html xmlns=”http://www.w3.org/1999/xhtml” xml:lang=”en”>
New Paradigms in Sepsis
Mervyn Singer, MD, FRCP, FFICM
Objective
- To challenge existing concepts on sepsis and infection, and to highlight new mechanistic insights and therapeutic developments
Key words: sepsis, bacteremia, mitochondria, stress
Multicenter studies assessing immunomodulatory therapies for sepsis have continued to disappoint for more than 2 decades, leading to calls for new directions.1 As a sad indictment of the lack of real progress in diagnostics, inclusion criteria have barely changed over this time. These are still heavily reliant on a likely diagnosis of infection, evidence of a systemic inflammatory response syndrome, and new-onset organ dysfunction (with or without circulatory shock). Perhaps it is unsurprising that so many promising candidates have fallen by the wayside, as they have all followed the same route: early encouragement from carefully controlled, homogenous laboratory models, some evidence of therapeutic potential in small-scale phase 2 trials, and ultimate rejection when extrapolated to large phase 3 survival studies. Does that mean the drugs are uniformly ineffective? Clearly not. Or does it mean that they aid some people but perhaps even harm others, balancing out the profit–loss equation? Probably true. Or does it mean that they’re often the right drugs but are given at the wrong time and/or the wrong dose and/or the wrong duration? Almost certainly the case. Yet, at the same time, it is clear that sepsis outcomes have improved.2,3 This has not been achieved through any particular new intervention or specific care bundle but, more likely, through earlier identification and treatment and less iatrogenic harm. Using these questions and points as a basis for generating thought and discussion, I shall explore some new paradigms that may cast light on the pathophysiology of sepsis and, perhaps, how it should be managed.
The Infecting Organism—Main Threat or Mere Touchpaper?
Infection triggers an inflammatory response as part of the body’s normal defense mechanism. However, as is described later, some patients are clearly more susceptible to developing an exaggerated systemic response due to as yet undetermined genetic predisposition. This inbuilt predisposition is also affected, both positively and negatively, by environmental factors (eg, concurrent medication) and endogenous factors such as age and comorbidity that influence the immune response.
Clearly, the virulence of the organism, the amount of inoculum, and the location of the infection (eg, peritoneum vs urinary tract) will be important contributors to the degree of systemic inflammation generated. However, it is noteworthy that an identical (although not overwhelming) inoculum of bacteria or fecal slurry to rodents of similar age, gender, upbringing, and often consanguinity will generate a broad and highly variable level of sepsis and resulting mortality.4 Turnbull et al5 found that a similar septic insult delivered to the same mouse species yet at different ages resulted in a significantly higher mortality and a markedly reduced impact from antibiotic therapy. The proinflammatory cytokine response was greater in aged mice, although a high plasma interleukin-6 level at 24 hours predicted mortality at any age.
Provided the experiment is run long enough to ensure full recovery, a model with a 50% mortality rate means that 50% of subjects survive. Notably, such models have highly variable levels of fluid resuscitation, and subjects infrequently receive antibiotics yet are still able to recover. This suggests an individualized propensity to die or, perhaps, an intrinsic inclination to survive. Using a 7-day cecal ligation and puncture mouse model, Osuchowski et al6 demonstrated prognostic capability from plasma cytokine levels taken at 6 hours post insult.
Using a 72-hour resuscitated rat model of fecal peritonitis, we recently demonstrated similar prognostication using 6-hour values of stroke volume and heart rate.7
Great stock is also placed on the presence of bacteremia. However, this generally consists of only a few bacteria per milliliter of blood,8 thus the need to take multiple cultures and to wait 1 or more days to culture enough bacteria to enable a positive identification.9 This bacterial load is hardly enough to generate an inflammatory response in itself. Indeed, transient bacteremia is common after routine procedures including toothbrushing and dental work10; in a small proportion, bacteremia can even persist more than 60 minutes after toothbrushing, yet this does not appear to be a clinical risk, even in critically ill patients in whom this procedure is routinely performed.
So what does bacteremia signify in a sick patient? Possibly an increased risk of distant seeding, causing endocarditis, osteomyelitis, or multiple abscesses. However, this is a rare clinical event, even in the absence of appropriate antibiotics. Does bacteremia perhaps represent a failure of the immune system to either prevent “spillage” or to instantly mop up bacteria that escape from their source into the circulation, and is it thus indicative of a sicker, more immunosuppressed patient? Not necessarily, as the toothbrushing example given above would suggest.
Antibiotics—Crucial Panacea or Adroit Marketing?
Is the evidence base underpinning the necessity of giving early, appropriate antibiotics that compelling? Certainly, the literature is highly conflicting with respect to patient benefit, especially in the sicker patient. Such studies are predominantly retrospective analyses of databases and are heavily criticized for their methodological imperfections.11 Even the most cited retrospective study, purporting to show hour-by-hour increasing detriment with delayed treatment, actually demonstrated a higher mortality in the group treated with appropriate antibiotics before the onset of hypotension compared with those whose treatment commenced in the first 6 hours after shock occurred.12 After giving young and aged mice a similar bacterial inoculum in a peritonitis model, Turnbull et al13 reported a similar bacterial burden in both blood or peritoneal cultures, yet the local and systemic inflammatory response and mortality differed widely with age.13
So, arguably, focusing on the bacteria itself is less crucial than other aspects of care such as restoration of adequate organ perfusion. Clearly, the source of infection has to be dealt with promptly, be it repairing or resecting a bowel perforation, draining an abscess, or debriding or removing infected necrotic tissue, as these conditions if untreated will continue to fuel the fires of inflammation. The above observations should not be viewed at present as a justification to not give appropriate antibiotics when sepsis is first suspected. Yet the safety profile of these agents, other than well-recognized complications such as renal dysfunction, cholestasis, and rash, should not be taken for granted. Apart from having depressant effects on mitochondrial function and turnover,14 antibiotics, particularly those with a bactericidal action, can destroy the bacterial cell wall. This may then lead to a Jarisch-Herxheimer-type reaction15 following release of large amounts of bacterial cell wall products (such as endotoxin) and intracellular constituents (such as DNA) into the circulation directly or via the lymphatics. This, in itself, engenders a systemic inflammatory response through recognition by the host of these specific pathogen-associated molecular patterns (PAMPs) and can be readily seen in human volunteers on injection of endotoxin alone. Because clinical deterioration may be seen following commencement of antibiotics for tuberculosis meningitis and pericarditis, Pneumocystis jiroveci pneumonia, and bacterial meningitis, guidelines for these specific yet diverse infections recommend pretreatment or cotreatment with corticosteroids.16-18 Yet should this also apply to other types of infection, such as peritonitis, where patients not infrequently deteriorate markedly after treatment has begun?
PAMPs, DAMPs, Schmamps
The above observations have been reinforced in recent years by identification of a similar “genomic storm” in patients following diverse insults and by numerous descriptions in animal models of endogenous host molecules released during injury that generate an identical systemic inflammatory response to bacteria or endotoxin.
Approximately 30% of all injured patients with trauma or hemorrhagic shock develop a systemic inflammatory response syndrome and multiple-organ failure that are clinically indistinguishable from sepsis. Using leukocytes sampled from patients and volunteers, Xiao et al19 showed a similar genomic response (albeit varying in duration) to trauma, burn injury, or infusion of low-dose bacterial endotoxin. In more than 5,000 genes whose expression changed 2-fold after severe trauma, 98% showed the same direction of change with burn injury and 88% following endotoxemia.
Damage-associated molecular pathogens (DAMPs) are substances that often reside intracellularly, such as DNA, mitochondria, adenosine triphosphate, and S100 proteins. However, following tissue damage, be it from trauma, hemorrhage, surgery, or infection, DAMPs are released into the circulation, triggering a systemic inflammatory response through direct activation of Toll-like and other pattern recognition receptors.20 Liu et al21 examined the plasma proteome in trauma patients and found that up to 70% of unique proteins measured in the plasma were intracellular components. Indeed, increased plasma DNA levels taken from critically ill patients on ICU admission correlate with worse outcomes.22 Is this simply a biomarker of more tissue damage, and thus disease severity, or perhaps a direct contributor to systemic inflammation? An effect resembling acute respiratory distress syndrome following traumatic injury could be generated in laboratory models by direct injection of mitochondria.23 Likewise, histones, around which nuclear (but not mitochondrial) DNA is wrapped, can have a direct toxic effect on endothelial cells when released either from dying cells or from activated neutrophils into the circulation.24 Chloroquine (an inhibitor of Toll-like receptor activation),25 antihistone antibodies, and activated protein C24 were all able to improve survival in these models by blocking the effects of these DAMPs. With sepsis, the implicit and conventional assumption that receptor activation is derived entirely from the microorganism (and its constituents) can thus be reasonably challenged. A new therapeutic paradigm opens up for sepsis, trauma, and other shock states in preventing and attenuating the impact of excessive DAMP-related inflammation. However, whether this should be targeted at the DAMP itself24 or at a downstream effector such as Toll-like receptor-9 or p38 mitogen-activated protein kinase25 needs further study.
The Multiple Roles of Mitochondria
The mitochondrion is increasingly recognized as central to many of the pathophysiological processes underlying critical illness, including multiple-organ failure.26 Mitochondria have a wide range of functions apart from being the major provider of energy (adenosine triphosphate) to most of the body’s cell types.27 Mitochondria regulate calcium, activate cell death pathways, perform important signaling roles, and serve as the major generator of reactive oxygen species within the body.28-30 Many of the pathways and enzymes involved in drug action or metabolism are located within mitochondria: for example, 3-hydroxy-3-methylglutaryl coenzyme A reductase, which is the target enzyme for statin inhibition.28
Hormone production often involves mitochondrial enzymes. As an example, for cortisol production as part of the acute stress response, cholesterol must be rapidly delivered to Cyp11a1. This enzyme is located on the inner mitochondrial membrane of adrenal cortical cells, catalyzing the first and rate-limiting step in the steroid biosynthetic pathway. The steroidogenic acute regulatory protein mediates the flux of cholesterol from outer to inner membrane. Some of the other downstream enzymes involved in the cortisol pathway, such as other cytochrome P450 or hydroxysteroid dehydrogenase enzymes, are also located within the mitochondria.
Hormones may affect mitochondrial activity directly. Thyroxine controls basal metabolic rate through increases in both cell mitochondrial density and unit mitochondrial energy production. This, however, decreases mitochondrial efficiency, with a significant proportion of electron transfer down the electron transport chain (and thus oxygen consumption) being uncoupled from oxidative phosphorylation and lost as heat.31,32
In sepsis, numerous studies show clear in vivo, ex vivo, and in vitro evidence of mitochondrial dysfunction and damage in sepsis.33,34 This has been demonstrated in a wide range of tissues and models ranging from human and experimental animal to cell lines.35-37 Effects are seen on tissues sampled directly from septic patients or animal models or where isolated cells or primary cell lines are incubated with plasma taken from septic patients.38-40
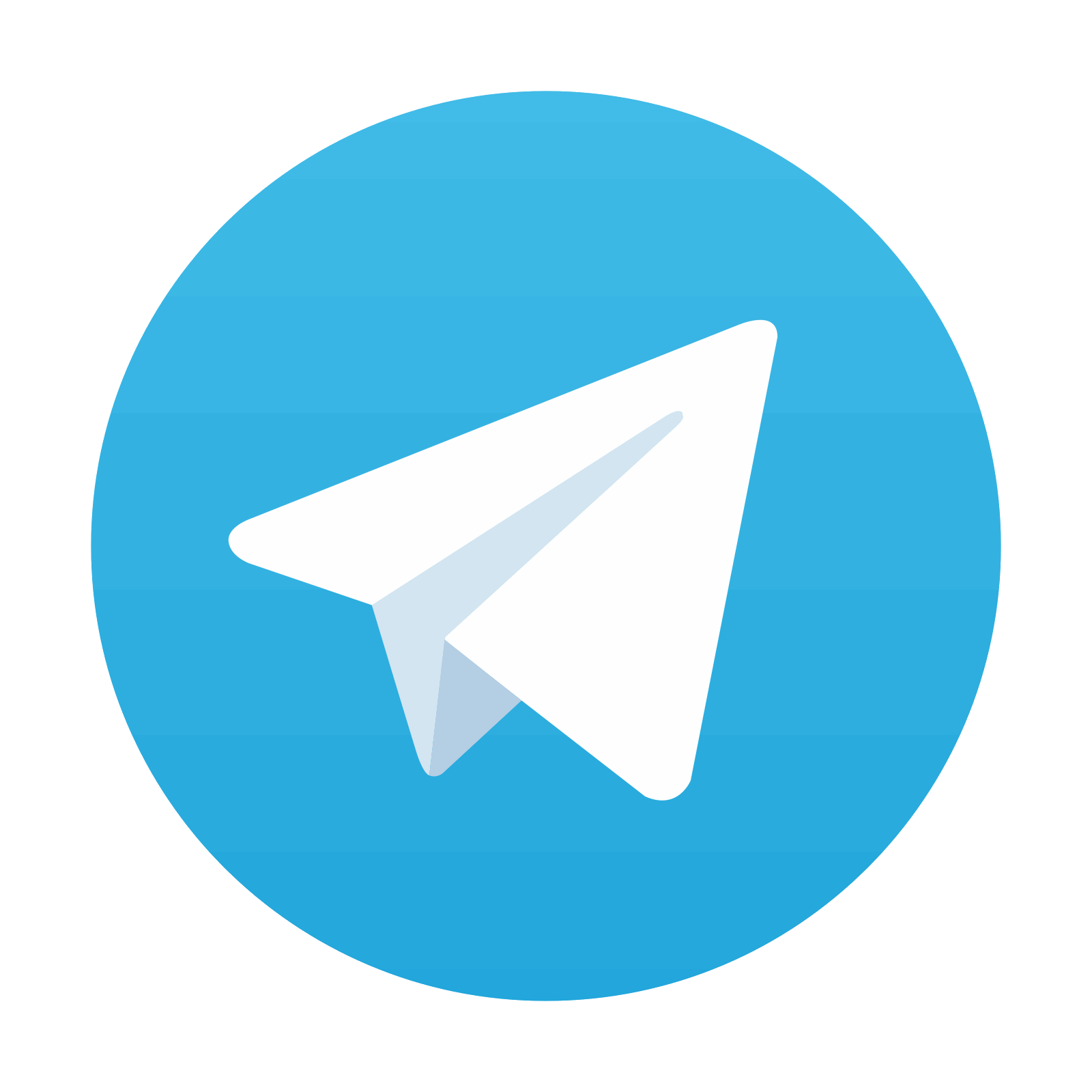
Stay updated, free articles. Join our Telegram channel

Full access? Get Clinical Tree
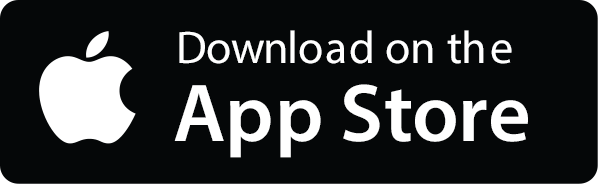
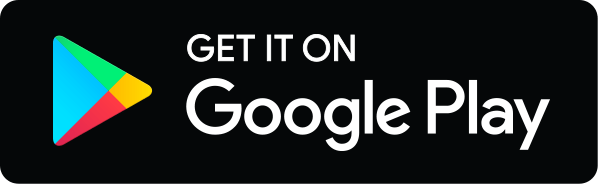