particularly prone to developing sequelae because of their young age. The duration, dose, and interval of radiation therapy together contribute significantly to the early side effects of the radiation, whereas the dose and age of the child contribute to the late side effects. In general, the sequelae develop in the organ being irradiated, although the singular exception is the development of subsequent solid neoplasms as outlined below [46, 47].
All tissues damaged by radiation are susceptible to inflammation and subsequent fibrosis. For example, radiation to the neck may cause the tissues to stiffen and fibrose, which in turn distort and narrow the airway leading to a potentially difficult airway. Thoracic radiation may affect both the heart and lungs. Cardiac manifestations may include pericarditis, effusions, cardiomyopathy, valvular fibrosis, and conduction abnormalities [48]. To minimize cardiac toxicity, radiation doses are limited to <25–30 Gy. The lungs are particularly at risk for fibrosis. Both fibrosis and interstitial pneumonitis can occur but are more likely to result from chemoradiation combination therapy. In the abdomen, radiation may cause progressive nephrotoxicity at doses exceeding 20 Gy. Gastrointestinal manifestations include malnutrition, chronic enterocolitis, and bowel obstruction. Whole-brain radiation may cause long-term diminution in cognitive function and intelligence quotient, the severity of which depends on the age of onset of the radiation and the magnitude of the dose. The younger the child and the larger the dose, the greater the impact on cognitive function, with learning disabilities and memory loss more prominent than generalized intellectual impairment. Radiation therapy of the sella turcica with doses that exceed 35–40 Gy may cause pituitary dysfunction manifested by isolated hypothyroidism and growth retardation to panhypopituitarism. Finally, both conductive hearing loss and sensorineural hearing loss have been reported after cranial radiation. Other common sequelae of radiation therapy include blistering of the skin and non-melanoma skin cancer, hair loss, nausea, and anemia. Perhaps the most worrisome complication after radiation therapy is the risk of developing a subsequent solid malignant neoplasm. These occur in up to 47 % of children after a 10-year or greater latency period after radiation. The risk of developing a neoplasm that is distinct from the original primary neoplasm increases the younger the child at the time of the radiation exposure and the greater the dose of the radiation. Examples of solid subsequent neoplasms include thyroid, breast, brain, sarcoma, and others.
16.2.2 Anterior Mediastinal Mass (AMM)
Children with AMMs require general anesthesia and/or sedation for a tissue (lymph node) biopsy, CT scan or MRI for diagnosis, or indwelling central line for chemotherapy [49]. Children with these tumors present a significant risk for anesthesia, since cardiac arrest has been reported in the past. Understanding the pathophysiology of the disease enables the clinician to anticipate complications and prepare the anesthetic to avoid them.
Four tissue types comprise most AMMs in children: lymphomas, teratomas, thymomas, and thyroid with lymphomas being the most common, 45 % of AMMs [49]. The most rapidly growing tumor in the anterior mediastinum is the lymphoblastic T-cell lymphoma, a non-Hodgkin’s lymphoma, which has a doubling time of only 12–24 h. These children may present with minor findings (e.g., night sweats) that rapidly progress over 1–2 days to life-threatening problems (e.g., orthopnea, superior vena cava syndrome). In children, anesthesia is usually required to delimit the extent of and tumor effects on mediastinal structures in radiology as well as for tissue biopsy and chronic chemotherapy access in the operating room.
The decision to proceed with local, regional, or general anesthesia depends on the age and level of cooperation of the child, the extent of mediastinal organ involvement, and the access of the node or tumor being biopsied or the planned procedure. A multidisciplinary team that includes the surgeon, anesthesiologist, and oncologist should review all radiological and preoperative data before embarking on the surgery.
Preoperatively, all children should have a chest X-ray (anterior-posterior and lateral views) as well as a preoperative echocardiogram. The latter is particularly helpful in determining whether the right atrium, pulmonary artery, pericardium, and pericardial sac are involved with the tumor. Although echocardiographic examinations are performed awake and cannot rule out compression of these structures during anesthesia, they can identify whether the structures may be at risk during anesthesia.
Those children who can tolerate the surgery under local anesthesia and sedation are managed in this manner. However, this is not usually possible in younger children, in children who cannot tolerate local anesthesia and sedation, and in those whose tumor severely compromises the airway and/or pulmonary artery. In such instances, a 12–24 h course of intravenous steroids or limited radiation should be considered and discussed with the oncologists to shrink the tumor to facilitate an anesthetic and reduce the risk of cardiac arrest [50]. The risks associated with administering a brief course of steroids or radiation are infrequent but may include widespread tumor necrosis that may both render the diagnosis of the cell type difficult and possibly trigger tumor lysis syndrome [51]. Some oncologists are reluctant to treat these children with steroids because of the potential difficulty in establishing the tissue diagnosis should extensive tumor necrosis ensue. Establishing the tumor type is critical for determining the treatment regimen for the specific tumor type. Thus, a balance between the oncologist’s and anesthesiologist’s requirements must be sought.
For most children who require a radiological investigation, tumor biopsy, or chemotherapy access, general anesthesia with spontaneous respiration and avoiding paralysis are the optimal prescription [49]. If the child cannot lie flat, anesthesia can be induced and the trachea intubated with the child positioned in the left lateral decubitus or less desirably, in the sitting position. The trachea should be intubated at induction of anesthesia to ensure a patent airway should it become necessary to turn the child prone to reverse circulatory collapse. Tracheal intubation is performed without muscle relaxation to preserve spontaneous respiration. Spontaneous respiration best preserves the negative intrathoracic pressure gradient to suspend the tumor above the mediastinal structures and avoid pressure on the pulmonary artery and right atrium as well as the tracheobronchial tree. Maintaining spontaneous respiration preserves negative intrathoracic pressures, although simply induction of anesthesia may decrease the magnitude of the negative intrathoracic pressure sufficiently to allow the tumor to press on cardiac vessels. Therefore, one must always monitor the cardiac output (via the capnogram; see below) and be prepared to emergently reestablish circulation. The latter may be achieved by either turning the child prone or applying one towel clip at the sternal notch and one at the xiphoid junction in the anesthetized child and lifting the sternum to restore patency of the cardiac vasculature (usually the pulmonary artery). The capnogram is a very useful monitor to confirm the adequacy of the pulmonary circulation (and cardiac output); the sudden loss of or reduction in the capnogram may herald compression of the pulmonary artery before systemic cardiovascular sequelae occur. Unless the femoral artery and vein have been cannulated before induction of anesthesia, it is exceedingly unlikely that extracorporeal membrane oxygenation could be instituted rapidly enough to restore the circulation in a child who arrests with an AMM.
16.2.3 Airway Lesions
Mucositis and xerostomia are possible sequelae of chemotherapy/radiation therapy developing within weeks of beginning treatment. Mucositis and ulcerative lesions can also occur after hematopoietic stem cell transplant as a part of GVHD. This can result in pseudomembrane formation, supraglottic edema, friability/bleeding, and the potential for aspiration [37]. Irradiation of the neck can lead to fibrosis and airway distortion (subglottic edema, supra and subglottic stenosis, hypoplasia of the jaw, and chondronecrosis of the epiglottis, arytenoids, and trachea) [46]. Concerns for difficult airway, poor LMA seating, and the need for smaller ET tubes may result. For children who received HSCT and required mechanical ventilation, 30 % of intubations were reported to be difficult, most often because of bleeding or edema from mucositis [46].
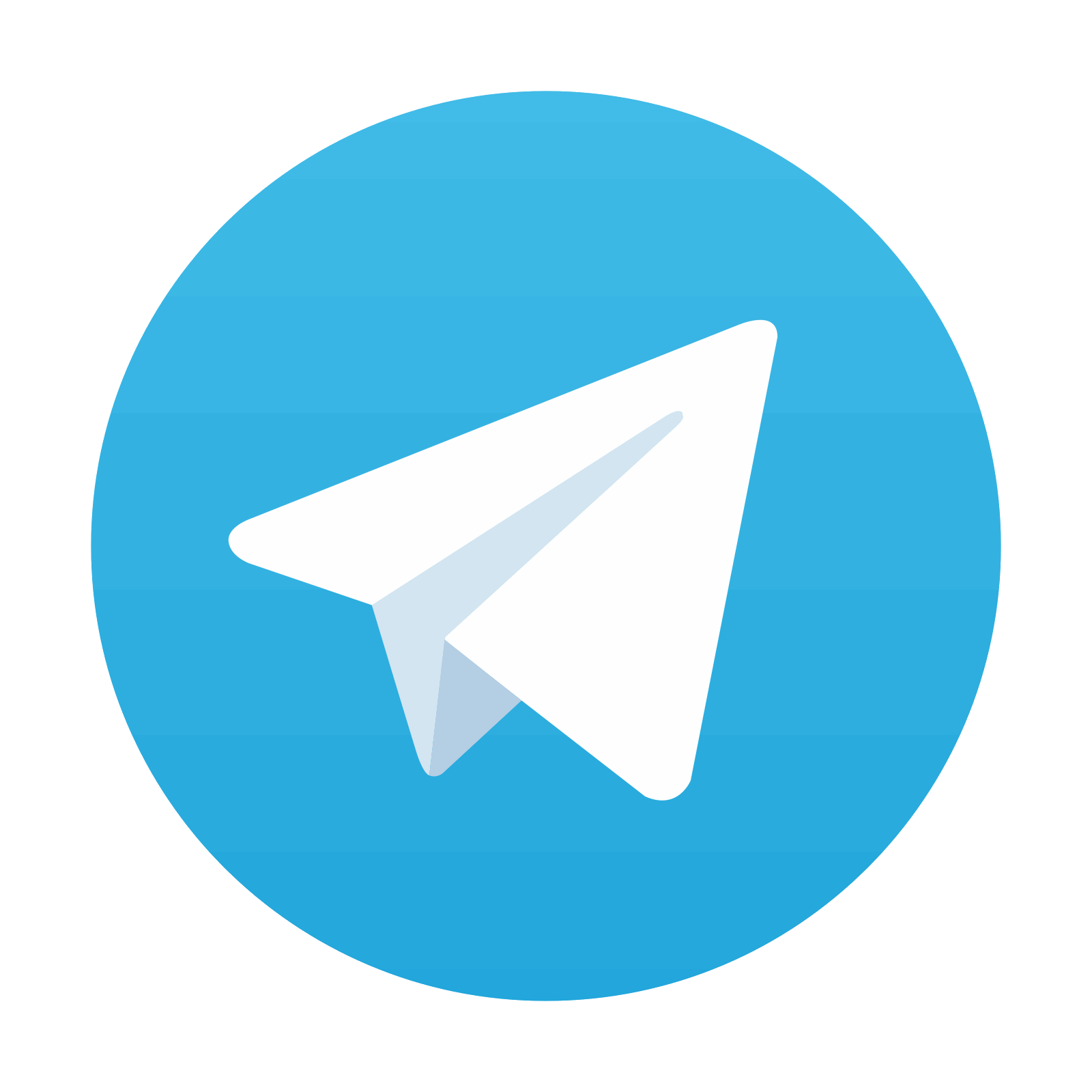
Stay updated, free articles. Join our Telegram channel

Full access? Get Clinical Tree
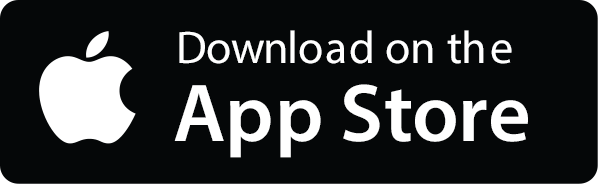
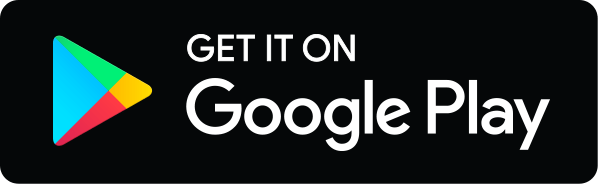