Fig. 2.1
Schematic of cross section of a spinal nerve
2.2.2 Current Intensity and Duration
Whether or not a nerve is stimulated depends on a combination of both the strength and duration of the stimulus and the polarity of the electrode. All modern nerve stimulators produce a square wave pulse of current. The total energy (or charge) applied to the nerve (Q) is a product of the current intensity (I) and the duration of the stimulus (t): Q = (I)(t). The relationship between the characteristics of the nerve, its excitability, and the current required to stimulate it is described by the formula
(Fig. 2.2), where I is current required, Ir is the rheobase, C is the chronaxie, and t is the duration of the stimulus. Rheobase is the minimum current (in mA) required to stimulate a nerve using a long pulse duration or pulse width. As seen in Fig. 2.2, the current required to stimulate the nerve is at a minimum once the pulse duration exceeds 0.3 ms. The chronaxie is determined from the strength-duration curve and is defined as the duration of stimulus (in ms) required to stimulate the nerve at twice the rheobase; in other words, the stimulus applied is twice the strength of the minimum strength required to stimulate the nerve. As Raj et al. [2] stated, “The chronaxie can be used as a measure of the threshold for any particular nerve and is useful when comparing different nerves or nerve fiber types.” The pulse width can therefore be varied in order to target different fiber types. In mammalian peripheral nerves, the respective chronaxies are Aα fibers, 0.05–0.1 ms; Aδ fibers, 0.17 ms; and C fibers, 0.4 ms.



Fig. 2.2
Stimulation curve plotting current intensity and pulse duration
2.2.3 Rate of Current Change
Regardless of the stimulus intensity, a rate of current change that is too low will reduce nerve excitability.
Low subthreshold intensity or slowly increasing rates will inactivate sodium conductance and prevent depolarization – this is termed accommodation.
2.2.4 Polarity of Stimulating and Returning Electrodes
The polarity of the needle will affect its ability to stimulate the nerve at a given current. If the needle is connected to the anode, the current required to stimulate the nerve will be up to four times greater than if the needle is attached to the cathode. The flow of current within the needle when it is attached to the cathode produces an area of depolarization around the needle tip. This reduces the resting membrane potential of the neuronal cell membrane, causing depolarization and an action potential. When the needle is connected to the anode, the flow of current produces hyperpolarization in the area around the needle tip and leads to a higher current being required for stimulation. This phenomenon is known as preferential cathodal stimulation (Fig. 2.3) and refers to the significantly reduced current that is required to elicit a motor response when the cathode is used as the stimulating electrode. Hence, it is normal practice to attach the cathode to the needle. The anode is then attached to the patient’s skin and acts as a returning electrode to complete the circuit. It was previously thought that the distance between the needle and the returning electrode was an important contributor to the resistance of the entire circuit; however, it is now known that the overall resistance remains much the same regardless of where the returning electrode is positioned.


Fig. 2.3
Preferential cathodal stimulation. The flow of current within the needle when attached to the cathode causes depolarization around the needle tip. This reduces the resting membrane potential of the neuronal cell membrane, resulting in an action potential
2.2.5 Distance-Current Relationship
As one would expect, the further the distance between the stimulating electrode and the nerve, the greater the current that is required to stimulate the nerve (Fig. 2.4). This relates to the current intensity at a given distance from the stimulating electrode and is described by Coulomb’s law, I = k(i/r 2), where I = current required, k is Coulomb’s constant, i = minimal current, and r = distance from nerve. From this equation it can be seen that the current required is inversely proportional to the square of the distance from the nerve. Hence, small changes in the distance from the nerve greatly affect the current required. The current required to stimulate the nerve at 2 cm away can be 400 times greater than that required to stimulate the nerve when the electrode is in direct contact with the nerve.


Fig. 2.4
Distance-current curve. Noninsulated needles require more current than insulated needles at the same distance from the nerve and have less discrimination of distances as the needle approaches the nerve. The current threshold is minimal (0.5 mA) for insulated needles when the needle is on the nerve
Another factor in determining whether or not the nerve will be stimulated at a particular distance and current is the pulse width of the stimulus. As shown by the pulse width-current curve, short pulse widths (i.e., 0.04 ms) are a better indicator of the distance between the nerve stimulator and the nerve, based on changes in current (Fig. 2.5). With long pulse widths (i.e., 1 ms), there is little difference in the current required to stimulate the nerve, regardless of whether the stimulating needle is in direct contact with the nerve or 1 cm away. In contrast, at a pulse width of 0.04 ms, there is a large difference in the stimulating current required when comparing direct contact with the nerve versus a distance of 1 cm away.


Fig. 2.5
Current intensity at different pulse widths. A shorter pulse width requires more current with greater nerve-needle distances but is a good discriminator
When undertaking a peripheral nerve block, an initial current of between 1 and 2 mA (pulse width of 0.1–0.2 ms) is used to elicit a response superficially. Accurate placement of the needle is indicated when a response is still generated once the current is reduced to between 0.2 and 0.5 mA. If stimulation of the nerve continues at a current below 0.2 mA, then intraneural injection is possible and the needle should be repositioned. Thus, nerve stimulation below 0.2 mA has a high positive predictive value for intraneural placement of the needle.
Several recent studies have raised concerns that intraneural injection can occur in the absence of nerve stimulation within a range that is normally considered a risk for intraneural injection. Bigeleisen et al. [3] examined supraclavicular blocks and found that in 54 % of patients intraneural needle placement was associated with a motor response in the 0.2–0.5 mA range. These results contradicted the previously established guidelines which stated that a motor response below 0.2 mA indicated intraneural needle placement. Robards et al. [4] combined ultrasound and nerve stimulation for localization of the sciatic nerve at the popliteal fossa and found that, in 20 of 24 patients, a motor response was not obtained until the needle tip was advanced into an intraneural location. Given these findings, other methods of determining intraneural placement of the needle, such as changes in electrical impedance, have been explored.
Percutaneous electrode guidance can be used to determine the optimal site for needle insertion. The negative electrode of the nerve stimulator connects to a commercially available surface electrode (0.5 cm diameter) (Fig. 2.6). The initial current used is generally 5 mA with a 0.2 ms pulse width. The current is then reduced to a minimum using the technique explained above. The widespread use of ultrasound has led to a significant reduction in the use of percutaneous guidance systems.


Fig. 2.6
Surface electrodes for percutaneous electrode guidance. The large diameter (0.5 cm) electrodes attach to the negative electrode of the nerve stimulator
2.2.6 Current Density of Electrodes and Injectates
At the needle tip, the conductive area for current flow will modify the current density and response threshold. Small conductive areas will condense the current and reduce the threshold current needed for motor responses. The needle/catheter-tissue interface can also affect the density of the current, as the area of conductance can change with different injectates and with different tissue compositions. Whether the injectate has conducting properties or not will significantly affect the current at the needle tip.
2.2.7 Electrodes
Types of electrodes include insulated and noninsulated needles and stimulating catheters. Insulated needles have nonconducting shafts (e.g., Teflon) that direct the current density to a sphere around the uncoated needle tip – the smaller the conducting area, the higher the density of current. This means that a lower stimulating current can be used to elicit a motor response. Noninsulated needles are bare metal and transmit current throughout their entire shaft. The current density at the tip is therefore much lower as compared to insulated needles, meaning that noninsulated needles often require more than 1 mA of current for nerve stimulation. Stimulating catheters are similar to insulated needles, although when normal saline is used as a priming solution or a dilating medium, a much higher threshold current is often required to determine correct placement due to the dispersion of current by the ionic solution.
2.2.8 Injectates
Injection of ionic solutions such as local anesthetic or normal saline results in an increase in the conductive area at the needle tip and an increase in the threshold requirement for stimulation of the nerve (Fig. 2.7). This effect, rather than the displacement of the nerve from the needle tip, is responsible for the abolition of motor response with test dose of local or normal saline (the Raj test).
Fig. 2.7
Conductive properties of electrodes and injectates. Top row, insulated needles have a small conducting area, allowing low threshold current stimulation, while noninsulated needles transmit current through their entire length and have a lower current density at the tip. Bottom row, conducting solutions (e.g., saline or local anesthetic) increase the conductive area at the needle tip and increase the threshold current requirement. Nonconducting (nonelectrolyte) solutions (e.g., D5W) reduce the conductive area at the tip and increase the current density. The motor response from nerve stimulation after D5W injection remains the same (or is augmented) during needle/catheter placement
The use of nonelectrolyte/nonconducting injectates, such as D5W, reduces the conductive area and increases the current density at the needle tip, resulting in maintenance or augmentation of the motor response at a low current (<0.5 mA).
Nerve stimulation is clearly sensitive to changes at the tissue-needle/catheter interface. In a physiological context, the electrical field surrounding the needle tip and the conductive area generated by the needle tip are nonuniform, occasionally leading to an unstable electrical stimulation response.
During advancement of a stimulating catheter, it may be beneficial to use nonconducting solutions to dilate the perineural space, because as long as the tip of the stimulating catheter stays in proximity to the nerve, the use of nonconducting solutions will reduce the current leakage and promote continued motor response during advancement of the catheter.
2.2.9 Electrical Impedance
At the most basic level, impedance is defined as resistance in an AC circuit. It is calculated as the voltage-current ratio for a single complex exponential at a particular frequency. Impedance is highly sensitive to tissue composition and has been shown to vary depending on the water content of the tissue. A significant difference between extraneural and intraneural impedance, resulting from differences in the physical composition of tissue components, has been demonstrated in the porcine sciatic nerve [5]. This difference in electrical impedance may be explained by variation in water and lipid content between the intraneural and extraneural space (Fig. 2.8): the intraneural compartment contains considerably greater amounts of nonconducting lipid and lower water content (5–20 % by weight) compared to the surrounding muscle (73–78 % water by weight).


Fig. 2.8
Differences in intra- and extraneural impedance. This may be explained by variation in water and lipid content between the two spaces
The highly variable response to nerve stimulation as observed during ultrasound-guided regional anesthesia may be explained in part by the significant difference between intraneural and extraneural tissue impedance at different sites along the same nerve. An inverse relationship between electrical impedance and current threshold was demonstrated for the median nerve [6]; stimulation of the nerve in the axilla required significantly higher current thresholds than at the elbow. The lower impedance of the axillary muscle tissue may have produced a region of highly conductive tissue around the needle tip, dispersing current away from the nerve. Higher current settings may be required when a stimulating needle is advanced through low-impedance muscle tissue. On the other hand, lower current settings may be needed for nerves located in high-impedance fat or connective tissue.
A recent study assessing accidental nerve puncture during ultrasound-guided blocks in adult patients demonstrated the utility of electrical impedance in clinical scenarios [7]. It was shown that a >4.3 % increase in impedance may indicate intraneural needle placement. To date, an investigation of the use of electrical impedance in guiding needle placement in pediatric regional blocks has yet to be performed.
2.3 Electrical Epidural Stimulation
Electrical stimulation of an epidural catheter can confirm epidural placement and can be used to determine the optimal position to deliver postoperative analgesia. This technique is particularly relevant in children where small distances can significantly alter the nerve distribution blocked by the epidural.
The epidural stimulation test (aka the Tsui test) [8] applies similar principles to those of peripheral nerve blockade, that is, using electrical pulses and the current versus nerve-distance relationship. This test possesses between 80 and 100 % positive predictive value for epidural placement and has been effective in guiding catheters within two segmental levels of the target level, as confirmed by radiological imaging. This can facilitate appropriate placement and allow adjustments in the event of catheter migration, kinking, or coiling. In addition to confirming and guiding epidural catheter placement, this test has the potential to detect intrathecal, subdural, or intravascular catheter placement. The principles of this test can be applied to both catheter and single-shot techniques with both epidural and caudal approaches.
2.3.1 Test Equipment and Procedure
There are specialized kits available for use in electrical epidural stimulation (Tsui epidural kit, Pajunk, Geisingen, Germany). A conventional epidural kit can also be modified for electrical stimulation. This test is designed to be used in addition to the traditional loss-of-resistance technique:
1.
Anesthetize or sedate the patient without the use of muscle relaxants, and position them appropriately. Insert the epidural via the traditional loss-of-resistance technique, and advance the catheter into the epidural space. The epidural catheter must contain a metal coil to enable electrical stimulation.
2.
After sterile preparation, connect a nerve stimulator to the epidural catheter using an electrode adaptor (e.g., Arrow-Johans ECG adaptor, Arrow International Inc., Reading, PA, USA) (Fig. 2.9).


Fig. 2.9
An adaptor (Arrow-Johans ECG Adaptor) is attached to the connector of the epidural catheter (19 G Arrow FlexTip Plus); the adaptor and catheter are filled with normal saline, and the cathode lead of the nerve stimulator is attached to the metal hub of the adapter
3.
Prime the catheter and adaptor with sterile normal saline.
4.
Attach the cathode lead of the nerve stimulator to the metal hub of the adaptor. Attach the grounding anode lead to an electrode on the patient’s body surface.
5.
Set the nerve stimulator to low frequency and pulse width (1 Hz, 0.2 ms).
6.
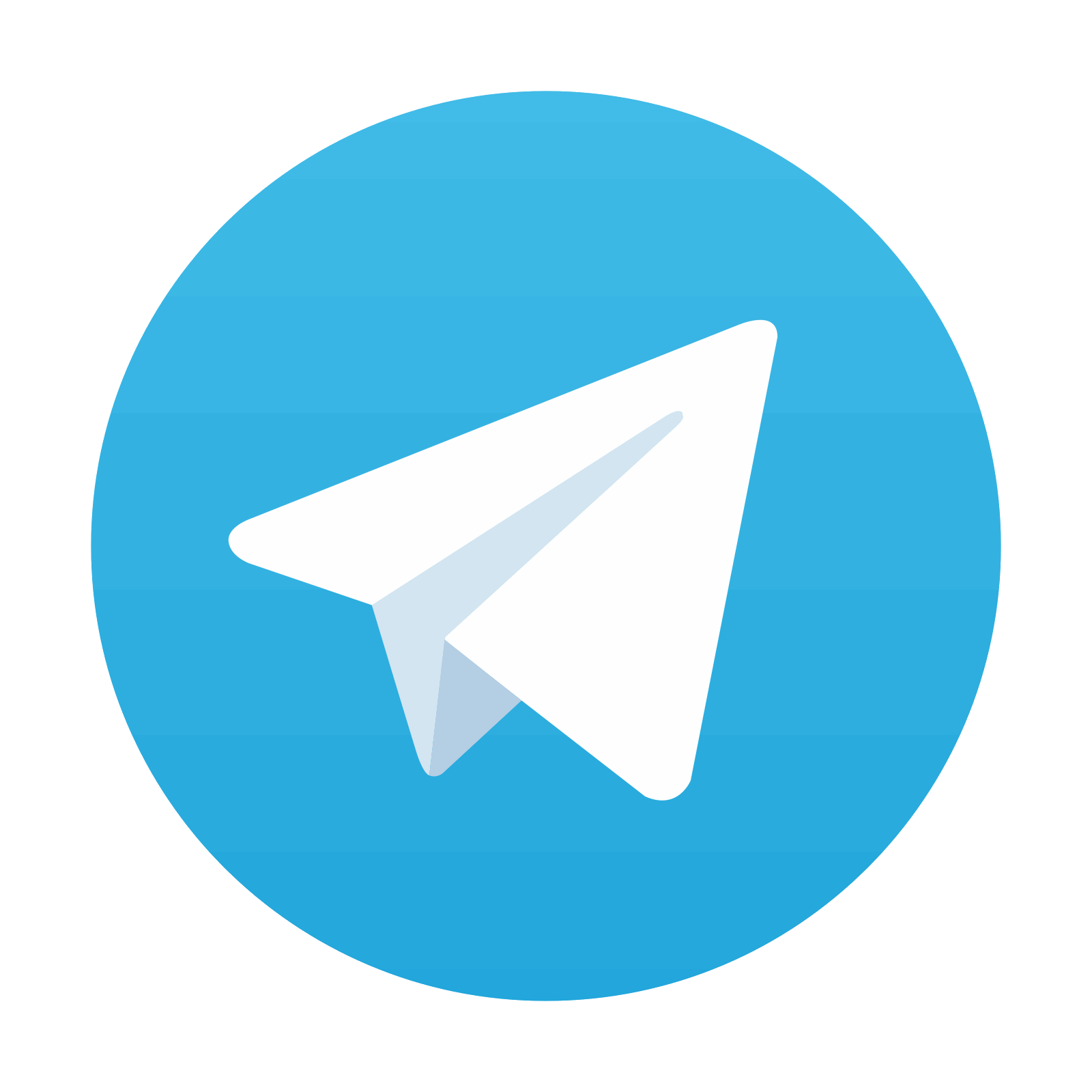
Carefully and slowly increase the current intensity until motor activity begins.
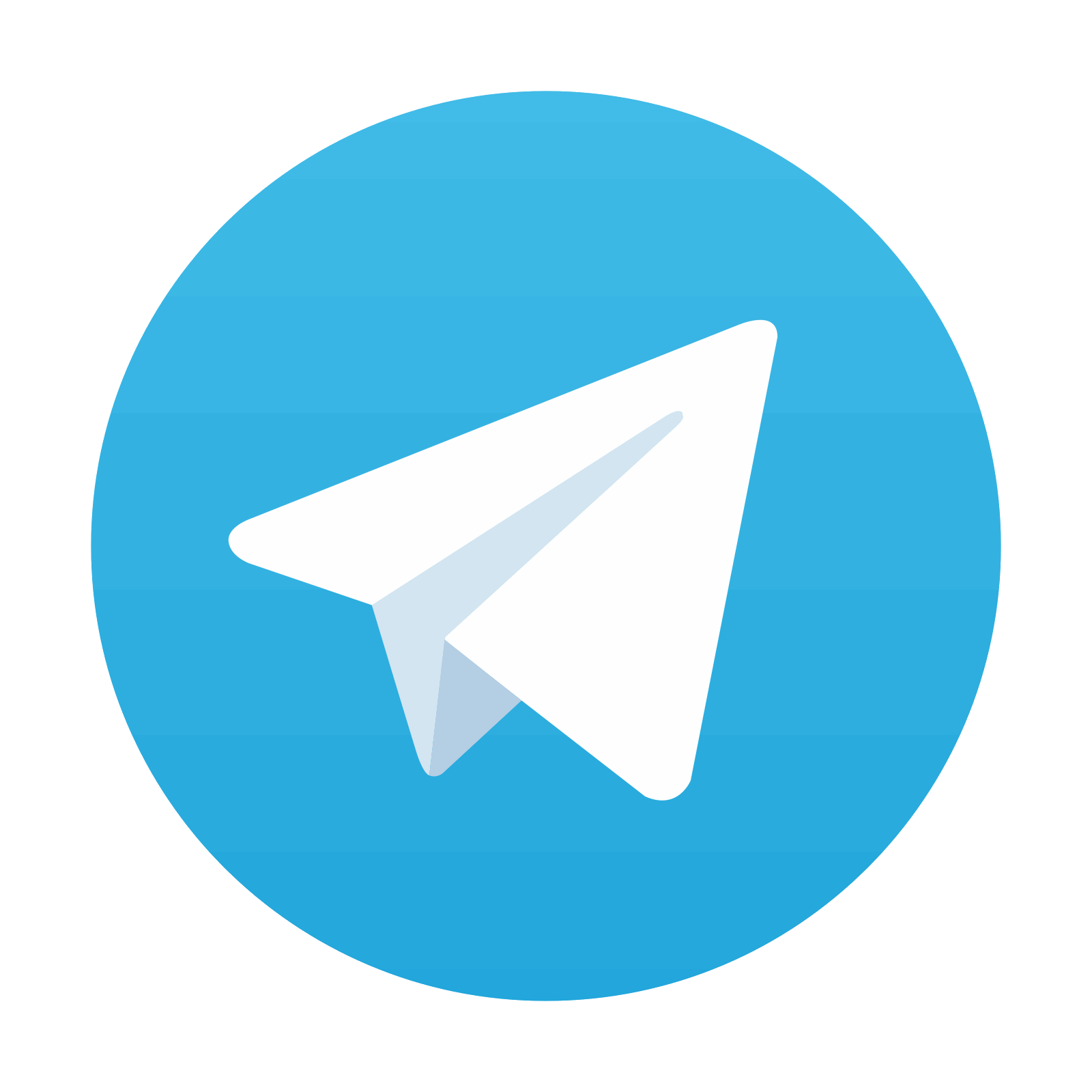
Stay updated, free articles. Join our Telegram channel

Full access? Get Clinical Tree
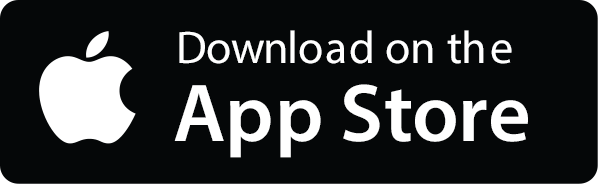
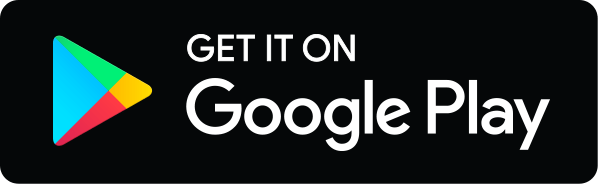
