Factor
Effect
Low-threshold A-fiber mechanoreceptors terminate centrally on nociceptive relay pathways
Weaker stimuli activate pain specific pathways
Weak intrinsic inhibitory mechanisms in spinal cord
Relative augmentation of pain signal
Reduced descending inhibition
Relative augmentation of pain signal
Large and overlapping cutaneous receptive fields
Amplification of stimulus effect due to increased numbers of neurons activated
Poorly localized and diffuse sensory-motor connexions
Less anatomically specific and more generalized motor responses

Fig 14.1
(a) Adult sensory inputs. (b) Neonatal sensory inputs
In early development, these central terminals are relatively less well localized, and those of low-threshold A-fibers overlap with C-fiber terminals in lamina II (Fig 14.1b), thereby potentially activating nociceptive projection neurons when stimulated. A reduction in specificity due to this structural difference is augmented by lack of myelination and immature ion channel kinetics that alter neuronal conduction times and synaptic strength leading to a more diffuse central response to peripheral stimuli. Intrinsic spinal cord and descending inhibitory controls are also less well organized and reduced in strength. In contrast to the adult, contralateral cutaneous inhibitory receptive fields are not matched to their corresponding excitatory fields [6]. Cutaneous receptive fields, the area of skin that excites an individual sensory neuron when stimulated, are relatively larger and more overlapping at birth such that each stimulus is cable of inducing a response in many more neurons at this time [9]. This lack of specificity, organization, and control is mirrored in motor circuits such that output responses are also more diffuse and less well integrated spatially and temporally [6]. Although little is currently known about nociceptive processing in higher centers of the brain, physiological studies in premature neonates have demonstrated that painful inputs are capable of producing measurable responses from at least 24 weeks postconception [10].
Sensitization, Inflammatory and Neuropathic Pain
The decrease in sensory thresholds that develop at the site of an injury is known as primary hyperalgesia; it is accompanied by a temporary reduction in thresholds both in the surrounding non-injured tissue and at distant sites known as secondary hyperalgesia. These post injury changes in sensitivity are characteristic of inflammatory pain, a normal part of the healing process. This pain responds fairly well to analgesics and will usually resolve spontaneously as the injury resolves. The processes responsible are known as sensitization, a phenomenon that involves many different mechanisms both in the periphery and CNS [11]. If damage occurs to nerves or nervous tissue, a state of more prolonged pain that is known as neuropathic pain may follow. Although neuropathic pain also involves sensitization, unlike inflammatory pain, it often does not resolve spontaneously and is often difficult to treat. Neuropathic pain is a component of many chronically painful conditions such as phantom limb pain, diabetic neuropathy, trigeminal neuralgia, complex regional pain syndrome (CRPS) and many others. Both sensitization and the mechanisms underlying neuropathic pain are under intense research scrutiny with the aim of finding more effective analgesics [12].
These responses are different in neonates. Primary hyperalgesia at the site of an injury is known to occur from birth. Although recovery seems to be more rapid in neonates, a more prolonged inflammatory hyperalgesia has also been demonstrated after repetitive injury [13]. Secondary hyperalgesia appears to be less prominent at younger ages and is slower to develop [14]. Neuropathic pain has not been reported in neonates or infants, even after severe nerve injuries that represent a potent cause of pain in adults, such as brachial plexus damage [15]. Laboratory investigations have confirmed that nerve damage does not produce signs of neuropathic pain in either the neonatal or infant period. Recent studies have suggested that this may be due to immaturity of immune responses in the spinal cord involving microglia and peripheral cellular immunity known to maintain neuropathic pain in adults [16–18].
Long-Term Effects of Pain and Analgesia in the Neonatal Period
There is also evidence that pain in the neonatal period can lead to augmented responses to pain some time later. Boys who underwent neonatal circumcision without analgesia showed an enhanced response to pain at 3 months during immunization in comparison with those who did receive analgesia or were not circumcised [19]. Infants who had abdominal surgery repeated in the same dermatome as a previous operation before 3 months of age showed increased pain responses and analgesic requirements compared with controls [7]. Even more “minor” events such as heel stick blood sampling are a significant cause of pain in the neonate [20]. They too can lead to augmentation of the response to subsequent pain or may even be associated with more serious morbidity and poorer outcomes, especially when repeated frequently, e.g., in ICU [13, 21]. More complex and subtle effects have also been shown in cohorts of children who had neonatal surgery and ICU admission. A relative increase in temperature and touch thresholds near the site of surgery has been observed, but some children also have a more generalized decrease in temperature threshold [8, 22, 23]. Although the precise mechanisms behind these observations are not known, it is clear that sensory development depends on a normal balance of sensory activity, and if this is disrupted, then abnormal patterns or even failure of normal maturation can occur. In the laboratory, maturation of nociceptive reflexes can be delayed or abolished by blocking sensory inputs with local anesthesia for long periods [24]. NMDA receptor activity has been shown to be important for normal sensory development in rat pups as chronic NMDA receptor blockade prevents the normal withdrawal of A-fibers from lamina II described above and a consequent persistence of low sensory thresholds [25].
A panoply of other physiologic sequelae have been reported after painful stimulation in premature infants. During the early brain growth period in premature infants, repetitive painful procedures have been shown to reduce both white matter and subcortical gray matter, leading to impaired brain development [26]. A growing body of evidence has associated repetitive painful procedures in the early postnatal period in premature infants with reduced weight gain and increased head circumference in the early postnatal period [27].
A number of drugs and chemical compounds may also cause long-term adverse effects when administered in the neonatal period over and above altered pharmacokinetic or pharmacodynamic responses due to immaturity. Neuroapoptosis, or programmed cell death, is a component of normal maturation in which cells that do not form functional connexions are eliminated. Drugs that are NMDA antagonists and/or GABA agonists in particular have the potential to markedly increase apoptosis to such an extent that neural development is damaged leading to deficits in, e.g., memory and learning. Although these effects have only been demonstrated in animal models to date, many general anesthetics have been implicated including ketamine (see below), a potent non specific NMDA antagonist that is also used as an analgesic.
These many factors impact the assessment, measurement and management of neonatal pain such that considerable specialist knowledge and skills are needed in order to deliver developmentally appropriate care.
Assessment of Pain
Frequent assessment of pain is an essential component of good pain management; however, this can be problematic in immature, preverbal infants. Accurate assessment including measurement of pain intensity contributes to the prevention or early recognition of pain, as well as for monitoring the effectiveness of analgesia [28]. Overall there are three fundamental approaches to pain assessment in children:
Self-report: an individual’s personal description of pain and rating of intensity
Behavioral: observation of changes in facial expression and body posture due to pain
Physiological: measurement of changes in physiological arousal consequent to pain
Obviously, self-report is impossible in neonates, and therefore one of the indirect measures of pain must be used. This is associated with disadvantages; perceived pain intensity is known to depend on many subjective influences apart from the degree of injury and tissue damage. Stress, anxiety, attention, and expectation, which are modulated by context, mood, previous experience, and underlying personality traits all contribute to the degree of unpleasantness of pain; the extent to which such factors can influence pain perception in the neonate is largely therefore a matter of speculation.
Nevertheless, in neonates, the observation of behaviors such as facial expression, cry, and posture and measurements of physiological variables such as heart rate and blood pressure have been used to assess pain and gauge its intensity in the absence of viable more objective alternatives. These observations and measures are subject to many external and internal influences aside from pain, which leads to difficulties in interpretation. For physiological variables in particular, a reduction in their reliability tends to occur over time due to homeostatic controls. In an attempt to improve accuracy, observations and measurements have been frequently incorporated into multidimensional pain measurement “tools” or “instruments” that are generally presented as checklists or scoring systems; the range of such observations and their validity and usability have been reviewed recently [29–32].
Pain Measurement Tools
A bewilderingly large number of pain assessment tools or scales have been designed for use in the neonate, some examples are given in Table 14.2 [1]. There is a considerable research literature on the subject, and it is now agreed that in order to be “fit for purpose”, a pain assessment tool should have undergone a rigorous process of development.
Table 14.2
Pain measurement tools
BPS [33], behavioral pain score |
CHIPPS [34], children and infants postoperative pain scale |
CRIES [37] |
CSS [38], clinical scoring system |
DSVNI [39], distress scale for ventilated newborn infants |
LIDS [40], Liverpool infant distress scale |
NFCS [41], neonatal facial coding system |
NIPS [42], neonatal infant pain scale |
PAT [43], pain assessment tool |
PIPP [44], premature infant pain profile |
SUN [45], scale for use in newborns |
To be considered reliable, an individual tool must be validated in the patient population and the clinical context and type of pain (e.g., postoperative or procedural) for which it is to be used. Despite the proliferation and availability of tools, they have not always adequately completed this process nor been used consistently or well; inconsistencies have been identified between reported assessment practice and documented practice [46–48]. Several factors may be responsible for this situation including the large number of scales that are available, limitations to individual scales that mean no single one can be universally recommended for use in all neonates in every situation, and “usability” factors that lead to individual user preferences that might not be scientifically appropriate.
Given the difficulty in finding the “Holy Grail” of behavioral pain scales for neonates, investigators have begun to pursue objective physiologic tools [1]. These pursuits have included regional oxygen saturation in the brain (as in near-infrared spectroscopy), EEG, heart rate variability, skin conductance and neurohumoral responses. Although each appears to be an objective metric that may reflect the neonate’s response to a painful stimulus, some of the metrics under investigation are invasive, others reflect a time course that may not correspond to the level of stimulation, and others remain imprecise. This remains an active work in progress that may require an aggregate of different measurements to provide a reliable and consistent metric of pain in the neonate.
Selecting an Appropriate Pain Assessment Tool
Recommendations and guidelines have been produced by a number of professional bodies outlining the currently available tools and advising on their suitability for different circumstances [29, 31, 32]. Training and support are required for successful implementation of the best validated tools, and this should be combined with ongoing monitoring and audits of practice. Three of the most widely endorsed tools are the PIPP [44], CRIES [37], and COMFORT [35] scales. The PIPP (Table 14.3) creates a score from 18 to 21 depending on gestational age and behavioral state, with 0–6 reflecting no pain, 6–12 reflecting mild to moderate pain and above 12 indicating severe pain; it is suitable for procedural pain and ongoing postoperative pain. CRIES includes similar indicators to PIPP: crying, oxygen requirements, increases in heart rate or blood pressure, facial expression and sleep behavior. CRIES creates a score from 0 to 10, similar to most self-report or observational measures of pain. The COMFORT [36] tool is more complex, originally developed in 1992 as an assessment of global comfort in pediatric intensive care. Since that time it has undergone a number of validation studies for both procedural and ongoing postoperative pain in intensive care. It is frequently chosen for use in the sickest neonates, e.g., after cardiac surgery.
Gestational age | |
≥36 weeks | 0 |
32 weeks to 35 weeks 6 days | 1 |
28 weeks to 31 weeks 6 days | 2 |
<28 weeks | 3 |
Behavioral state | |
Active/awake eyes open facial movements | 0 |
Quiet/awake eyes open no facial movements | 1 |
Active/sleep eyes closed facial movements | 2 |
Quiet/sleep eyes closed no facial movements | 3 |
Heart rate maximum | |
0–4 beats per minute increase | 0 |
5–14 beats per minute increase | 1 |
15–24 beats per minute increase | 2 |
≥25 beats per minute increase | 3 |
Oxygen saturation minimum | |
0–2.4 % decrease | 0 |
2.5–4.9 % decrease | 1 |
5.0–7.4 % decrease | 2 |
7.5 % decrease or more | 3 |
Brow bulge | |
None (≤9 % of time) | 0 |
Minimum (10–39 % of time) | 1 |
Moderate (40–69 % of time) | 2 |
Maximum (> = 70 % of time) | 3 |
Eye squeeze | |
None (≤ 9 % of time) | 0 |
Minimum (10–39 % of time) | 1 |
Moderate (40–69 % of time) | 2 |
Maximum ≥70 % of time) | 3 |
Nasolabial furrow | |
None (≤9 % of time) | 0 |
Minimum (10–39 % of time) | 1 |
Moderate (40–69 % of time) | 2 |
Maximum (≥70 % of time) | 3 |
Score total (0–21) |
Planning and Organizing Pain Management
Multimodal or Balanced Analgesia
Current strategies for the treatment of acute pain are centered on the concept of multimodal analgesia, which was first proposed in order to increase the efficacy of analgesics while reducing their adverse effects [49]. The supporting rationale is that the major pharmacological groups of analgesics act on different components of pain pathways and as such their effects are likely to be complementary. This is also likely to be true in the neonate, but developmental factors influencing the effects and therefore appropriateness of many analgesics must also be considered. It is logical to use combinations of analgesics, such as acetaminophen, opioids, and local anesthetics in conjunction in order to achieve the optimum effect while keeping the dose of each, and therefore side effects, at a moderate level. Sucrose and non-pharmacological pain management strategies such as nonnutritive sucking (NNS), swaddling, massage, etc. also have an important place in neonatal pain management, particularly for procedural pain, and should therefore be included in a multimodal regimen where it is appropriate.
Information and Protocols: Pain Management Plans
The provision of training and education for healthcare workers and availability of written and verbal information for families and carers are pivotal for successful pain management. Analgesic regimens should be pre-planned wherever possible and implemented with supporting educational programs, provision and maintenance of necessary equipment and clear developmentally appropriate management protocols. Pain management protocols must be sufficiently flexible to allow for differences in analgesic requirements due to developmental age and other factors; they should include a pain assessment and reassessment plan, encompass management of background and incident (breakthrough) pain and stipulate monitoring and management of adverse effects.
A well-designed protocol will therefore ensure efficacy and uniformity of treatment and facilitate ongoing evaluation of effectiveness. Protocols for pain management should also be designed in conjunction with ongoing global management strategies such as family-centered and developmental care [50, 51]. The implementation of family-centered care involves the establishment of a partnership between parents or carers and nursing staff and other healthcare workers that substantially increases parents’ role in their child’s in-hospital care. Developmental care in NICU is an increasingly popular strategy for reducing stress-related morbidity in premature neonates; stressful and painful inputs are reduced by observing responses on an individualised basis and carefully reorganizing and planning care [52].
Developmental Pharmacology of Analgesics
Relatively few analgesics have a clearly established role in neonatal pain management. Detailed analgesic clinical pharmacology is discussed in other chapters.
Acetaminophen (Paracetamol)
Acetaminophen is an antipyretic and mild analgesic that has been widely used for all ages, including premature neonates. Acetaminophen is used for the management of pain of mild to moderate severity; more severe pain is not controlled by acetaminophen alone. It is often combined with more potent analgesics for postoperative pain after major surgery in neonates, with conflicting results: one study showed significant morphine-sparing effect of intravenous paracetamol [53], whereas another showed no additional effect of rectal acetaminophen when combined with morphine [54].
The precise mechanism of action of acetaminophen is unknown, but central cyclooxygenase (COX) inhibition is probably important; other mechanisms have also been proposed including NMDA and serotonin antagonism and a possible action on cannabinoid receptors [55–57]. Alterations in the pharmacokinetic handling of acetaminophen have significant implications for safe dosing in neonates. Gastrointestinal absorption is delayed in premature neonates, whereas rectal bioavailability is initially greater in the premature and then decreases toward the usual value of 0.5 with increasing age [58]. The volume of distribution decreases and clearance increases from 28 weeks postconceptional age, resulting in a gradual decrease in the elimination half-life.
Acetaminophen is metabolized via both sulfation and glucuronidation, and the increased maturity of the sulfation pathway early in development and relatively high levels of glutathione at this time may provide some “protection” against toxicity in neonates [59]. However, as many kinetic studies have investigated single-dose administration only, caution is warranted with repeated dosing for more than two or three days [59]. Increased production of the reactive product N-acetyl-p-benzoquinoneimine occurs leading to liver toxicity if the usual metabolic enzyme systems become saturated due to overdose or if glutathione is depleted (e.g., with prolonged fasting).
Dose guidelines based on formulation, route of administration, weight and developmental age have been determined by pooled population analysis (Tables 14.4 and 14.5). Antipyretic plasma levels are 10–20 mg/l, levels required for analgesia are thought to be similar, and so most dosing regimens aim to maintain trough plasma concentrations of 10 mg/l [61]. A greater initial dose followed by maintenance doses not exceeding recommended maximum daily doses is generally recommended. Peak plasma levels are rapidly achieved after oral ingestion, but there is a 1–2 h lag before the maximum therapeutic effect; the onset of analgesia after IV administration may be much faster [62]. As rectal bioavailability is much reduced and more variable than oral bioavailability, greater initial doses are recommended when this route is used except in the premature infant. Two intravenous preparations of acetaminophen are available: IV acetaminophen and propacetamol. Propacetamol is a prodrug, which is hydrolyzed to 50 % acetaminophen and is therefore administered in twice the dose of the native drug, i.e., 1 g propacetamol is equivalent to 500 mg acetaminophen. This is a potential source of confusion and error [60]. The clearance of propacetamol is reduced in infants less than 1 year of age, thus reducing the maintenance doses [63]. Histamine release, pain on injection and contact dermatitis in healthcare workers have been reported with propacetamol. Additionally, mild platelet dysfunction has been reported [64, 65]. Intravenous acetaminophen appears to be devoid of these drawbacks, and therefore it has gained widespread acceptance in pediatric practice.
Table 14.4
Acetaminophen dosing guide—oral and rectal administration
Age | Route | Loading dose | Maintenance dose | Interval | Maximum daily dose | Duration at maximum dose |
---|---|---|---|---|---|---|
28–32 weeks PCA | Oral | 20 mg/kg | 10–15 mg/kg | 8–12 h | 30 mg/kg | 48 h |
Rectal | 20 mg/kg | 15 mg/kg | 12 h | |||
32–52 weeks PCA | Oral | 20 mg/kg | 10–15 mg/kg | 6–8 h | 60 mg/kg | 48 h |
Rectal | 30 mg/kg | 20 mg/kg | 8 h | |||
>3 months | Oral | 20 mg/kg | 15 mg/kg | 4 h | 90 mg/kg | 72 h |
Rectal | 40 mg/kg | 20 mg/kg | 6 h |
Table 14.5
Intravenous acetaminophen/propacetamol dosing guidea
Age | Drug | Loading dose (mg/kg) | Maintenance dose (mg/kg) | Dose interval | Maximum daily dose (mg/kg) |
---|---|---|---|---|---|
<32 weeks PCA | Propacetamol | 40 | 20 | 12 h | 60 |
Acetaminophen | 20 | 10 | 12 h | 30 | |
32–36 weeks PCA | Propacetamol | 40 | 20 | 8 h | 80 |
Acetaminophen | 20 | 10 | 8 h | 40 | |
36–52 weeks PCA | Propacetamol | 40 | 20 | 6 h | 100 |
Acetaminophen | 20 | 10 | 6 h | 50 | |
>1month | Propacetamol | 30 | 30 | 6 h | 120 |
Acetaminophen | 15 | 15 | 6 h | 60 |
Several cases of massive overdose of IV paracetamol have been reported in preterm neonates and young infants to date [66–68]. In all instances, full recovery occurred without long-term sequelae. These did not appear to be the result of confusion of paracetamol with propacetamol. Recognizing the risk for potential liver failure or death from an iatrogenic overdose of acetaminophen in a neonate should prompt every institution to implement very tight controls on the dose of paracetamol when it is prescribed for neonates.
Nonsteroidal Anti-inflammatory Drugs
Nonsteroidal Anti-inflammatory Drugs (NSAIDs) are not currently used in neonates for analgesia due to the uncertainty regarding their efficacy, and potential for adverse effects results in an unfavorable benefit-risk ratio. They act by inhibition of cyclooxygenase, enzymes that regulate many cellular functions by the production of prostaglandins and other substances. Prostaglandins have multiple roles in early development, and inhibition of their synthesis with NSAIDs may potentially result in the disruption of the sleep cycle, an increased risk of pulmonary hypertension, alterations in cerebral blood flow, decreased organ perfusion and renal function and disrupted thermoregulation [69].
In premature neonates in intensive care, prophylactic intravenous indomethacin reduces both the need for surgical ligation of patent ductus arteriosus and the incidence of grades 3 and 4 intraventricular hemorrhage. Reductions in cerebral, renal, and mesenteric blood flow velocity occur for 2 h after bolus indomethacin, but can be minimized by continuous infusion. Renal effects are also less with the use of ibuprofen compared with indomethacin. There is therefore a potential to reduce NSAID-related adverse effects. However, laboratory studies have shown a reduced efficacy of NSAIDs in young rodents, casting doubt on their value as analgesics in infants [70].
Opioids
Morphine is the prototypic opioid, having been extensively investigated in the neonate and used to treat severe acute pain after surgery and in the ICU. Dose requirements and clinical responses to opioid agents differ markedly between premature and term neonates and infants and children. Multiple factors contribute to these differences including age-dependent alteration in body composition and organ function influencing opioid pharmacokinetics and genetic and developmental factors that change opioid pharmacodynamics. Therefore, regular pain assessment with individual titration and adjustment of doses according to response is required to achieve analgesia and minimize adverse effects. Tolerance leading to dose escalation and subsequent physical withdrawal response if opioid infusion rates are reduced too rapidly are distressingly frequent problems after medium- to long-term use in intensive care [71]. Other more lipophilic opioids such as hydromorphone, fentanyl, and remifentanil are also sometimes chosen for acute pain management in neonates and are therefore discussed below along with tramadol and the morphine prodrug, codeine.
Morphine
Morphine can be given orally or parenterally. Morphine solutions are generally well absorbed orally, but the pharmacokinetics and efficacy of oral opioids have not been clearly established in neonates. Oral morphine can be give at a dose of 0.2 mg/kg every 4 to 6 h in monitored non-ventilated neonates (Table 14.6). Parenteral morphine is usually given intravenously either by intermittent dosing, continuous infusion or in a nurse-controlled analgesia (NCA) regimen (Tables 14.5 and 14.6) [72]. Subcutaneous morphine is also used. The pharmacokinetics and clinical use of morphine in neonates have been reviewed extensively [73–76]. The pharmacokinetics of IV morphine are developmentally regulated. In the neonatal period, the pharmacokinetics are characterized by high inter-patient variability and reduced clearance, rendering the clinical effects of morphine less predictable than in older children. In neonates 1–7 days of age, the clearance of morphine is markedly diminished, being 30 % of that of older infants and children. As a result, the elimination half-life is approximately 1.7-fold greater than in older children [76, 77]. Infusion rates and dose intervals must therefore be adjusted according to both age and weight of the neonate, to avoid accumulation. Although the plasma levels associated with analgesia are not well defined, a mean steady-state plasma concentration of 10 ng ml−1 is a reasonable target in the neonate. This level may be achieved in children in intensive care after noncardiac surgery with a morphine hydrochloride infusion of 5 mcg h−1 kg−1 at birth (term neonates), 8.5 mcg h−1 kg−1 at 1 month, 13.5 mcg h−1 kg−1 at 3 months, 18 mcg h−1 kg−1 at 1 year and 16 mcg h−1 kg−1 for 1- to 3-years-old children [75]. A recent retrospective audit of morphine consumption over a wide age range by the same investigators indicated that morphine infusion rates of 10 mcg h−1 kg−1 appear appropriate for neonates and infants 1–6 months of age, but given the interindividual variability in responses, the rate should be adjusted to the infant’s pain level, concomitant medications, and physiological responses [78]. Conversely, a common threshold for respiratory depression in neonates, infants, and children has been defined as 20 ng ml−1 [79]. Any differences in efficacy observed between continuous infusion and intermittent boluses of morphine probably relate more to the age appropriate total dose of drug received, rather than the route of administration [80, 81] Sedation and respiratory depression are the most frequently reported adverse events after morphine (and other opioids) [82] administration. Adverse effects of morphine can be reversed by administering the opioid antagonist naloxone A timely administration of naloxone should facilitate complete recovery from the adverse effects.
Table 14.6
Morphine dosing and morphine infusion
Morphine dosing | |
---|---|
Preparation | |
Oral solution | 200 mcg/kg, 4–6 hourly |
Intravenous | 25–50 mcg/kg initial dose (titrated according to response) |
25 mcg/kg every 30 min–1h | |
Morphine infusion | |
Preparation | Morphine sulfate 1 mg/kg in 50 ml solution |
Concentration | 20 mcg/kg/ml (0.02 mg/kg/ml) |
Initial dose | 0.5–2.5 ml (0.01–0.05 mg/kg) |
Infusion rate | 0.1–0.6 ml/h (2–12mcg/kg/h) |
Box 1 Nurse-Controlled Analgesia (NCA)
NCA is a demand-led alternative for patients who are too young or unable to use PCA (patient-controlled analgesia). It is designed to provide safe, potent, flexible and convenient pain control by combining the possibility of a continuous opioid analgesic infusion with on-demand bolus doses of analgesia administered according to predetermined limits. NCA was first developed for infants and those children and adults who were unable to operate the PCA handset and was subsequently adapted for neonates [72]. The protocol for the initial infusion of NCA in a postsurgical neonate is shown in Table 14.7.
Table 14.7
NCA (morphine) protocol for neonates and infants
NCAa for neonatal use | |
---|---|
Preparation | Morphine sulfate 1 mg/kg in 50 ml solution |
Concentration | 0.02 mg/kg/ml |
Initial dose | 0.5–2.5 ml (0.01–0.05 mg/kg) |
Pump programming | |
Background infusion | 0–0.5 ml (0–0.01 mg/kg/h) |
NCA dose | 0.5–1.0 ml (0.01–0.02 mg/kg) |
Lockout interval | 20 or 30 min |
Fentanyl
Fentanyl is a synthetic, high-potency (100x morphine) lipid-soluble opioid; its main use is for intraoperative analgesia where its rapid onset, short initial half-life, and cardiovascular stability at larger doses are an advantage. Fentanyl is also used by infusion in ICU, and it has some advantages for procedural pain owing to its rapid onset. Unfortunately it also creates the potential to more rapidly develop tolerance after prolonged use and may cause opioid withdrawal syndromes.
After a single intravenous dose, the duration of action of fentanyl is 30–45 min. Given its high lipid solubility, the pharmacokinetic profile of fentanyl is context sensitive, such that its half-life progressively increases with the duration of the infusion [83]. High-dose fentanyl has been associated with chest wall rigidity and subsequent difficulty in ventilation. Accordingly, large doses are usually given only when respiration is controlled [84]. Fentanyl can also be given neuraxially; in the epidural space, it is used alone or in combination with an infusion of local anesthetic after major surgery [85, 86]. Alfentanil and sufentanil are fentanyl analogs with different potencies and durations of effect. Their principal use is during anesthesia, but they have also been used for postoperative pain and pain due to brief procedures particularly in the ICU [87, 88]. Sufentanil is more potent, but otherwise very similar to fentanyl in its clinical effect. It has been administered by infusion in the ICU, but probably does not offer significant advantage. Alfentanil is less potent than fentanyl. Its pharmacokinetics have been studied in the neonate. Its duration of action after a single dose is relatively brief, making it suitable for use during tracheal intubation [78]. However like fentanyl, doses effective for painful procedures can lead to chest wall rigidity in neonates and therefore should probably only be used if ventilation is controlled [89].
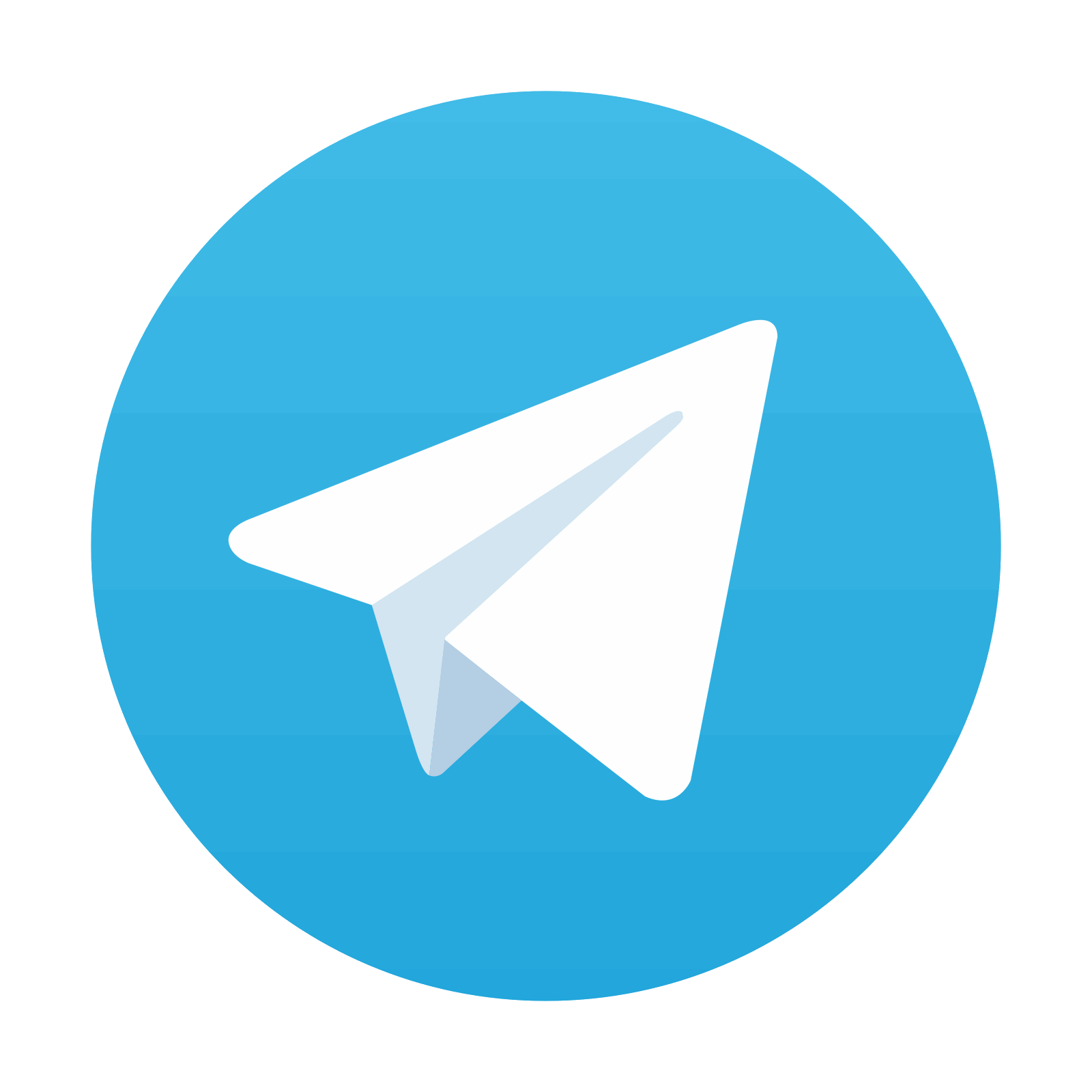
Stay updated, free articles. Join our Telegram channel

Full access? Get Clinical Tree
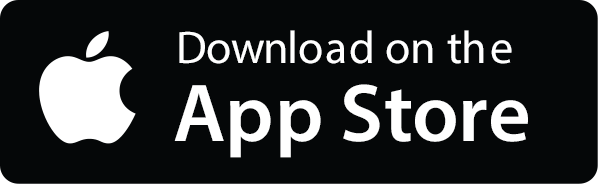
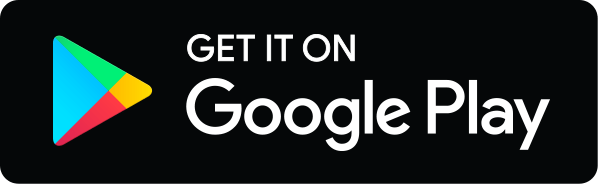