An anesthesia provider will be present in the operating room throughout the entire anesthetic course
Oxygen delivery will be measured using an oxygen analyzer placed in the inspiratory limb of the anesthesia circuit. This analyzer will contain a low inspired oxygen concentration alarm to prevent the delivery of a hypoxic oxygen mixture
Blood oxygenation will be monitored quantitatively using a device such as a pulse oximeter and qualitatively by assessing patient skin color
During general anesthesia, adequacy of ventilation shall be assessed by observing chest rise and by auscultation of breath sounds and continuous quantitative end-tidal carbon dioxide (ETCO2) monitoring. During moderate and deep sedation, ventilation shall be assessed by qualitative clinical signs
Circulation shall be assessed using continuous electrocardiography (ECG) to monitor heart rate and blood pressure measurement at least once every five minutes. In addition, circulation shall be continuously monitored, either through the use of pulse oximetry or invasive monitoring
Body temperature will be measured when body temperature changes are expected
Arterial Blood Pressure Monitoring
Maintaining arterial blood pressure within a physiologic range is of paramount importance to the anesthesiologist. Arterial hypotension can precipitate numerous adverse outcomes such as stroke, renal failure, and organ hypoperfusion. Conversely, arterial hypertension can lead to increased risk of myocardial infarction, surgical bleeding, and rupture of a preexisting vascular aneurysm leading to cerebral or aortic hemorrhage. Some basic information is described below.
Systolic blood pressure (SBP) is the peak pressure generated during systolic contraction of the left ventricle. Normal SBP ranges from 90 to 140 mmHg (Table 6.2).
Table 6.2
Normal and abnormal blood pressures
Category
Systolic (mmHg)
Diastolic (mmHg)
Hypotension
<90
<60
Normal
90–140
60–90
Mild hypertension
141–160
91–100
Moderate hypertension
161–180
101–110
Severe hypertension
>180
>110
Diastolic blood pressure (DBP) is the trough pressure during diastolic relaxation of the left ventricle. Normal DBP ranges from 60 to 90 mmHg.
Mean arterial pressure (MAP) is the average arterial pressure during a single cardiac cycle and signifies the perfusion pressure of the organs in the body. Normal MAP ranges from 70 to 110 mmHg. MAP can be calculated by the formula MAP = DBP + 1/3 PP, where PP is the pulse pressure. Pulse pressure is the difference between SBP and DBP. Low MAP (<60 mmHg) can lead to ischemia of the organs in the body.
Noninvasive Blood Pressure Monitoring
Noninvasive blood pressure (NIBP) monitoring is accomplished by placing an inflatable cuff around an upper or lower extremity. Traditionally, the upper arm has been utilized, but depending on the patient’s body habitus or the surgical procedure, the forearm and the calf are suitable alternatives. The blood pressure cuff is not placed over arteriovenous fistulas, infective sites, and wounds.
Selecting the appropriate cuff size is critical in achieving reliable results. An appropriately sized cuff should have a width of approximately 40 % of the circumference of the extremity and a bladder length that encircles at least 60 % of the extremity. A cuff that is too small or tight will produce falsely elevated blood pressure readings, while a cuff that is too large or loose will produce falsely low blood pressure readings.
The blood pressure reading is usually taken at 3–5 min intervals. Complications are rare with the use of NIBP monitoring. The two major complications are (1) venous congestion (leading to hematoma formation under and distal to the cuff and causing extravasation of intravascular fluid and edema) and (2) neuropathy from ulnar nerve ischemia. Both of these complications occur most commonly when NIBP monitoring is used for long procedures and at short intervals.
Methods of NIBP Monitoring
NIBP monitoring can be accomplished by using several different methods. The palpatory method involves palpation of a peripheral pulse, inflating the blood pressure cuff placed proximally to the artery to occlude the artery, then slowly releasing the cuff, and palpating the artery for return of pulsations. The latter point denotes the systolic pressure. Diastolic blood pressure cannot be measured by this method. A Doppler probe, if available, can be substituted for the anesthesiologist’s fingers, which will give a more accurate reading of the systolic blood pressure.
The most simplistic method of NIBP measurement is manual auscultation (via a stethoscope and sphygmomanometer) of the brachial artery (Korotkoff sounds) as the blood pressure cuff is slowly deflated (Fig. 6.1). The first sound auscultated represents SBP, and the last sound auscultated represents the DBP. While reasonably accurate, manual auscultation has a number of limitations. It is time consuming and cumbersome, cannot be performed during procedures that require the upper extremities to be tucked next to the patient, and is inaccurate in the presence of low flow states (hypotension) and with increased deflation speed, which underestimates the blood pressure.


Fig. 6.1
Auscultatory method of blood pressure monitoring
In the operating room, an automated NIBP monitor, the oscillonometer, is the most commonly used method. This device has a transducer which is connected to a blood pressure cuff. The cuff is inflated until there is no oscillation present in the transduced pressure. This occurs at a pressure above the patient’s systolic blood pressure. As the cuff slowly deflates, the oscillations increase in amplitude as shown in Fig. 6.2. The pressure at the peak oscillatory amplitude is the MAP.


Fig. 6.2
Blood pressure measurement via an automated oscillonometer
There are two methods used to calculate the SBP and DBP, the maximal slope method and the set ratio method. With the maximal slope method, the SBP is defined as the point at which the oscillations decrease in amplitude most rapidly and the DBP as the point where the oscillations increase in amplitude most rapidly. With the set ratio method, a set ratio of oscillation amplitude to the maximum amplitude is utilized to estimate SBP and DBP. Each manufacturer maintains a proprietary microprocessor algorithm used to perform this calculation. Because of differences between algorithms, the most reliable measurement is the MAP. Limitations of using oscillonometers are similar to those of the auscultatory method. In addition, oscillonometers underestimate MAP in patients with increased pulse pressure (atherosclerosis and decreased arterial compliance) and should not be used during cardiopulmonary bypass (lack of pulsatile flow).
Although less frequently used intraoperatively, there are several other methodologies for noninvasive arterial blood pressure monitoring. Arterial digital photoplethysmography obtains blood pressure measurements through monitoring the artery of a digit. Concerns about this method include its inaccuracy and its inability to detect abrupt changes in blood pressure. Arterial tonometry measures blood pressure using sensors placed on an artery, thereby externally measuring the arterial pressure. For this device to work, a palpable artery must be present and it has been demonstrated that arterial tonometry, rather than measuring absolute systolic or diastolic blood pressures, is more accurate in detecting pulse pressure.
Invasive Blood Pressure Monitoring
Many surgical procedures require close monitoring and manipulation of arterial pressure to assist the surgeon during specific procedures, such as carotid endarterectomy, aortic cannulation, and orthopedic procedures, where significant blood loss is anticipated (Table 6.3). It is important for the anesthesiologist not only to monitor the blood pressure, but also to understand how the device measures and interprets data so that he/she will be able to determine whether a blood pressure reading is reliable or not.
Table 6.3
Indication and contraindications of invasive blood pressure monitoring
Indications | Contraindications |
---|---|
Major surgery requiring beat-to-beat monitoring of blood pressure | Severe peripheral vascular disease with lack of adequate collateral blood flow |
Clinical conditions—hypotension, sepsis, vasopressor therapy, anticipated wide swings in blood pressure | Coagulopathies or bleeding disorders |
Inability to accurately measure noninvasive blood pressure—obese patients | Infected sites |
Repeated blood sampling—arterial blood gas, hematocrit | Extremity burns |
Invasive blood pressure monitoring is the gold standard for the intraoperative measurement of arterial blood pressure. A normal arterial pulse waveform is shown in Fig. 6.3. Normal components of the waveform are:


Fig. 6.3
Normal arterial pulse waveform
Upstroke: the upstroke (anacrotic limb/anacrotic rise) begins at the opening of the aortic valve in early systole. The steepness or rate of ascent and height of this initial upswing is related to the contractility and stroke volume of the left ventricle.
Systolic peak: the systolic peak represents the highest pressure generated by the left ventricle during cardiac contraction. This peak point marks the actual systolic blood pressure of the patient.
Dicrotic limb: the dicrotic limb begins during late systole as the flow of blood out of the left ventricle starts to decrease.
Dicrotic notch: the dicrotic notch represents the closure of the aortic valve and the beginning of diastole.
Diastolic pressure: the end diastole pressure landmark is the location at which the patient’s actual diastolic blood pressure is measured.
Radial artery pressures are higher than aortic pressure because of the distal location of the radial artery. Pressure waveforms from the aortic root (central) to the radial artery (peripheral) are shown in Fig. 6.4. However, it should be noted that during cardiovascular bypass, aortic pressures are higher than radial artery pressures because of decreased vascular resistance of the extremities. Secondly, in patients with severe peripheral vascular disease, there may be a difference in the blood pressure reading in the two extremities, in which case the higher blood pressure reading should be used.


Fig. 6.4
Arterial pulse waveform change from central to the periphery. Pressures are higher in the more distal arteries than central arteries
Technique of Radial Artery Catheterization
Invasive arterial blood pressure monitoring is accomplished through the insertion of a catheter into an artery. Each arterial site has its own advantages and disadvantages (Table 6.4). If the radial artery is used for arterial catheterization, a modified Allen test can be performed prior to cannulation to ensure that the ulnar artery provides adequate circulation to the hand to prevent tissue ischemia or necrosis. To perform the Allen test, pressure is applied to occlude both the radial and ulnar arteries. The patient then opens and closes the fist several times until the hand blanches. The pressure is then maintained on the radial artery while releasing pressure from the ulnar artery. The hand is observed for the return of blood flow or flushing. If the hand color does not return within 5–10 s, the radial artery should not be used for arterial catheterization. The technique of radial artery catheterization is shown in Fig. 6.5.

Table 6.4
Sites of insertion on arterial catheters
Characteristics | |
---|---|
Arteries commonly used | |
Radial | Superficial location, good collateral blood flow via ulnar artery, most commonly used site |
Brachial | Large artery, easily identifiable, less waveform distortion, end artery, kinking of catheter at elbow |
Femoral | Large artery, good accessible site, site prone to infection and thrombosis, hematoma formation, avoid site in children (aseptic necrosis of femoral head) |
Arteries less commonly used | |
Dorsalis pedis | Most distortion of waveform (overestimates systolic blood pressure), avoid site in patients with peripheral vascular disease |
Ulnar | Deep and tortuous artery, blood supply to the hand may be compromised, do not use if repeated attempts were made to cannulate the radial artery of the same hand |
Axillary | Risk of nerve injury, emboli (air or thrombi) to brain, hematoma formation |

Fig. 6.5
Radial artery catheterization. (a) The wrist is positioned supine and dorsiflexed supported by a roll of gauze and stabilized over a board. The skin is then anesthetized with lidocaine. (b) A 20G catheter over a needle is inserted at a 45° angle, and as soon as a blood flash is seen, the angle is dropped to 30°. (c) A wire is passed through the catheter into the artery. (d) The catheter is advanced over the wire into the artery and the needle is completely withdrawn. The catheter is then attached to transducer tubing
The wrist is positioned in the supine and dorsiflexed position supported by a roll of gauze over a board.
Sterile technique is maintained throughout the procedure.
The skin is prepared with a Betadine or a chlorhexidine preparation.
The artery is palpated using the index and middle fingers of the nondominant hand.
The skin over the artery is infiltrated with 0.5–1 ml of 1 % lidocaine with a 25–30G needle.
A 20G arterial catheter with the needle in it is introduced into the skin at a 45° angle.
As soon as flash of blood is seen, the catheter angle is dropped to 30°, and the needle and the catheter are advanced 1–2 mm further into the artery.
The catheter is then advanced into the artery after which the needle is completely withdrawn. Alternatively, a wire is passed through the needle into the artery, over which the catheter is passed into the artery.
The catheter is connected to a saline-filled transducer tubing, which transmits the arterial pressure waveform to a pressure transducer. The transducer then converts this physical energy to an electrical signal, which is then processed, filtered, and amplified before being displayed as an arterial waveform.
The catheter is then fixed firmly to the skin by adhesive tape or may be sutured.
Natural Frequency and Damping
The accuracy of the transducer is dependent on two physical properties of the system: the natural frequency and damping. The natural frequency is the frequency at which a system freely oscillates. The natural frequency of the arterial system ranges from 1 to 30 Hz, while the natural frequency of arterial transducer systems exceeds 30 Hz. This is done to prevent the development of a resonant frequency occurring between the arterial and transducer systems. A resonant frequency occurs when a force with a similar frequency is applied to the system, causing the system to oscillate at its maximum amplitude. If the natural frequency of the transducer is similar to that of the arterial waveform, the system will resonate, causing excessive amplification and distortion of the electrical signal, manifesting as erroneously elevated systolic and widened pulse pressures. The natural frequency of the system is also determined by the properties of its components and may be increased by air in the tubing, the increased tubing length, and the addition of three-way stopcocks.
Damping occurs when there is a condition present that reduces/increases the energy in an oscillating system, thereby reducing/increasing the amplitude of the oscillations. The system can either be overdamped or underdamped. The transducer system is overdamped when there is excessive energy loss, which prevents the system from oscillating. With an overdamped system there is a blunted waveform tracing, with a falsely low SBP and elevated DBP. The transducer system is underdamped when there is excessive energy producing an increase in oscillations. With an underdamped system, there is an overshoot of the pressure waves, with excessively elevated SBP and low DBP (Fig. 6.6). In both scenarios, the MAP remains relatively accurate. Factors that cause overdamping include increased number of stopcocks; air or clots in the tubing; arterial vasospasm; narrow, low, or compliant tubing; narrow arterial cannulas; and kinks in the arterial cannula or tubing. Damping also reduces the natural frequency of the system, allowing resonance, and distortion of the arterial waveform.


Fig. 6.6
Arterial waveform: normal, overdamped, and underdamped
Zeroing the Transducer
To obtain accurate IABP measurements, the transducer must be properly zeroed and leveled. To zero a transducer, the transducer is exposed to atmospheric pressure by turning the stopcock to air and calibrating the pressure reading to zero. This subtracts the atmospheric pressure from the total pressure measurement. Transducers must be leveled with the patient’s heart at the 4th intercostal space in the midaxillary line, to measure blood pressure accurately. Failure to level will result in the hydrostatic pressure of the fluid in the tubing being added to or subtracted from the real blood pressure. A transducer that is higher than the level of the heart will produce a falsely low blood pressure reading, whereas a transducer that is lower than the level of the heart will produce a falsely elevated blood pressure reading. Every 10 cm error in leveling results in a 7.4 mmHg error in the pressure measured.
Complications of Arterial Catheterization
Though a relatively safe procedure, arterial cannulation is not without the risk of complications (Table 6.5). Thrombotic complications, leading to limb ischemia, although rare, may be minimized by using a catheter with the smallest diameter possible, limiting the duration of its use, and by suctioning the catheter during removal. Infectious complications are also rare but increase with duration of the indwelling catheter. Aseptic technique should be used for insertion at any site. Other complications include air embolism, fistula formation, skin necrosis, and aneurysm formation. A pulse oximeter can be placed on the same hand as the radial artery catheter to monitor perfusion.
Table 6.5
Complications of arterial catheterization
Bleeding (disconnection) and hematoma formation |
Infection—bacteremia, cellulitis |
Thromboembolism (air bubbles, thrombi) |
Management errors—disconnection, measurement errors, unintended intra-arterial drug injection |
Nerve damage |
Vasospasm |
Limb ischemia |
Pulse Oximetry
The pulse oximeter is one of the standard ASA monitors for any type of anesthetic care from light sedation to general endotracheal anesthesia. A pulse oximeter monitors the oxygen saturation of a patient’s blood (as opposed to measuring oxygen saturation directly through a blood sample). Prior to its use, anesthesiologists had to rely on qualitative assessments of oxygen saturation such as color of the skin or blood. Pulse oximetry first became available in the 1970s, and today it is widely used, making anesthesia care much safer.
Commonly used sites for pulse oximetry are the finger, toe, or the earlobe. SaO2 readings above 95 % are considered normal, while readings below 90 % demand immediate investigation. Additionally, a pulse oximeter reading of 90 % may indicate a PaO2 of less than 60 mmHg. Pulse oximeters become increasingly inaccurate with SaO2 below 75–80 %. It is important to know that pulse oximetry measures solely oxygenation, not ventilation, and is not a substitute for blood gas analysis.
Pulse oximetry relies on the principle that as light passes through a substance, certain wavelengths of light will penetrate the substance and others will get absorbed. Modern pulse oximeters consist of two light-emitting diodes (LEDs) that emit light containing wavelengths in the red (660 nm) and infrared (940 nm) spectra. This light penetrates tissue, typically of a digit or an earlobe, and light that is not absorbed is captured by a photoreceptor on the opposite side of the tissue.
Oxyhemoglobin absorbs more infrared light and allows more red light to pass through, while deoxygenated hemoglobin absorbs more red light and allows more infrared light to pass through (Fig. 6.7). The transmitted light is processed to eliminate scatter and absorbance by tissues other than blood, the red/infrared (R/IR) ratio is analyzed, and the oxygen saturation (SaO2) is then calculated by the microprocessor from an empiric formula based on calibration curves derived from healthy individuals. The SaO2 is displayed as a waveform produced by the contraction and relaxation of digital arteries during a cardiac cycle. To ensure accuracy the displayed SaO2 is an average of data collected from the prior 5–15 s. Because of this delay, hypoxemia or its treatment may not be immediately recognized.


Fig. 6.7
Absorbance red and infrared light by oxy- and deoxyhemoglobin. Oxyhemoglobin absorbs more infrared light, whereas deoxyhemoglobin absorbs more red light
The waveform of the pulse oximeter display is important in determining the accuracy of the displayed SaO2. Any situation (Table 6.6) that reduces the amount of pulsatile blood flow in the area being measured will affect waveform and SaO2 accuracy. This can be seen with hypotension, use of vasoconstrictors, hypothermia, and low cardiac outputs. The waveform can also be affected by nail polish and fluorescent and xenon light. Another factor that can affect SaO2 measurements is the administration of dyes, such as methylene blue, indocyanine green, and indigo carmine, which transiently cause a falsely decreased SaO2 reading. Hyperbilirubinemia, fetal hemoglobin, and acrylic fingernails do not affect the accuracy of pulse oximetry.
Table 6.6
Factors affecting pulse oximeter reading
Factor | Notes |
---|---|
Decreased perfusion | Hypotension, use of vasoconstrictors, hypothermia, low cardiac output states |
Nail polish | Blue, green, pink, black |
Dyes | Methylene blue, indigo carmine |
Methemoglobin | SaO2 of 85 % (falsely elevated/depressed) |
Carboxyhemoglobin | Co-oximeter needed to differentiate carboxyhemoglobin from oxyhemoglobin |
Presence of abnormal hemoglobin variants will also affect pulse oximetry readings. Carboxyhemoglobin (COHb) is frequently encountered in smokers and in those who have suffered smoke inhalation. COHb absorbs light of the same wavelength (660 nm) as oxyhemoglobin producing misleading SaO2 readings. Methemoglobin (MetHb) absorbs equal amounts of light at both 660 nm and 940 nm. Since the ratio of the red and infrared absorbances is equal to 1 at an oxygen saturation of 85 %, the presence of significant methemoglobinemia will produce an SaO2 of 85 %. This will be falsely elevated in the presence of hypoxia and falsely depressed in the presence of adequate oxyhemoglobin concentrations. If the presence of abnormal hemoglobin variants is suspected, co-oximetry can be used. A co-oximeter is a device that can measure more than two wavelengths of light at a time and can be used to determine the presence of carboxyhemoglobin or methemoglobin.
Electrocardiography
Electrocardiography (ECG), which measures the electrical activity of the heart, is a standard ASA monitor that is continuously displayed in every patient undergoing anesthesia. It can be used as both a cardiac monitor, to detect the presence of myocardial ischemia or arrhythmias, and a physiologic monitor, to assess excessive autonomic activity. ECG is commonly used to measure the rate and regularity of heartbeats, the size and position of the chambers, the presence of any damage to the heart, and the effects of drugs or devices used to regulate the heart, such as a pacemaker.
ECG Waveform
A cardiac cycle is composed of a P wave, corresponding to atrial depolarization, the QRS complex, corresponding to ventricular depolarization, and a T wave, corresponding to ventricular repolarization. The PR interval represents the signal delay caused by impulse conduction through the AV node, the ST segment represents the period between ventricular depolarization and repolarization, and the QT segment represents the entire period of ventricular depolarization and repolarization (Fig. 6.8, Table 6.7).


Fig. 6.8
A normal ECG tracing
Table 6.7
Significance of ECG waves and intervals
ECG | Characteristics | Duration |
---|---|---|
P wave | Electrical impulse during atrial depolarization traveling from the SA node toward the AV node, spreading from the right atrium to the left atrium | 0.08–0.1 s |
PR interval | It denotes AV node function. The PR interval is measured from the beginning of the P wave to the beginning of the QRS complex and is the time interval the electrical impulse takes to travel from the SA node to reach the ventricles via the AV node | 0.12–0.2 s |
QRS complex | Rapid depolarization of the right and left ventricles. Duration greater than 0.12 s signifies bundle branch block | <0.1 s |
J point | This point denotes the ending of the QRS complex and beginning of the ST segment | |
ST segment | The time period when the ventricles are depolarized | 0.8–0.12 s |
T wave | Repolarization of the ventricles, usually less than 10 mm in amplitude | 0.16 s |
QT interval | Prolonged interval increases risk for ventricular arrhythmias and sudden death | 0.35–0.42 s |
U wave | Usually follows T wave, has low amplitude, or may be absent. May be caused by repolarization of the interventricular system. Prominent U waves are seen in hypokalemia and hypercalcemia |
In the operating room, either a three-lead or five-lead ECG is utilized. With the five-lead ECG, the most commonly displayed leads are II and V5. Lead II is chosen because it is approximately 60° from the right arm to the left leg and is most parallel to the atria, which makes it the most useful lead for accessing the P wave, heart rhythm, and inferior wall ischemia (Table 6.8). Lead V5 is chosen because it lies at the fifth intercostal space over the anterior axillary line making it the most sensitive for detection of anterior and lateral ischemia. When using a three-lead ECG, V5 can be obtained by placing the left arm lead in the V5 position and selecting lead I on the monitor.
Table 6.8
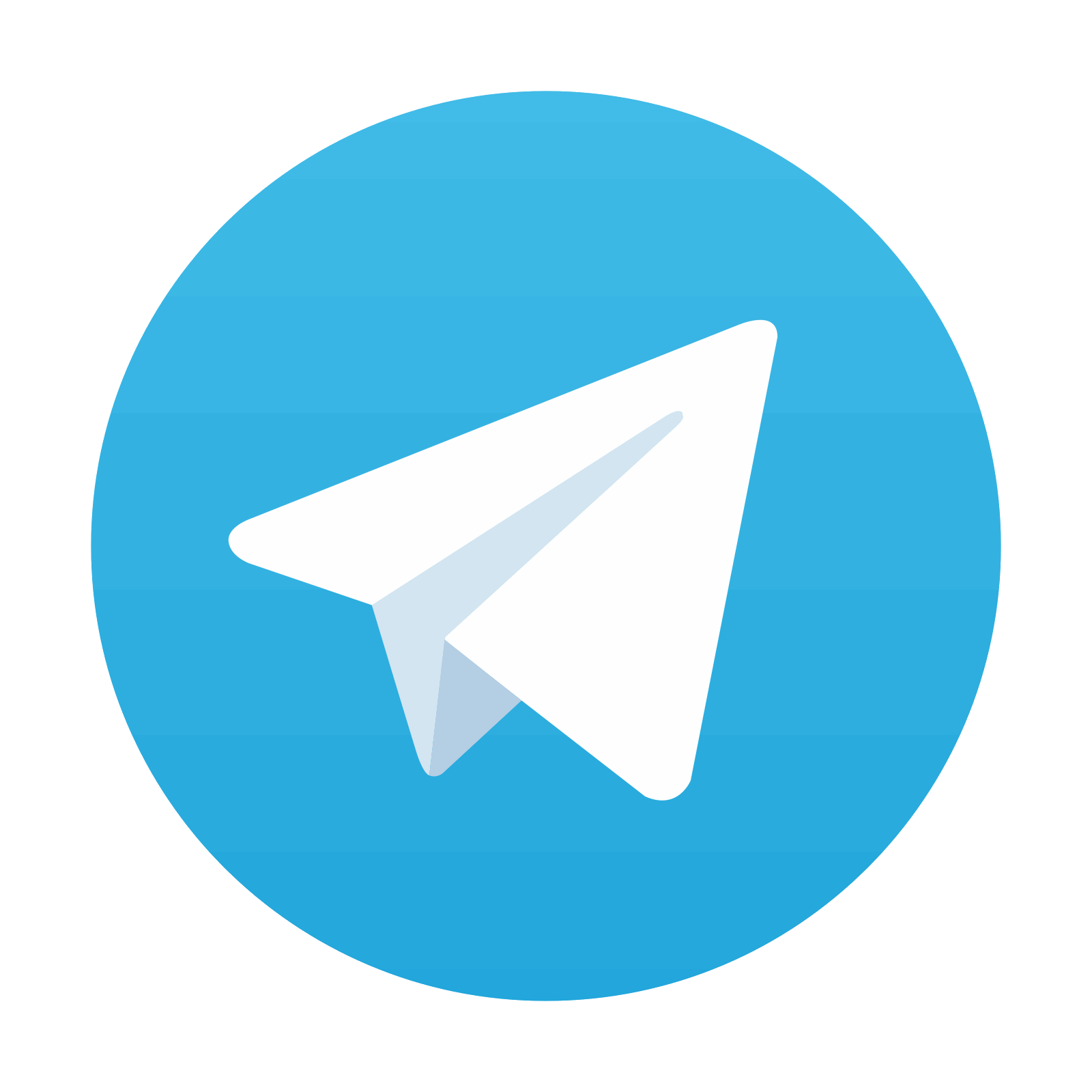
ECG leads corresponding to areas of the heart
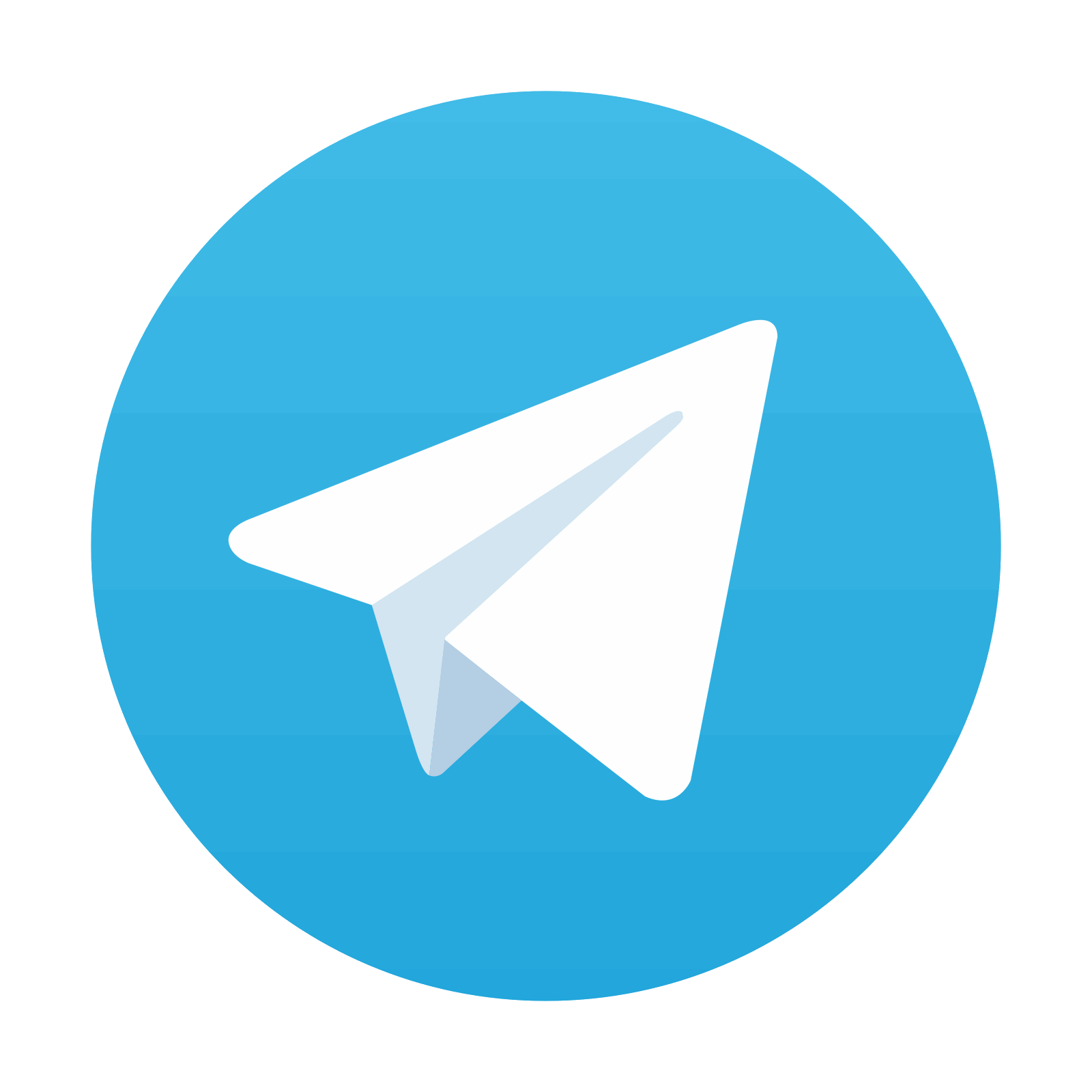
Stay updated, free articles. Join our Telegram channel

Full access? Get Clinical Tree
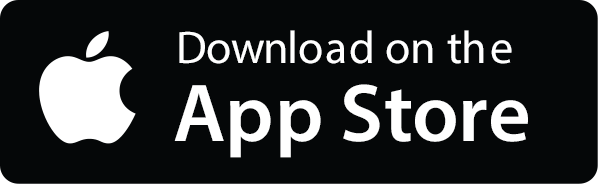
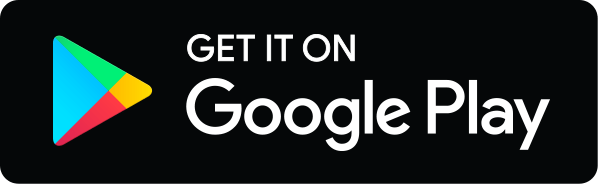
