A variety of receptors and ion channels have been identified on dorsal root ganglion neurons and on peripheral terminals of nociceptive afferent fibers. These receptors, including purinergic,5 metabotropic glutamatergic, tachykinin,6 TRPV1 receptor and neurotrophic receptors, and ion channels (e.g., Nav1.8) in primary sensory neurons may also undergo significant adaptation after noxious stimuli, significantly lowering the firing thresholds of nociceptors and critically contributing to the induction and maintenance of neuronal sensitization, which manifest as allodynia and hyperalgesia.7
Primary Hyperalgesia and Secondary Hyperalgesia
In general, tissue injury and inflammation may activate a cascade of events leading to enhanced pain in response to a given noxious stimulus, termed hyperalgesia (e.g., a mild pinprick causing severe pain). Hyperalgesia is defined as a leftward shift of the stimulus–response function that relates magnitude of pain to stimulus intensity. Hyperalgesia is a consistent feature that appears following somatic and visceral tissue injury and inflammation. Hyperalgesia at the original site of injury is termed primary hyperalgesia, and hyperalgesia in the uninjured skin surrounding the injury is termed secondary hyperalgesia. Primary hyperalgesia is usually manifested as decreased pain threshold, increased response to suprathreshold stimuli, spontaneous pain, and expansion of receptive field. Whereas primary hyperalgesia is characterized by the presence of enhanced pain from heat and mechanical stimuli, secondary hyperalgesia is characterized by enhanced pain response to only mechanical stimuli. It is usually accepted that interaction between the proinflammatory mediators and their receptors in nociceptors leads to the induction of primary hyperalgesia, and sensitization of central neuronal circuits processing nociceptive information may account for the secondary hyperalgesia after tissue injury.
Central Nervous System Physiology
Pain transmission from peripheral nociceptors to the spinal cord and higher structures of the CNS is a dynamic process involving several pathways, numerous receptors, neurotransmitters, and secondary messengers. The spinal dorsal horn functions as a relay center for nociceptive and other sensory activity. The ascending pathways convey pain-related activity to the brainstem and forebrain in humans. Forebrain somatosensory cortex (SI and SII) accounts for the perception of sensory-discriminative of peripheral painful stimuli (i.e., the location and intensity of pain). Brain regions in the limbic cortex and thalamus account for the perception of motivational-affective components of pain. Descending projections originating from periaqueductal gray–rostral ventromedial medulla (PAG–RVM) system may either depress or facilitate the integration of painful information in the spinal dorsal horn (Fig. 6-2).

Dorsal Horn: The Relay Center for Nociception
Afferent fibers from peripheral nociceptors enter the spinal cord in the dorsal root, ascend or descend several segments in the Lissauer tract, and synapse with the dorsal horn neurons for the primary integration of peripheral nociceptive information. The dorsal horn contains four major neuronal components: the central terminals of primary afferent axons; intrinsic neurons, which terminate locally or extend into other spinal segments; projection neurons that pass rostrally in the white matter to reach various parts of the brain; and descending axons that extend caudally from several brain regions and terminate in the dorsal horn where they play an important role in modulating the integration of nociceptive information.
The central terminals of primary afferents occupy highly ordered spatial locations in the dorsal horn. The dorsal horn consists of six laminae (Fig. 6-3). Laminae I (marginal layer) and II (substantia gelatinosa) are often referred to as the superficial dorsal horn and are the primary regions where afferent C fibers synapse on second-order neurons. Lamina I contains both projection neurons and interneurons, and all of the neurons in lamina II are small interneurons. Lamina V is the site of second-order wide dynamic range (WDR) and nociceptive-specific (NS) neurons that receive input from nociceptive and nonnociceptive neurons. The NS neurons respond only to noxious stimuli in their peripheral environment, whereas WDR neurons respond to innocuous and noxious stimuli of many types, providing the neuronal mechanism for encoding of the intensity of stimuli. Both types of neurons are believed to be important in the perception of nociceptive information. Myelinated fibers innervating muscles and viscera terminate in laminae I, IV to VII, and the ventral horn, and the unmyelinated fibers from these organs mostly terminate in laminae I, II, and V as well as X.

Interneurons make up the great majority of the neuronal population throughout the dorsal horn. Many dorsal horn interneurons have axons that remain in the same lamina as the cell body, and they also give rise to axons that extend into other laminae. Interneurons in the dorsal horn can be divided into two main functional types: inhibitory cells, which use GABA and/or glycine as their principal transmitter, and excitatory glutamatergic cells. Interneurons in dorsal horn are important for integration and modulation of incoming nociceptive information.
Projection neurons with axons that project to the brain are present in relatively large numbers in lamina I and are scattered through the deeper part of the dorsal horn (laminae III to VI) and the ventral horn. Both the lamina I and the laminae III and IV projection neurons that express the NK1 receptor are heavily innervated by substance P–containing primary afferents. Those in lamina I, together with some of the projection cells in deeper laminae, have axons that cross the midline and ascend to a variety of supraspinal targets including the thalamus, the midbrain PAG, lateral parabrachial area of the pons, and various parts of the medullary reticular formation.
Two types of descending monoaminergic (serotoninergic and norepinephrinergic) axons project from the brain throughout the dorsal horn, mostly terminating in laminae I and II, and are involved in descending pain modulation. Serotoninergic axons in the spinal cord originate in the medullary raphe nuclei, whereas those that contain norepinephrine are derived from cells in the locus ceruleus and adjacent areas of the pons.
Gate Theory
The gate control theory of pain was first proposed by Ronald Melzack and Patrick Wall in 1965 to illustrate the neuronal network underlying pain modulation (a neurologic “gate”) in the spinal dorsal horn. According to this theory, painful information is projected to the supraspinal brain regions if the gate is open, whereas painful stimulus is not felt if the gate is closed by the simultaneous inhibitory impulses (Fig. 6-4). Here is a commonly used example to describe how this neuronal network modulates pain transmission. Usually, rubbing the skin of painful area seems to somehow relieve the pain associated with a bumped elbow. In this case, rubbing the skin activates large-diameter myelinated afferents (Aβ), which are “faster” than Aδ fibers or C fibers conveying painful information. These Aβ fibers deliver information about pressure and touch to the dorsal horn and override some of the pain messages (“closes the gate”) carried by the Ad and C fibers by activating the inhibitory interneurons in the dorsal horn. This hypothesis provided a practical theoretical basis for some approaches such as massage, transcutaneous nerve stimulation, and acupuncture to effectively treat pain in clinical patients.

Central Sensitization of Dorsal Neurons
Peripheral inflammation and nerve injury could alter the synaptic efficacy and induce central sensitization in the dorsal horn neurons and is considered a fundamental mechanism underlying the induction and maintenance of chronic pain. This central sensitization takes a number of different and distinct forms.
One form of central sensitization is wind-up of dorsal horn neurons, an activity-dependent progressive increase in the response of neurons over the course of a train of inputs. Repetitive discharge of primary afferent nociceptors results in a co-release with glutamate of peptidergic neuromodulators such as substance P and CGRP from the nociceptor central terminals in dorsal horn. Temporal summation of these peptide-mediated slow excitatory postsynaptic potentials (EPSPs) may activate NMDA receptor, by removing Mg2+ suppression of the channel, and increase the excitability of dorsal horn neurons. A behavioral correlate of wind-up can be produced in humans by repeated peripheral noxious heat or mechanical stimuli, where the pain increases with each successive stimulus even though the stimulus intensity does not change.8 After peripheral nerve injury, light touch can produce pain (allodynia) and repeated light touch can produce progressively increasing pain (summation).
The second form of central sensitization is a heterosynaptic activity-dependent plasticity that outlasts the initiating stimulus for tens of minutes. After the induction of this form of activity-dependent central sensitization by a brief (as short as 10 to 20 seconds), intense nociceptor-conditioning stimulus, normally, subliminal/subthreshold inputs begin to activate dorsal horn neurons as a result of an increase in synaptic efficacy. This NMDA receptor-mediated increase of synaptic efficacy occurs not only in those nociceptor central terminal synapses activated by the conditioning or initiating stimulus but also the synapses not activated by the conditioning or initiating stimulus (Fig. 6-5). For example, low-threshold sensory fibers activated by innocuous stimuli such as light touch can, after the induction of the heterosynaptic central sensitization, activate normally high–threshold nociceptive neurons, producing allodynia.

Other forms of central sensitization include long-term potentiation, transcription-dependent central sensitization, loss of inhibition, and rearrangement of synaptic contacts. The former refers to the fact that brief duration, high-frequency primary afferent stimulation does induce a potentiation of AMPA receptor–mediated responses at homosynapses on to second-order neurons. Peripheral noxious stimuli may produce transcriptional changes of several proteins critically involved in pain transmission (e.g., brain-derived neurotrophic factor [BDNF] and cytokines) in primary sensory and dorsal horn neurons, altering their function and facilitating pain transmission for prolonged periods. Activation of Aδ primary afferents may also induce long-term depression of transmission at primary afferent synapses on to inhibitory dorsal horn neurons, contributing to the augmentation of nociceptive information. Following a lesion to a peripheral nerve, the central axons of injured myelinated Aβ fibers sprout from their normal termination site in the deeper laminae (laminae II and IV) into lamina II of the dorsal horn, contributing to nerve injury–induced tactile allodynia. It is notable that accumulating evidence suggests that a critical role is played by microglia-mediated neuroinflammation in the dorsal horn plasticity that leads to neuropathic pain.9
Ascending Pathway for Pain Transmission
Ascending pathways from the spinal cord to sites in the brainstem and thalamus are important for the perception and integration of nociceptive information. The major ascending pathways important for pain include the spinothalamic tract (STT, direct projections to the thalamus), spinomedullary and spinobulbar projections (direct projections to homeostatic control regions in the medulla and brainstem), and spinohypothalamic tract (SHT, direct projections to the hypothalamus and ventral forebrain). Some indirect projections, such as the dorsal column system and the spinocervicothalamic pathway, also exist to forward nociceptive information to the forebrain through the brainstem. Similar pathways originating from the medulla trigeminal sensory nuclei also exist to process the nociceptive information from the facial structures.
Among these pathways, the STT is the most closely associated with pain, temperature, and itch sensation. Retrograde tracing studies demonstrate that the fibers traveling in the STT originate in the spinal dorsal horn neurons in lamina I (receiving input from small-diameter Aδ and C primary afferent fibers), laminae IV and V (receiving input primarily from large-diameter Aβ fibers from skin), and laminae VII and VIII (receiving convergent input from large-diameter skin and muscle, joint inputs). About 85% to 90% of neuronal cells with projections extending through the STT are found on the contralateral side, with 10% to 15% on the ipsilateral side. The axons of STT cells generally cross in the dorsal and ventral spinal commissures to reach the white matter of the contralateral spinal cord within one or two segments rostral to the cells of origin. The lateral STT originates predominantly from lamina I cells, and the anterior STT originates from deeper laminae V and VII cells. In the lateral STT, the axons from caudal body regions tend to be located more laterally (i.e., superficially) in the white matter, whereas those from rostral body regions are located more medially (closer to midline). The axons of STT terminate in several distinct regions of the thalamus.
Spinobulbar projections originate from similar neurons as those in the STT (i.e., laminae I, V, and VII in the spinal dorsal horn). Spinal projections to the medulla are bilateral, and those to the pons and mesencephalon have a contralateral dominance. Ascending spinobulbar projections terminate mainly in four major areas of the brainstem, including the regions of catecholamine cell groups (A1–A7), the parabrachial nucleus, PAG, and the brainstem reticular formation. Spinal projections to the brainstem are important for the integration of nociceptive activity with processes that subserve homeostasis and behavior.
The spinohypothalamic tract (SHT) originates bilaterally from cells in laminae I, V, VII, and X over the entire length of the spinal cord. The SHT axons often have connections with the contralateral diencephalon, decussate in the optic chiasm, and then descend ipsilaterally through the hypothalamus and as far as the brainstem. The SHT appears to be important for autonomic, neuroendocrine, and emotional aspects of pain.
Supraspinal Modulation of Nociception
Several brain areas have been recently defined using human brain imaging studies as key supraspinal regions involved in nociceptive perception. The most commonly activated regions during acute and chronic pain include SI, SII, anterior cingulate cortex (ACC), insular cortex (IC), prefrontal cortex, thalamus, and cerebellum (see Fig. 6-2). These brain regions form a cortical and subcortical network, which are critically involved in the formation of emotional aspects of pain and the central modulation of pain perception.
In primates, SI and SII receive noxious and innocuous somatosensory input from somatosensory thalamus.10 Cingulate cortex receives input from medial thalamic nuclei that contain nociceptive neurons, including nucleus parafascicularis and the ventrocaudal part of nucleus medialis dorsalis, as well as from lateral thalamic regions. The IC also receives direct thalamocortical nociceptive input in the primate. Prefrontal cortical regions are activated in a number of imaging studies of acute pain in normal subjects, but these activations are not as common as those in the other cortical regions described earlier. The prefrontal cortex receives input from ACC, but there is no evidence that it receives direct thalamocortical nociceptive input. Several nuclei in the thalamus receive nociceptive input from the dorsal horn, and the cerebellum also has reciprocal spinal connectivity. Activation of the hypothalamus during acute and chronic pain is likely mediated by direct spinohypothalamic projections. Other subcortical regions, such as the striatum, nucleus accumbens, amygdala, hypothalamus, and PAG are also reported to be active in human pain imaging studies.
In general, somatosensory cortices (e.g., SI and SII) are more important for the perception of sensory features (e.g., the location and intensity of pain), whereas limbic and paralimbic regions (e.g., ACC and IC) are more important for the emotional and motivational aspects of pain.11 Anesthetized humans, without conscious awareness of pain, still exhibit significant pain-evoked cerebellar activation, suggesting that pain-evoked cerebellar activity may be more important in regulation of afferent nociceptive activity than in the perception of pain.
Descending Pathways of Pain Modulation
The relationship between reported pain intensity and the peripheral stimulus that evokes the pain sensation depends on a host of variables, including the presence of other somatic stimuli and psychological factors such as arousal, attention, and expectation. Certain central mechanisms also exist to either impede or enhance the centripetal passage of nociceptive messages.12 Evidence demonstrates that descending pathways originating from certain supraspinal regions may concurrently promote and suppress nociceptive transmission through the dorsal horn, termed the descending inhibition pathway (DI) and the descending facilitation pathway (DF).12 Notably, there is no absolute, anatomic separation of substrates subserving these processes, and the stimulation of a single supraspinal structure may, via divergent actions of diverse transmitters and different receptor types, simultaneously trigger both DI and DF.
Electrical stimulation of the PAG and more rostral periventricular structures inhibits activity of nociceptive dorsal horn neurons and noxious stimulus-evoked reflexes and induces stimulation-evoked analgesia in rodents and humans. This establishes the PAG and the RVM regions of the brainstem as the critical brain regions underlying descending pain modulation (Fig. 6-6). PAG neurons receive direct or indirect inputs from several brain structures, including the amygdala, nucleus accumbens, hypothalamus and others, with ascending nociceptive afferents from the dorsal horn. The RVM includes the midline nucleus raphe magnus and the adjacent reticular formation that lies ventral to the nucleus reticularis gigantocellularis. The PAG and the adjacent nucleus cuneiformis are the major source of inputs to the RVM. The RVM receives input from serotonin-containing neurons of the dorsal raphe and neurotensinergic neurons of the PAG. The PAG–RVM connection is critical for pain modulation. The PAG projects only minimally to the spinal cord dorsal horn, and the pain-modulating action of the PAG on the spinal cord is relayed largely, if not exclusively, through the RVM.

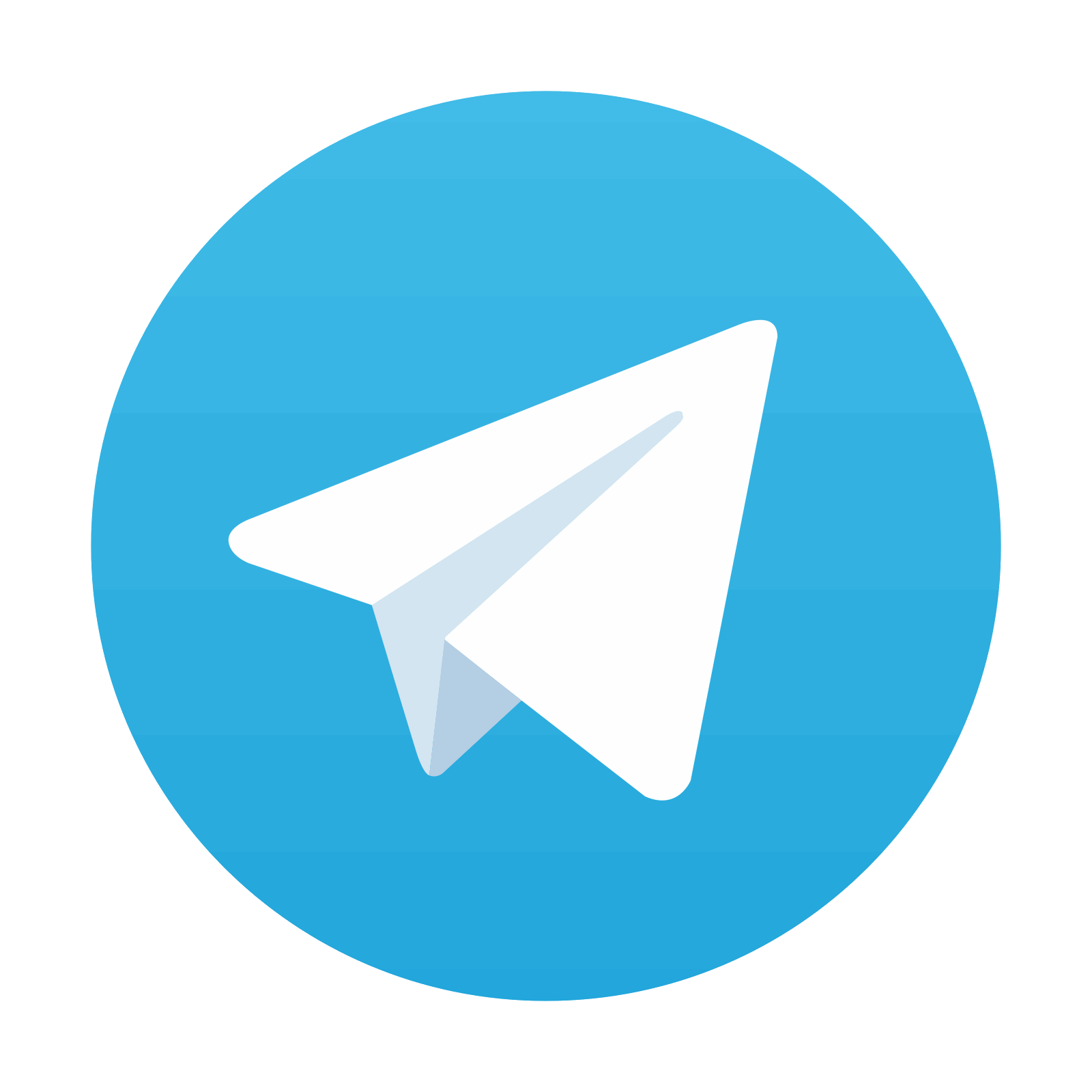
Stay updated, free articles. Join our Telegram channel

Full access? Get Clinical Tree
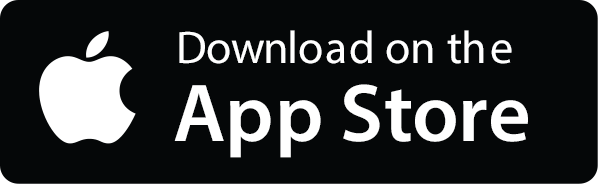
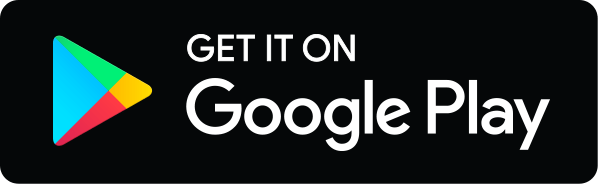