31 Otorhinolaryngologic Procedures
OTORHINOLARYNGOLOGIC PROCEDURES REPRESENT A large segment of elective pediatric surgery. Anesthetic management of these children is provided by both pediatric and general anesthesiologists, frequently working in anesthesia care teams, most commonly in ambulatory surgery centers and office practices.1 Additionally, anesthesiologists are often consulted to help manage potentially life-threatening pediatric otolaryngologic emergencies. These include airway obstruction suffering from croup, foreign body aspiration, airway trauma and acute epiglottitis.2 In both the elective and emergent scenarios, it is essential to understand the pathophysiology, and to discuss the anesthetic plan in advance of the procedure with the surgeon, who will frequently be sharing the airway with the anesthesiologist. This ensures safe anesthetic management and ideal conditions for both children and surgeons.
Anesthesia for Otologic Procedures
Myringotomy and Ventilating Tube Insertion
Myringotomy with tube insertion is a very brief operation, usually performed as ambulatory surgery using a potent inhalational agent (e.g., sevoflurane), oxygen, and nitrous oxide administered by facemask with spontaneous respirations. An oropharyngeal airway may assist in maintaining a patent airway when the head is laterally rotated and reduces head movement (which is amplified through the microscope). Gentle manual assistance of ventilation can also help reduce head movement. Occasionally, a laryngeal mask airway (LMA) may be used in children in whom the procedure is expected to be prolonged (e.g., children with narrow ear canals) or those with a difficult airway. Most children can be managed safely without intravenous (IV) access,3 but it is reasonable to have an IV setup ready. Some children with severe underlying medical or surgical conditions will require IV access, despite the anticipated brief duration of the minor procedure. Although premedication is often omitted because their duration of action exceeds that of the procedure, an anxious child may still benefit from a sedative premedication.
Discomfort after myringotomy and tube insertion is usually managed by the administration of acetaminophen, either via the oral route preoperatively or the rectal route intraoperatively. The recommended dose of acetaminophen to achieve therapeutic blood levels is 10 to 20 mg/kg when administered via the oral route, and 30 to 40 mg/kg when administered via the rectal route.4–7 Oral acetaminophen is very rapidly absorbed, achieving therapeutic blood levels in minutes, whereas rectal acetaminophen is slowly absorbed, with a time to onset of action of 60 to 90 minutes, and a time to peak effect of 1 to 3 hours.8–11 Consequently, the oral route is preferred for this procedure.
Preschool-aged children who receive sevoflurane without an analgesic for myringotomy and tube insertion may exhibit emergence delirium and postoperative agitation (see Chapter 4). Although pain may be partially responsible for these responses, their etiologies are not completely understood. Because the procedure is so brief and IV access is not usually established, intranasal fentanyl, 1 to 2 µg/kg, has been shown to provide analgesia and to reduce the frequency of emergence agitation.12,13 The only significant side effect is a 12% incidence of vomiting when oral fluids are administered in the early postoperative period.5 Other medications, including IV ketorolac (1 mg/kg), or intranasal butorphanol (25 µg/kg), and intranasal dexmedetomidine (1 to 2 µg/kg), have been shown to reduce the pain after myringotomy and tube insertion.14–16 However larger doses of dexmedetomidine (2 µg/kg) significantly prolong the duration of stay in the postanesthesia care unit (PACU). Some practitioners prefer to use more soluble anesthetics, such as isoflurane, for anesthesia maintenance to reduce the incidence of agitation after myringotomy and tube insertion, although there is limited evidence to support this practice.
Children with chronic otitis frequently have persistent rhinorrhea and suffer recurrent upper respiratory tract infection (URI) (see Chapter 11). Eradication of middle ear congestion and improved fluid drainage often resolves the concomitant symptoms. The frequency of perioperative complications in children with mild URIs is similar to that in children who are asymptomatic. In general, morbidity is not increased in children who present for minor surgery with acute uncomplicated mild URIs, provided tracheal intubation can be avoided.17,18 Canceling this surgery because of rhinorrhea or recurrent mild respiratory symptoms is not usually justifiable. It is, however, recommended that children with respiratory symptoms have their oxygen saturation measured before induction of general anesthesia, and that supplemental oxygen is administered postoperatively to those whose oxygen-saturation readings are less than 93%.19
Middle Ear and Mastoid Surgery
To gain access to the surgical site, the child’s head is placed on a headrest, which may be positioned below the operating table. In addition, extreme degrees of lateral rotation may be required to visualize the middle ear anatomy. The anesthesiologist and surgeon must be especially vigilant to ensure that nerves, muscles, and bony structures are not injured as a result of this unusual positioning; the sternocleidomastoid muscles generally limit the safe degree of lateral head rotation. Left or right tilting (airplaning) of the operating room (OR) table minimizes the need for extreme lateral head rotation as in the case of children with Down syndrome. The laxity of the ligaments of the cervical spine, as well as immaturity of the odontoid process in these children predisposes them to C1-C2 subluxation. Of children with Down syndrome or achondroplasia, 15% to 31% have atlantoaxial instability.20–23 Anteroposterior positioning requires the utmost care to avoid injury. Positioning of the OR table to allow access to the respective middle ear and accommodate all the extra surgical equipment can also pose a challenge. Depending on the room configuration, the table may be rotated 90 degrees or even 180 degrees away from the anesthesia machine, necessitating the use of extra-long breathing circuits (Fig. 31-1). As a result of the limited access to the airway, very careful attention must be paid to securing the tracheal tube. Draping must allow immediate access to the airway should that be required.
The middle ear and sinuses are air-filled, nondistensible cavities. An increase in the volume of gas within these cavities increases the pressure within the cavities. Nitrous oxide diffuses along a concentration gradient into air-filled middle ear spaces more rapidly than nitrogen moves out because nitrous oxide is 34 times more soluble in blood than nitrogen. The middle ear is vented through the opening of the eustachian tube. Normal passive venting of the eustachian tube occurs at 20 to 30 cm H2O pressure. Nitrous oxide increases the pressures within the middle ear such that they exceed the ability of the eustachian tube to vent the middle ear within 5 minutes, leading to pressure buildup.24 If the function of the eustachian tube is compromised during the surgical procedure, then pressure in the middle ear can increase further. Venting the middle ear occurs intermittently, and leads to constant fluctuations in middle ear pressure that cause movement of the tympanic membrane.25 During procedures in which the tympanic membrane is replaced or a perforation is patched, nitrous oxide should be discontinued or, if this is not possible, limited to a maximum of 50% of the concentration before the application of the tympanic membrane graft to reduce the potential for pressure-related displacement.26 The omission of nitrous oxide does not significantly increase the requirements (minimal alveolar concentration) for the less-soluble inhaled anesthetics, desflurane, or sevoflurane in children.27 After nitrous oxide is discontinued, it is quickly reabsorbed, creating a void in the middle ear, with resulting negative pressure. This negative pressure may result in serous otitis, disarticulation of the ossicles in the middle ear (especially the stapes), and hearing impairment, which may last up to 6 weeks postoperatively. The use of nitrous oxide may increase the incidence of postoperative nausea and vomiting (PONV), as a direct result of negative middle ear pressure during recovery. The negative pressure created by the reabsorption of nitrous oxide stimulates the vestibular system by producing traction on the round window. Although all children are at risk for PONV, older children and adolescents, in particular, seem to be at greatest risk.28 Prophylactic administration of antiemetics (e.g., dexamethasone and ondansetron) is usually warranted. Local infiltration of the great auricular nerve can provide pain relief equivalent to that of opioids and may reduce the incidence of opioid-induced vomiting (see Chapter 41).29
Cochlear Implants
In recent years, the indications for cochlear implants have broadened and continue to evolve. With the application of universal neonatal hearing screening programs, a large pool of hearing-impaired infants has been identified. The benefits of early intervention with cochlear implants are being explored. Younger children with severe to profound hearing loss markedly improve their auditory, speech, and language skills after cochlear implants, and more of these children can be mainstreamed with their age-appropriate hearing peers when they receive an implant early in life. Experience has shown that cochlear implant surgery is safe in infants older than 6 months of age, provided that special attention is paid to the physiologic and anatomic differences present in this age-group. Surgery requires meticulous care with hemostasis, soft tissue dissection, and bone drilling because bleeding from bone can be difficult to control and can complicate the surgical outcome. Availability of skilled postoperative nursing and a pediatric intensive care unit (ICU) is also essential.30 Postoperative fitting of the externally worn speech processor is very important for successful use of the cochlear implant. However, this fitting process can be difficult, particularly in infants and young children, because of limited communication capabilities. Stapedius reflex thresholds obtained intraoperatively have been used for postoperative speech processor fitting, although the influence of anesthetics on the threshold values must be taken into account. More reliable threshold values can be obtained by adjusting the dosage of hypnotics to achieve a lighter level of hypnosis during stapedius reflex measurement.31 In most children, increasing the concentration of inhalational anesthetics increases the stapedius reflex threshold. As always, appropriate communication with the surgeon will help ensure a successful outcome.
Anesthesia for Rhinologic Procedures
Chronic sinusitis in children can be caused by antibiotic-resistant bacteria and is usually treated with broad-spectrum antibiotics. In some children with obstructive adenoid pads, adenoidectomy will improve the signs and symptoms of sinusitis. Functional endoscopic sinus surgery using sharp biting instruments and/or a microdebrider has become the primary method of surgical therapy for chronic sinusitis.32 Current techniques aim to leave the mucosa intact to prevent scarring in the frontal recess. Although sometimes controversial, there is no evidence at present that functional endoscopic sinus surgery affects facial growth in children. Of interest to the anesthesiologist is that many children who require functional endoscopic sinus surgery have coexisting medical problems, such as asthma and cystic fibrosis. These conditions must be optimized before surgery (see Chapter 11).
Because bleeding is inevitable with this surgery and can interfere with the surgical exposure, packing the nasal cavity with a vasoconstricting solution is frequently done before surgery commences. The most commonly used topical vasoconstrictors include oxymetazoline 0.025% to 0.05%, phenylephrine 0.25% to 1%, and cocaine 4% to 10%. It is important for the anesthesiologist to be aware of the type and dose of the vasoconstrictor used and that no more than the maximum effective dose is applied. Application of topical phenylephrine or other potent vasoconstrictors to mucous membranes or open surgical sites can cause severe hypertension, reflex bradycardia, and even cardiac arrest.33 Hypertension that is induced by topically applied vasoconstrictors often resolves spontaneously and may not require aggressive treatment. The use of β-adrenergic blockers or calcium-channel blockers to control blood pressure in these circumstances can depress cardiac output, leading to pulmonary edema and cardiac arrest.33 It is recommended that the initial topical dose of phenylephrine should not exceed 20 µg/kg in children.33
Corticosteroids, such as IV dexamethasone (0.25 to 0.5 mg/kg), are usually administered to reduce swelling and scarring. Frequently, the surgeon will want to leave an absorbable stenting material, such as MeroGel (Medtronic ENT, Jacksonville, Fla.), at the end of surgery. Unfortunately, this will interfere with nasal breathing and may increase the incidence of emergence agitation. An anesthetic technique that ensures adequate analgesia and rapid return of consciousness at the end of surgery is therefore desirable. One of us (RSH) has found that a combination of desflurane, fentanyl, and low-dose propofol works well in this regard. A unilateral or bilateral infraorbital nerve block can also be performed via the intraoral or extraoral route to provide analgesia (see Chapter 41).34 One further concern is the need to avoid nonsteroidal antiinflammatory drugs (NSAIDs) in children with asthma and sinusitis secondary to nasal polyps (Samter triad).35
Adenotonsillectomy
Adenotonsillectomy is one of the oldest and most commonly performed pediatric surgical procedures worldwide. More selective indications, however, have reduced the annual caseload.36,37 Chronic or recurrent tonsillitis and obstructive adenotonsillar hyperplasia are the major indications for surgical removal, although other indications do exist (Table 31-1).38,39 Surgical treatment is required when tonsillitis recurs despite adequate medical therapy, or when it is associated with peritonsillar abscess or acute airway obstruction. Halitosis, persistent pharyngitis, and cervical adenitis may accompany chronic tonsillitis. Tonsillar hyperplasia may lead to chronic airway obstruction, resulting in sleep apnea, CO2 retention, intermittent nocturnal hypoxemia, cor pulmonale, failure to thrive, swallowing disorders, and speech abnormalities (Fig. 31-2). Many of these adverse effects are reversible with surgical excision of the tonsils. Certain children with cardiac lesions may be at risk for endocarditis caused by recurrent streptococcal bacteremia secondary to infected tonsils and will require prophylactic antibiotics (see Chapter 14).
TABLE 31-1 Indications for Adenotonsillectomy
Surgical techniques for adenotonsillectomy include guillotine and snare techniques, cold and hot knife dissection, suction, radiofrequency ablation, and unipolar and bipolar electrocautery techniques. A major advantage of the electrocautery dissection technique is a reduction in the incidence of intraoperative blood loss, as well as postoperative hemorrhage; a major disadvantage is greater pain and poor oral intake postoperatively.40–44
The mortality associated with adenotonsillectomy is estimated at 1 per 16,000 to 1 per 35,000 procedures.45,46 Hospital-based reviews of anesthetic mortality continue to list adenotonsillectomy as a surgical procedure associated with perioperative cardiac arrest and death.47,48 Bleeding, burns, and airway fires account for over one third of malpractice claims associated with this procedure.49
Surgical complications after adenotonsillectomy are rare but include uvular amputation, uvular edema, velopharyngeal insufficiency, and nasopharyngeal stenosis. Atlantoaxial subluxation manifesting as neck pain and torticollis, mandibular subluxation and condylar fracture, cervical adenitis, and cervical osteomyelitis have also been reported.46,50
Throat pain, otalgia, emesis, poor oral intake, and dehydration are common morbidities. Respiratory morbidity after adenotonsillectomy in the otherwise healthy child affects less than 1%,51–53 but has assumed a greater importance since obstructive breathing has replaced infection and halitosis as the most common indication for adenotonsillectomy.
Age has a major influence on postadenotonsillectomy complications. Secondary postadenotonsillectomy hemorrhage is more common in children older than the age of 10 years.54,55 Young age is a risk factor for both poor oral intake and respiratory complications. The majority of children younger than age 3 years experience airway problems after adenotonsillectomy for obstructive breathing.56,57
Preoperative Evaluation
The general health of the child and the indications for surgery must be reviewed. URIs are frequent in these children and can interfere with the timing of adenotonsillectomy because the risk of respiratory morbidity and hemorrhage is increased.46,51,58,59 A history of bleeding tendencies requires investigation. Medications that interfere with coagulation include aspirin, NSAIDs, and valproic acid. Discontinuation of these drugs preoperatively is sometimes problematic, and preoperative consultation with neurology, cardiology, and hematology specialists may be indicated.
A careful cardiorespiratory history and physical examination is essential. Children with chronic tonsillar hypertrophy may have long-standing hypoxemia and hypercarbia, which can lead to cor pulmonale (see Fig. 31-2). The oropharynx should be evaluated and the tonsillar size classified (Fig. 31-3).60 In some centers, a complete blood cell count is required before adenotonsillectomy. There is no evidence that routinely performed preoperative coagulation studies are beneficial unless they are indicated by history.61,62 The indications for the procedure should be clearly delineated. If the indication is for obstructed breathing, then further evaluation for symptoms of possible obstructive sleep apnea (OSA) is indicated. Parents should be asked if the child snores loudly, if the snoring can be heard through a closed door, if there are gasps or pauses in respirations, if there is daytime somnolence, night terrors, nocturnal enuresis, attention deficit disorder, or poor school performance. A positive response to any of these questions is suggestive of OSA, in particular when combined with obesity (weight greater than the 95th percentile).63–67
Special Considerations for the Child with OSA
The single most important task during the preoperative evaluation of the child for adenotonsillectomy is to distinguish the child with OSA from the child with obstructive breathing, because the former is at greater risk for developing severe perioperative respiratory complications, possibly including death, after adenotonsillectomy.68–71b
OSA is the most severe form of sleep-disordered breathing. Sleep-disordered breathing ranges from normal respirations to primary snoring, upper airway resistance syndrome (UARS), obstructive hypopnea, and OSA. At the most extreme form of sleep-disordered breathing, OSA, clinical signs of partial or complete upper airway obstruction (UAO) must be present during sleep, as well as some degree of hypercarbia and/or hypoxemia.72 Although it is important to recognize the significance of OSA in children who are scheduled for adenotonsillectomy, children who do not meet the criteria for OSA but who have less severe forms of sleep-disordered breathing, such as UARS or obstructive hypopnea, may also be at increased risk for morbidity after surgery. Guidelines for the perioperative management of these children continue to be developed.71a,73,74
A high index of suspicion is required to identify the child with OSA on clinical criteria, although clinical criteria do not distinguish primary snoring from OSA in children.75 There is a greater incidence of OSA in Asian and African American populations.76,77 In addition, African American children desaturate more profoundly during sleep-related obstructive airway events than do Caucasian and Hispanic children78; the reason for this difference is unclear.
Anatomic features, including increased nasal resistance, may underlie the pathogenesis of OSA; common medical conditions and syndromes that predispose to the development of OSA are listed in Table 31-2. Infants suffering acute life-threatening events have a greater incidence of OSA in childhood and adolescence.79–81
TABLE 31-2 Medical Conditions in Children That Predispose to Development of Obstructive Sleep Apnea
The obstructive events that characterize OSA result in recurrent episodes of hypoxia, hypercarbia, and sleep disruption, a trilogy that has been linked to the development of medical sequelae that accompany severe OSA. Because adenotonsillectomy is very often the initial treatment for the majority of children, these children may present with a spectrum of disease affecting multiple organ systems. Failure to thrive is common. Cardiovascular abnormalities, including ventricular dysfunction, a depressed ventricular ejection fraction, right ventricular hypertrophy, and pulmonary hypertension, may be present.82–85 Repeat infections affecting the lower respiratory tract have been linked to chronic aspiration.86
The severity of OSA is assessed by the frequency and severity of the obstructive respiratory events during sleep; both vary with sleep stage and occur most often during rapid eye movement (REM) sleep. The frequency and severity of obstructive events worsen after midnight, a finding that may reflect the greater proportion of REM sleep in the latter part of the night and fatigue of the upper airway musculature.87–89
Apneas are classified as central, obstructive, and mixed. Central apnea occurs when there is no apparent respiratory effort. Obstructive apnea is associated with apparent, often vigorous, inspiratory efforts that are ineffective because lack of upper airway patency. A mixed obstructive apnea is diagnosed when both central and obstructive apnea occur without interruption by effective respirations. The presence of sleep-disordered breathing is documented by polysomnography and is quantitated by the frequency of obstructive events and by oxygen-desaturation indices. The polysomnogram simultaneously records the electroencephalogram, electromyogram, electrocardiogram, pulse oximetry, airflow, and thoracic and abdominal movement during sleep. A recent consensus paper on the criteria for diagnosing OSA in children has been developed.71a,84 A common definition of obstructive apnea in children is an obstructive effort that includes more than two obstructive breaths, regardless of the duration of the apnea.87 An obstructive apnea index of 1 is the cutoff for normality in children.90 Hypopnea is defined as a reduction in airflow of more than 50%.87 The apnea hypopnea index (AHI) is the summation of the number of obstructive apnea and hypopnea events and is analogous to the respiratory disturbance index (RDI). A common definition of desaturation is a 4% decrease in oxygen saturation from baseline. The saturation nadir is the minimum oxygen saturation recorded during the sleep study. A saturation nadir of 92% is the minimum normal saturation in children.90,91
The severity of OSA predicts the nature of perioperative respiratory complications (Table 31-3). An RDI of greater than 20 events per hour is associated with breath holding during induction, whereas an RDI greater than 30 is associated with laryngospasm and desaturation during emergence.92 Ten obstructive events per hour during a screening polysomnogram is the threshold for severe postoperative respiratory complications.69 An oxygen-saturation nadir less than 80% is associated with a greater incidence of respiratory morbidity after adenotonsillectomy, compared with a saturation nadir greater than 80%.68,71
TABLE 31-3 Clinical Diagnostic Criteria for Pediatric Obstructive Sleep Apnea Syndrome
1. Predisposing physical characteristics
2. History of apparent airway obstruction during sleep (two or more of the following)
3. Somnolence (one or more of the following)
Note: If signs and symptoms in at least two categories are present, there is a significant probability of moderate obstructive sleep apnea (OSA). If severe abnormalities are present, children should be treated as having severe OSA.
Modified from Table 1 in Gross JB, Bachenberg KL, Benumof JL, et al. Practice guidelines for the perioperative management of patients with obstructive sleep apnea: a report by the American Society of Anesthesiologists Task Force on Perioperative Management of patients with obstructive sleep apnea. Anesthesiology 2006;104:1081-93.
The RDI and AHI correlate inversely with the oxygen-saturation nadir,93,94 making simplified testing with continuous pulse oximetry a meaningful metric. The McGill oximetry score has been shown to correlate with the risk of respiratory complications after adenotonsillectomy in children (Fig. 31-4). Of children with a McGill oximetry score of 4, 24% experienced major postoperative respiratory complications.94
Consultations to plan the perioperative care of children with severe OSA are important. Young children with profound oxygen desaturation during sleep and CO2 retention may require admission to the pediatric ICU for optimization before and/or after adenotonsillectomy.36,94 Urgent adenotonsillectomy for severe OSA is associated with significant respiratory morbidity after surgery.70,95 On occasion, adenotonsillar hypertrophy may progress to compromise the upper airway during wakefulness. In some instances the anesthetic considerations for the obstructed and difficult airways may overlap.
Anesthetic Management and Postoperative Considerations
The anesthetic goals for adenotonsillectomy are (1) to provide a smooth, atraumatic induction; (2) to provide the surgeon with optimal operating conditions; (3) to establish IV access for volume expansion and medications as indicated; and (4) to provide rapid emergence so that the child is awake and able to protect the recently instrumented airway. The need for a premedication is determined during the preanesthetic evaluation. Children with symptoms of sleep-disordered breathing who require premedication should be closely observed, although the desaturation is transient and infrequent (1.5% of cases) after oral midazolam premedication.96 Monitoring with pulse oximetry after premedication may be indicated for select children with severe OSA and confounding variables.96 Premedication with short-acting drugs and/or those that can be antagonized is advised (see further).
The anesthetic techniques for adenotonsillectomy are varied and include the choice of an inhalational or IV technique, the choice of an ETT or LMA, and the choice of spontaneous or controlled ventilation. Of the currently available inhalational agents, sevoflurane provides a smooth induction of anesthesia, and desflurane (for those whose airway is secured with an ETT) used for maintenance provides a rapid emergence and recovery.97,98 The rapid return of airway reflexes is particularly important when the dose of opioids must be titrated after extubation.
Children who are scheduled for adenotonsillectomy have a high incidence of airway reactivity and laryngospasm. This will influence the choice of airway management. Placement of an oral RAE or standard uncuffed ETT with a leak at 20 cm H2O (the leak increases with neck extension and insertion of the mouth gag) is generally sufficient to prevent soiling of the trachea during the surgery, yet reduces the incidence of postextubation croup. Cuffed ETTs have become increasingly used in children of all age-groups.99 A cuffed tube prevents an air leak and the consequent bubbling of gases through the oropharyngeal secretions and blood that can interfere with surgery. It also minimizes pollution by anesthetic gases and decreases the risk of an airway fire when electrocautery is used.
Blood and secretions may be present in the oropharynx at the conclusion of surgery and should be carefully suctioned before emergence from anesthesia. Emptying the stomach with an orogastric tube, a maneuver frequently performed by the surgeon under direct vision after completion of surgery, does not reduce the incidence of PONV, although the study was underpowered to detect a difference.100
It is preferable to wait until the child is fully awake and able to clear blood and secretions from the oropharynx before removing the ETT. A common practice is to position the child in the lateral position (known as the “tonsil” or “recovery” position) with the head slightly down at the time of extubation to permit blood and secretions to pool in the dependent cheek and drain out of the mouth rather than accumulate at the laryngeal inlet. Intact airway and pharyngeal reflexes are of utmost importance in preventing aspiration, laryngospasm, and airway obstruction.101 The child should remain in the tonsil position postoperatively, while being carefully observed and monitored during transport to the recovery room.
The use of the LMA for adenotonsillectomy was described in 1990, but it was not until the widespread availability of a model with a flexible spiral, metallic reinforced shaft that it was widely used (E-Fig. 31-1).102,103 The wide, rigid tube of the original model did not fit under the mouth gag and was easily compressed or dislodged during full mouth opening. The newer, flexible model has a soft, reinforced shaft, which easily fits under the mouth gag without becoming dislodged or compressed. Adequate surgical access can be achieved and the airway is reasonably well protected from exposure to blood during the surgery.104,105 Early advantages cited for the LMA over the ETT include a decrease in the incidence of postoperative stridor and laryngospasm, and an increase in immediate postoperative oxygen saturation,106 although recent evidence disputes any difference in the frequency of laryngospasm.107 Insertion of the LMA, however, may be difficult in the presence of tonsillar enlargement, and careful placement to avoid kinking of the LMA is essential.108 Although it has been recommended that the LMA be used only in spontaneously breathing children and that positive-pressure ventilation be avoided, gentle assisted ventilation is both safe and effective if peak inspiratory pressure is limited to 20 cm H2O or less. If there is any leak of gases around the LMA then the potential for an airway fire must be considered and appropriate precautions taken if electrocautery is used.
Analgesic Management
Surgical technique has a major impact on the analgesic requirements after adenotonsillectomy because electrocautery techniques are generally associated with greater pain, presumably owing to increased thermal injury,40,41,109 although this is debated.110 Opioids have been the mainstay of perioperative analgesia. However, because opioids increase the incidence of emesis111 and respiratory morbidity, the use of opioid-sparing adjuncts has been advocated, including dexamethasone, acetaminophen, NSAIDs, and ketamine.
A single intraoperative dose of dexamethasone reduces postadenotonsillectomy pain and edema when electrocautery has been used. Large doses are traditionally used, especially in children with OSA. Dexamethasone (1 mg/kg) administration is associated with reduced parental- and physician-rated pain scores after adenotonsillectomy (Table 31-4).41 The minimum morphine-sparing dose for dexamethasone is reported to be 0.5 mg/kg.112 For dexamethasone doses between 0.0625 mg/kg and 1.0 mg/kg, the frequency of postoperative vomiting, pain scores, and times to first liquid and first analgesics were similar.113 A similar absence of a dose response for dexamethasone between 0.050 and 0.15 mg/kg for vomiting after tonsillectomy was reported in another study.114 Single doses of dexamethasone have not been associated with aseptic necrosis of the hip or infections, but have been responsible for several cases of acute tumor lysis syndrome, including one death.115–117 One study suggested an increased risk of bleeding after tonsillectomy in children who received dexamethasone up to 0.5 mg/kg (maximum 20 mg).118 These findings have been refuted by a recent meta-analysis and several studies.119–122a
TABLE 31-4 Effect of Single Intraoperative Dose of Dexamethasone on Postoperative Pain in Pediatric Tonsillectomy or Adenotonsillectomy: A Comparison of Randomized, Double-Blind Studies

The routine use of NSAIDs for adenotonsillectomy remains controversial because of the potential for postadenotonsillectomy hemorrhage. A meta-analysis of seven randomized controlled trials (505 children) on the effects of NSAIDs on bleeding risk after tonsillectomy reported the number needed to harm, in terms of reoperation for hemostasis, to be 29.123 NSAIDs were associated with a greater risk of both postoperative bleeding that required treatment and reoperation for hemostasis. The Cochrane Collaboration assessed the effect of NSAIDs on bleeding after pediatric tonsillectomy in 13 trials (955 children) and found no increase in bleeding that required reoperation for hemostasis.124 An audit of more than 4800 pediatric tonsillectomies in which the NSAIDs diclofenac and ibuprofen were routinely used, reported a primary hemorrhage rate of 0.9%.55 Because the effects of ketorolac on platelet function are reversible, the effect is dependent on the presence of ketorolac within the body.125 Thus, unlike the effect of aspirin, this effect is short-lived. However, we recommend administering NSAIDs only after consulting with the surgeon and, if in agreement, administering them after hemostasis is achieved.126 Acetaminophen is commonly used as a component of multimodal analgesic approach in these children.127 IV formulations of acetaminophen are now available in many countries, offering the theoretical advantage of greater predictability than the oral and rectal routes. However, recent studies suggest that the duration of analgesia after 15 mg/kg of acetaminophen given IV is less than that after 40 mg/kg given rectally.128 Furthermore, although introduced only 7 years ago, two reports of 10-fold overdoses of IV acetaminophen with near-catastrophic outcomes in infants should alert clinicians to the very serious risk of dosage errors with this medication.129
An IV infusion of dexmedetomidine 2 µg/kg over 10 minutes followed by 0.7 µg/kg/hr combined with an inhalation agent can provide satisfactory intraoperative conditions for adenotonsillectomy without adverse hemodynamic effects. In children with OSA syndrome, postoperative opioid requirements are significantly reduced and the incidence and severity of severe emergence agitation is reduced, with few children desaturating.130 After larger doses of dexmedetomidine (2 and 4 µg/kg), the opioid-free interval increases and the postoperative opioid requirements decrease. However, duration of stay in the PACU is prolonged.131
Infiltration of local anesthetics into the tonsillar fossa during tonsillectomy is sometimes reported to decrease postoperative pain, but the pain relief is transient (E-Fig. 31-2).132 In addition, life-threatening complications have been reported after local anesthetic infiltration in the tonsillar fossa, including intracranial hemorrhage, bulbar paralysis, deep cervical abscess, cervical osteomyelitis, medullopontine infarct, and cardiac arrest. The risks associated with injection of local anesthesia in the tonsillar fossa may outweigh its potential benefits, particularly in inexperienced hands.133,134
Postoperative Nausea and Vomiting
Emesis and poor oral intake are common comorbid conditions after adenotonsillectomy. Opioids increase the incidence of PONV, with two thirds of treated children experiencing PONV.37,55,135 The incidence of PONV increases with morphine dose.111,136 Propofol infusions,136a,136b ondansetron and dexamethasone are widely used to reduce the incidence of emesis after adenotonsillectomy. Postdischarge vomiting continues for days in some children. One study has shown that at-home use of oral ondansetron disintegrating tablets may prevent emesis during the first 3 days after adenotonsillectomy.137 A single intraoperative dose of dexamethasone reduces the incidence of emesis during the first 24 hours after adenotonsillectomy.138 The number of children needed to treat was only four, which means that the use of dexamethasone in four children undergoing adenotonsillectomy results in one less child experiencing PONV. In addition, children who received dexamethasone were more likely than those receiving placebo to advance to a soft diet on postoperative day 1, with a number needed to treat of five. Given the antiemetic and possible morphine-sparing advantages of a single dose of dexamethasone, and its low cost and safety profile, the evidence suggests that routine use of dexamethasone reduces morbidity after adenotonsillectomy in children.41,138 Although the literature supports the effectiveness of a single dose of dexamethasone, the smallest effective dose remains somewhat unclear. One study suggested an IV dose of 0.15 mg/kg,139 whereas another reported no difference in postoperative vomiting, pain scores, time to first liquid, and time to first analgesics between doses of 0.0625 and 1.0 mg/kg (Fig. 31-5).113 Acupuncture, acupressure, as well as therapeutic suggestion, have also been used with variable results.140–142
Special Considerations for Children with OSA
Children with OSA who require premedication should be closely observed, because transient oxygen desaturation has been reported in 1.5% of children with OSA who received 0.5 mg/kg oral midazolam.96
Induction of Anesthesia
Compared with children undergoing adenotonsillectomy for chronic tonsillitis, those whose indication was OSA experienced more respiratory complications during induction of anesthesia.92 The vulnerability of the upper airway musculature described for halothane143 has subsequently been reported for most anesthetic agents, resulting in a graded reduction in airway caliber with increasing anesthetic concentration.144–148 Airway obstruction occurs in the upper two thirds of the pharyngeal airway, and the smallest pharyngeal dimension is in the area of overlap between the adenoids and tonsils.147 During induction of anesthesia, early pharyngeal airway obstruction may require a jaw thrust maneuver, insertion of an oral or nasopharyngeal airway, and the application of continuous positive airway pressure (CPAP). Propofol-associated loss in airway caliber is reversed with the application of CPAP.148 CPAP acts as a pneumatic splint to increase the caliber of the pharyngeal airway (Fig. 31-6).149 Of equal importance, CPAP increases longitudinal tension on the pharyngeal airway, thereby decreasing the collapsibility of the upper airway (see Fig. 12-10), and increases lung volumes.150,151 Small increments in CPAP between 5 and 10 cm H2O increase the dimension of the pharyngeal airway dramatically (Fig. 31-7).152,153 The closing pressure of the pharynx increases with OSA severity, such that greater levels of CPAP are required in children with severe OSA compared with those with mild OSA. It is prudent to consider securing IV access before induction of anesthesia in children with severe OSA, to expedite administration of muscle relaxants or IV agents should pharyngeal obstruction or laryngospasm occur during induction. The small oropharynx and adenotonsillar hypertrophy associated with severe OSA may increase the difficulty in properly inserting an LMA.
Analgesic Management in Children with OSA
Severe OSA is characterized by recurrent episodes of transient hypoxia and hypercarbia during sleep. In animal models, exposure to intermittent hypoxia during development affects the opioid system, increasing the density of µ-opioid receptors in the respiratory-related areas of the brainstem. The cellular mechanism whereby this increased density is achieved has yet to be elucidated, but it may represent an adaptive response to the effects of recurrent intermittent hypoxia that allows µ-receptor–mediated opioid respiratory effects to predominate.154–157
For children with severe OSA, the severity of the nocturnal oxygen desaturation correlates with the sensitivity to exogenously administered opioids (Fig. 31-8).158–160 The morphine dose required to achieve a uniform analgesic endpoint in children with OSA who exhibited a low preoperative oxygen-saturation nadir during sleep (less than 85%) (Fig. 31-9) was less than in those whose preoperative saturation nadir was greater.159 Young age was also associated with an increased sensitivity to opioids. An unforeseen risk of perioperative opioid use in children with severe OSA is that smaller-than-expected doses of opioids may produce exaggerated respiratory depression. Of children with severe OSA who were anesthetized with halothane, 46% experienced apnea after a uniform dose of fentanyl, compared with 4% of controls.161 This increased sensitivity to the respiratory depressant effects of fentanyl in children with OSA is supported by the exaggerated respiratory depression to subsequent administration of a uniform dose of fentanyl in rat pups exposed to intermittent hypoxia.162 Hence, allowing spontaneous respirations during maintenance of anesthesia enables an assessment of the response to small challenges of opioid analgesics. In this manner, the anesthesiologist can assess the sensitivity of the child with OSA to opioids. Controlling respiration precludes such an evaluation. Sleep fragmentation blunts the arousal response to acute airway occlusion during sleep.163,164 In addition, exposure to intermittent hypoxia during development is associated with an increase in the arousal latency to hypoxia.165–167 Morphine acting at the level of the basal forebrain blunts arousal.168 If the increased sensitivity to both the analgesic and respiratory effects of exogenously administered opioids reported in children with OSA extends to arousal mechanisms, the use of opioids in children with severe OSA may further impair arousal mechanisms. Guidelines for the perioperative management of OSA assign a greater risk score if opioids are used for postoperative analgesic regimens in children with OSA.91 Although these guidelines suggest that the use of low-potency oral opioid analgesia carries a reduced perioperative risk, the use of codeine, a “low-risk” oral opioid commonly used in the ambulatory setting, may also be problematic in children with OSA. Codeine is metabolized by the cytochrome P450 debrisoquine 4-hydroxylase (CYP2D6) to its active analgesic metabolites. The CYP2D6 gene displays polymorphism, including gene duplication (ultra-rapid metabolizers) and inactive genes. Gene duplication may lead to ultra-rapid metabolism, which for prodrugs, such as codeine, might yield a 50% greater fraction of morphine and its glucuronides compared with extensive metabolizers.169 Respiratory arrest after codeine has been reported in both adults and children who demonstrate ultra-rapid metabolism of codeine.170–171a Whereas the ultra-rapid metabolizing genotype is present in 3% of Caucasians, it is present in 10% to 30% of Arabian and Northeast African populations. In contrast, almost 10% of children lack CYP2D6, rendering codeine an ineffective analgesic. Given the broad variability in codeine metabolism and our lack of knowledge of which polymorphism is carried by each child, the use of codeine and the dose prescribed for children with OSA must be very carefully considered or an alternate opioid selected.
Neural Blockade
Blockade of neural input to the upper airway dilator musculature in children with OSA is also problematic. Serious life-threatening complications, including severe UAO and pulmonary edema, have been reported after local anesthetics have been infiltrated in the tonsillar fossa to prevent pain after adenotonsillectomy. The pharynx in children with OSA is not only smaller in size,147,172 but also more collapsible, even during wakefulness, compared with those children who do not have OSA.173–175 Topical anesthesia applied to the mucosa of the pharynx of children with OSA reduces the caliber of the pharynx compared with control subjects.176
Extubation Strategy and Management of the Postoperative Period in Children with OSA
Extubation of the trachea is usually performed when the child is fully awake. Techniques that involve minimal stimulation of the airway have been suggested.101 Although a minority of children receive muscle relaxants for adenotonsillectomy, residual neuromuscular blockade in the recovery room will selectively depress the function of the upper airway dilators relative to the diaphragm, promoting collapse of the pharyngeal airway.177 Full antagonism of neuromuscular blockade is strongly recommended before extubating the tracheas of children with OSA.91 Antagonism of neuromuscular blockade with atropine and neostigmine after tonsillectomy has been associated with less PONV than antagonism with glycopyrrolate and neostigmine.178
Several other factors may increase the risk of respiratory difficulties after adenotonsillectomy in children with OSA. Otherwise healthy children with severe OSA, whose adenotonsillectomy is performed in the morning, are less likely than those whose surgery is performed in the afternoon to desaturate when managed in a PACU setting.179 In addition, meticulous attention to the position of the head and neck is required during recovery from anesthesia, because hypercarbia and a loss of lung volume (functional lung capacity) both promote collapse of the pharyngeal airway.151,180,181 Extension of the cervical spine, the sniffing position, the lateral recovery position, and mouth opening with anterior advancement of the mandible all increase the dimension of the pharynx182–186 and reduce the risk of UAO.
Two drugs, atropine and naloxone, have the potential to augment the function of the upper airway. Atropine administered after induction of anesthesia decreased the risk of postadenotonsillectomy respiratory complications.70 Of possible relevance is the report that muscarinic blockade of the hypoglossal nucleus in the rat model enhances activity of the genioglossus muscle.181 Agonists of opioid µ-receptors have been shown to depresses activity in the pharyngeal dilator muscles, including the genioglossus muscle.155,187–189 Given the increased sensitivity to both analgesic and respiratory effects of exogenously administered opioids in children with severe OSA, a similar sensitivity may also apply to the respiratory-related activity of the pharyngeal musculature. Small doses of naloxone may alleviate UAO after adenotonsillectomy if exogenous opioids have been administered.
The severity of OSA is a predictor of the outcome after adenotonsillectomy.190 A preoperative RDI above 19 may predict an RDI in excess of 5 in long-term follow-up.75,93 Children with OSA continue to demonstrate obstructive apnea and desaturation during sleep on the first night after adenotonsillectomy, with the frequency of the obstructive events and the severity of desaturation usually greater in those children with severe OSA (Fig. 31-10).191,192 Thus despite removal of the hypertrophied tonsils and adenoids, children with OSA continue to experience symptoms on the first postoperative night. This underscores the need to admit these children to a hospital for continuous overnight monitoring postoperatively, rather than discharge them home. Long-term follow-up studies more than 6 months after tonsillectomy in children with OSA show that symptoms completely resolve in those with mild OSA (AHI less than 10) but are persistent in 35% of those with severe OSA (AHI greater than 20).190 Furthermore, recent epidemiologic evidence suggests that residual sleep-disordered breathing is more likely to be present after adenotonsillectomy in older children (more than 7 years of age) and obese children. It has also been suggested that obese children with large tonsils and OSA also show evidence of systemic inflammatory disease that persists after the tonsillectomy.190,193
Measures to support airway patency in the postoperative period have included insertion of nasal airways, administration of noninvasive ventilatory support (e.g., CPAP), reintubation, ventilation, and the administration of bronchodilators, racemic epinephrine, and heliox. Bilevel positive airway pressure and/or CPAP may be useful in children with preexisting neurologic disorders.194 However, nasal secretions may be copious after adenotonsillectomy, limiting the efficacy of noninvasive ventilatory support. Children with complex medical diseases, who are critically dependent on the function of upper airway musculature, may benefit from delayed extubation. Acute relief of chronic UAO favors the exudation of intravascular fluid into the pulmonary interstitium and noncardiogenic pulmonary edema, which may present preoperatively, intraoperatively, and postoperatively. Supportive measures include the administration of oxygen, endotracheal intubation, mechanical ventilation with positive end-expiratory pressure, and administration of furosemide.195–197
Discharge Policy for Ambulatory Adenotonsillectomy
Children younger than 3 years of age and those with complex medical disorders are not candidates for adenotonsillectomy as outpatients.38,198 Although children undergoing adenotonsillectomy for obstructive breathing without apnea may undergo ambulatory surgery, those with OSA should not. A diagnosis of OSA increases the likelihood of respiratory complications after adenotonsillectomy from 1% in otherwise healthy children to 20% in those with OSA.37
The majority of children who are scheduled for adenotonsillectomy have symptoms of obstructive breathing,42,199,200 yet only 55% with clinical criteria suggestive of OSA subsequently meet sleep laboratory criteria for OSA.201 Sleep screening of children undergoing routine adenotonsillectomy for chronic tonsillitis revealed unexpectedly that 20% had severe obstructive episodes associated with desaturation.202 Because only a minority of children undergoing adenotonsillectomy undergo diagnostic testing for sleep-disordered breathing, the recently published guidelines on management of OSA have empowered clinical diagnostic criteria, such that a child with severe symptoms must be assumed to have moderate to severe OSA until proven otherwise by sleep laboratory testing (see Fig. 31-4). Ambulatory programs may now find it cost effective to screen children with a positive clinical history.
In otherwise healthy children, conversion from ambulatory to inpatient status was most frequently prompted by respiratory events in children whose indication for surgery was obstructive breathing.42 A systematic reduction in postoperative morphine use was associated with a reduced rate of hospital admission from 8% to 2.4%.55 Same-day discharge, which abbreviates the postoperative stay in hospital and the minimum period of observation before discharge from hospital, has been the subject of much debate. Because the onset of respiratory complications in these children may be delayed,69,179,191 a 6- to 8-hour period of observation for respiratory complications after adenotonsillectomy for OSA has been suggested. However, this extended period of observation does not preclude the delayed onset of sleep-related respiratory compromise after adenotonsillectomy. Table 31-5 presents common admission criteria for children undergoing elective tonsillectomy.
TABLE 31-5 Criteria for Overnight Admission after Tonsillectomy and Adenoidectomy
From Zalzal G: Personal communications, survey of major pediatric hospitals, 2006.
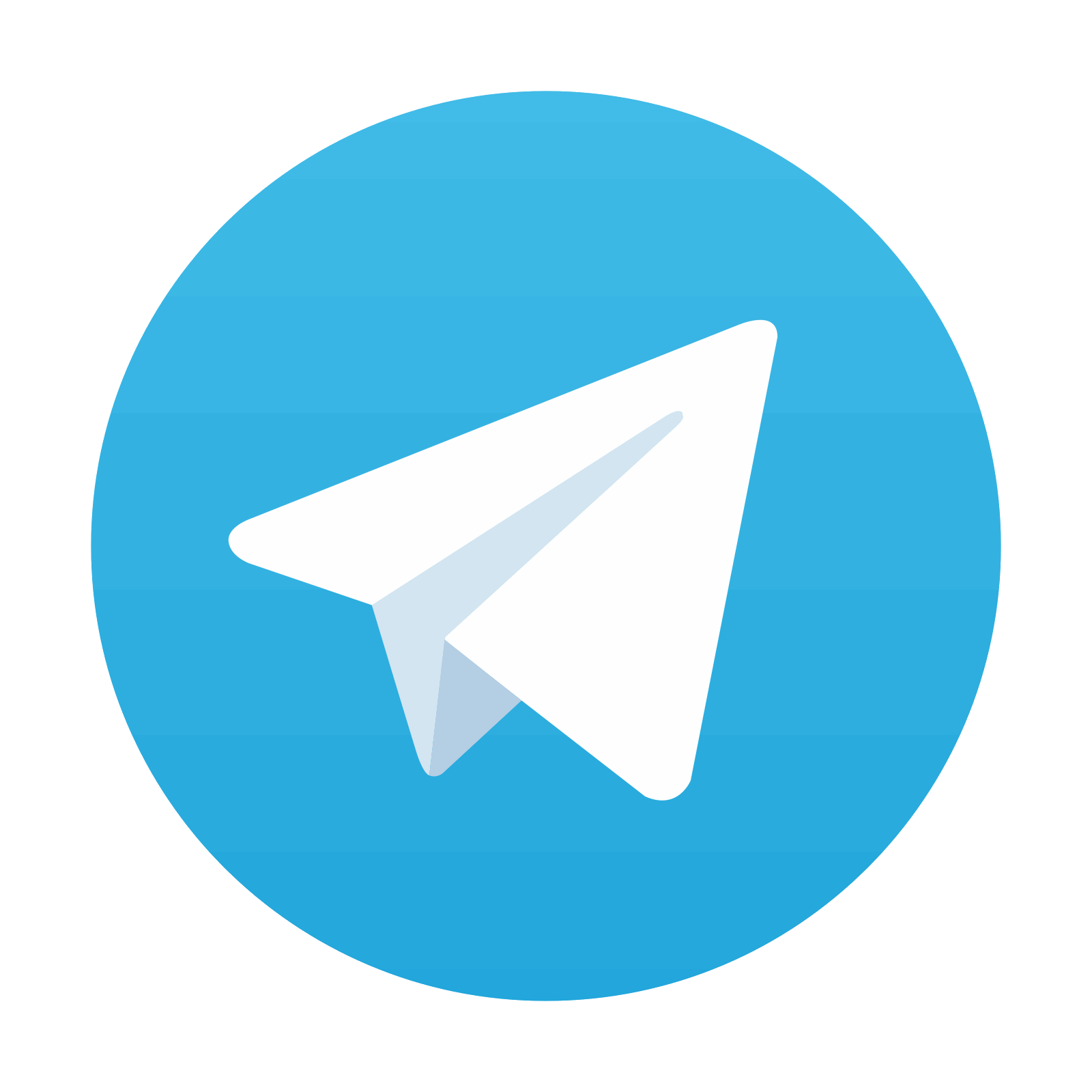
Stay updated, free articles. Join our Telegram channel

Full access? Get Clinical Tree
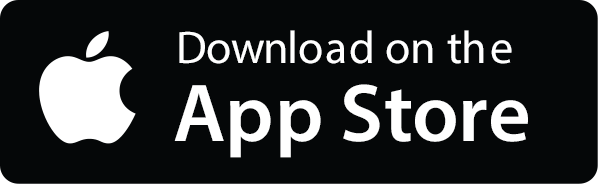
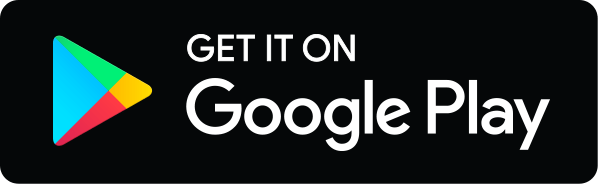